21 Thoracic Aorta
Thoracic aortic diseases typically require surgical intervention (Table 21-1). Acute aortic dissections, rupturing aortic aneurysms, and traumatic aortic injuries are surgical emergencies. Subacute aortic dissection and expanding aortic aneurysms require urgent surgical intervention. Stable thoracic or thoracoabdominal aortic aneurysms (TAAAs), aortic coarctation, or atheromatous disease causing embolization may be addressed surgically on an elective basis. The volume of thoracic aortic procedures has grown steadily because of factors such as increased public awareness, an aging population, earlier diagnosis, multiple advances in imaging, and advances in surgical techniques including endovascular stenting. Medical centers have emerged that specialize in thoracic aortic diseases, resulting in improved management and survival. This progress has created a set of patients who later require reoperation for long-term complications such as valve or graft failure, pseudoaneurysm at anastomotic sites, endocarditis, and/or progression of the original disease process into residual native aorta.
TABLE 21-1 Thoracic Aortic Diseases Amenable to Surgical Treatment
Aneurysm |
Congenital or developmental |
Marfan syndrome, Ehlers–Danlos syndrome |
Degenerative |
Cystic medial degeneration |
Annuloaortic ectasia |
Atherosclerotic |
Traumatic |
Blunt and penetrating trauma |
Inflammatory |
Takayasu’s arteritis, Behçet syndrome, Kawasaki disease |
Microvascular diseases (polyarteritis) |
Infectious (mycotic) |
Bacterial, fungal, spirochetal, viral |
Mechanical |
Poststenotic, associated with an arteriovenous fistula |
Anastomotic (postarteriotomy) |
Pseudoaneurysm |
Aortic dissection |
Stanford type A |
Stanford type B |
Intramural hematoma |
Penetrating atherosclerotic ulcer |
Atherosclerotic disease |
Traumatic aortic injury |
Aortic coarctation |
Data from Kouchoukos NT, Dougenis D: Surgery of the aorta. N Engl J Med 336:1876, 1997.
The anesthetic management of thoracic aortic diseases has unique considerations including the temporary interruption of blood flow, often resulting in ischemia of major organ systems. Critical components of anesthetic management include the maintenance of organ perfusion, the protection of vital organs during ischemia, and the monitoring and management of end-organ ischemia. As a result, the vigilant and skillful anesthesiologist contributes importantly to the overall success of these operations. The procedures performed by the thoracic aortic team for organ protection, such as partial left-heart bypass (PLHB) for distal aortic perfusion, cardiopulmonary bypass (CPB) with deep hypothermic circulatory arrest (DHCA), selective cerebral perfusion, and lumbar cerebrospinal fluid (CSF) drainage, are practiced routinely in no other area of medicine. The recently published multisociety guidelines represent a contemporary evidence-based, consensus-driven approach to thoracic aortic diseases. Their recommendations are referred to throughout this chapter, based on the well-known classification of recommendations and levels of evidence (Tables 21-2 and 21-3) by the American College of Cardiology (ACC) and American Heart Association (AHA).1
TABLE 21-2 Classification Scheme for Clinical Recommendations
Clinical Recommendations | Definition of Recommendation Class |
---|---|
Class I | The procedure/treatment should be performed (benefit far outweighs the risk). |
Class IIa | It is reasonable to perform the procedure/treatment (benefit still clearly outweighs risk). |
Class IIb | It is not unreasonable to perform the procedure/treatment (benefit probably outweighs the risk). |
Class III | The procedure/treatment should not be performed as it is not helpful and may be harmful (risk may outweigh benefit). |
Data from Hiratzka LF, Bakris GL, Beckman JA, et al: 2010 ACCF/AHA/AATS/ACR/ASA/SCA/SCAI/SIR/STS/SVM guidelines for the diagnosis and management of patients with thoracic aortic disease: Executive summary. A report of the American College of Cardiology Foundation, American Heart Association Task Force on Practice Guidelines, American Association for Thoracic Surgery, American College of Radiology, American Stroke Association, Society of Cardiovascular Anesthesiologists, Society for Cardiovascular Angiography and Interventions, and Society for Vascular Medicine. Circulation 121:e266–e369, 2010.
TABLE 21-3 Classification Scheme for Supporting Evidence for Clinical Recommendations
Supporting Evidence | Estimate of Certainty |
---|---|
Level A | Data derived from multiple randomized clinical trials (RCT) or meta-analysis. |
Level B | Data derived from a single RCT or nonrandomized studies. |
Level C | Only consensus opinions of experts, case studies, or standard of care. |
Data from Hiratzka LF, Bakris GL, Beckman JA, et al: 2010 ACCF/AHA/AATS/ACR/ASA/SCA/SCAI/SIR/STS/SVM guidelines for the diagnosis and management of patients with thoracic aortic disease: Executive summary. A report of the American College of Cardiology Foundation, American Heart Association Task Force on Practice Guidelines, American Association for Thoracic Surgery, American College of Radiology, American Stroke Association, Society of Cardiovascular Anesthesiologists, Society for Cardiovascular Angiography and Interventions, and Society for Vascular Medicine. Circulation 121:e266–e369, 2010.
Anatomy of the aorta
During fetal development, the ductus arteriosus diverts blood from the pulmonary artery into the distal aortic arch. After birth, lung expansion and constriction of the ductus arteriosus because of increased blood oxygen content drives the blood from the pulmonary artery into the pulmonary circulation. Typically, the ductus arteriosus is functionally closed by 48 hours and is permanently closed by 3 weeks after birth.2 It subsequently fibroses to become the “ligamentum arteriosum.” Occasionally, this process fails and the ductus arteriosus remains patent. Furthermore, occasionally a ductal diverticulum may persist and be confused in later life with an aortic injury during aortic imaging. The pathogenesis of aortic coarctation may be related to residual ductal tissue that narrows the aorta as it constricts.
The coronary arteries are the first branches of the aorta. The aortic arch subsequently gives origin to the innominate, left carotid, and left subclavian arteries that supply the head, neck, and arms. The innominate artery (brachiocephalic trunk) is the first branch of the aortic arch, followed by the left common carotid artery, and, finally, the left subclavian artery. There are multiple aortic arch anatomic variations, including vascular rings, right-sided aortic arch, and branching anomalies. A right-sided aortic arch is found in about 0.1% of the population. A relatively common aortic arch branch anomaly with a 4% prevalence rate is an isolated left vertebral artery, so named because it arises directly from the aortic arch.3
The aortic arch also modulates blood pressure via baroreceptors within its outer wall. The aortic bodies are located inferior to the aortic arch. The aortic baroreceptors respond to a greater threshold pressure and thus are less sensitive when compared with the carotid sinus receptors. These receptors send impulses to the brainstem that interact with the medullary cardiovascular center for modulation of autonomic nervous system activity.4
General considerations for the perioperative care of aortic surgical patients
Patients undergoing thoracic aortic surgery share common considerations for the safe conduct of anesthesia and perioperative care that are addressed in this section (Table 21-4). The unique considerations and care that apply to specific diseases and procedures are addressed in subsequent sections devoted to their management.
TABLE 21-4 Anesthetic Considerations for the Care of Thoracic Aortic Surgical Patients
Preanesthetic Assessment |
Urgency of the operation (emergent, urgent, or elective) |
Pathology and anatomic extent of the disease |
Median sternotomy vs. thoracotomy vs. endovascular approach |
Mediastinal mass effect |
Airway compression or deviation |
Preexisting or Associated Medical Conditions |
Aortic valve disease |
Cardiac tamponade |
Coronary artery stenosis |
Cardiomyopathy |
Cerebrovascular disease |
Pulmonary disease |
Renal insufficiency |
Esophageal disease (contraindications to TEE) |
Coagulopathy |
Prior aortic operations |
Preoperative Medications |
Warfarin (Coumadin) |
Antiplatelet therapy |
Antihypertensive therapy |
Anesthetic Management |
Hemodynamic monitoring |
Proximal aortic pressure |
Distal aortic pressure |
Central venous pressure |
Pulmonary artery pressure and cardiac output |
TEE |
Neurophysiologic monitoring |
Electroencephalography |
Somatosensory-evoked potentials |
Motor-evoked potentials |
Jugular venous oxygen saturation |
Lumbar cerebrospinal fluid pressure |
Body temperature |
Single-lung ventilation for thoracotomy |
Double-lumen endobronchial tube |
Endobronchial blocker |
Potential for bleeding |
Large-bore intravenous access |
Blood product availability |
Antifibrinolytic therapy |
Antibiotic prophylaxis |
Postoperative Care Considerations and Complications |
Hypothermia |
Hypotension |
Hypertension |
Bleeding |
Spinal cord ischemia |
Stroke |
Renal insufficiency |
Respiratory insufficiency |
Phrenic nerve injury |
Diaphragmatic dysfunction |
Recurrent laryngeal nerve injury |
Pain management |

Preanesthetic Assessment
The second consideration is to determine the aortic diagnosis because its extent and physiologic consequences dictate both anesthetic management and surgical approach. Aortic diseases proximal to the left carotid artery typically are approached via a median sternotomy, whereas aortic diseases distal to this point usually are approached via a left thoracotomy or thoracoabdominal incision. Although an aortic diagnosis often is established in advance, at times a definitive diagnosis must be verified after OR admission by direct review of diagnostic studies or by subsequent TEE. In every case, a review of the operative plan with the surgical team facilitates thorough anesthetic preparation. Direct review of adequate aortic diagnostic imaging studies not only verifies the operative diagnosis but also determines the surgical possibilities (ACC/AHA Class I recommendation; level of evidence C).1 The anatomic details of an aortic disease permit the anesthesiologist to anticipate potential perioperative difficulties, including likely postoperative complications.
The systematic assessment of each organ system in the aortic surgical patient should focus on how it will affect the conduct of anesthesia and surgery. The baseline functional reserve of each organ system determines the likely perioperative complications and allows ranking of organ-protective strategies. It is reasonable to obtain further tests to quantitate the functional reserve of affected organ systems, for example, neurocognitive testing, brain imaging, noninvasive carotid artery imaging, pulmonary function testing, echocardiography, and cardiac catheterization (ACC/AHA Class IIa recommendation; level of evidence C).1 For example, significant cerebrovascular disease affects blood pressure management to ensure adequate cerebral perfusion. Significant cardiac compromise typically increases the risks for heart failure, myocardial ischemia, and arrhythmias. Significant lung disease often is predictive for postoperative respiratory failure, pneumonia, or both. Significant renal insufficiency affects fluid management, triggers the avoidance of nephrotoxic drugs, and customizes the dosing of renally cleared drugs. Hepatic disease and hematologic dysfunction are risk factors for perioperative bleeding and transfusion. Severe aortic atheroma is a major risk factor for atheroembolism and consequent stroke and limb ischemia.
Because myocardial ischemia is an important predictor of perioperative outcome, it has featured prominently in the guidelines for thoracic aortic diseases. Patients with evidence of myocardial ischemia should undergo further evaluation to determine the extent and severity of coronary artery disease (CAD; ACC/AHA Class I recommendation; level of evidence C).1 If significant CAD is responsible for an acute coronary syndrome, then coronary revascularization is indicated before or concomitant with the thoracic aortic procedure (ACC/AHA Class I recommendation; level of evidence C).1 Concomitant coronary artery bypass grafting (CABG) is reasonable in patients who have not only stable but significant CAD, but who are also scheduled to undergo surgery for diseases of the ascending aorta or aortic arch, or both (ACC/AHA Class IIa recommendation; level of evidence C).1 In contrast, the benefit of coronary revascularization is less clear in patients who have stable but significant CAD and who are scheduled to undergo surgical intervention for descending thoracic aortic disease (ACC/AHA Class IIb recommendation; level of evidence C).1

Preoperative Medications
Preoperative medications typically provide detailed information about concomitant medical conditions. As a general rule, all cardiac, pulmonary, and antiseizure medications should be continued up until the morning of surgery. Angiotensin-converting enzyme inhibitors and angiotensin-receptor blockers should be discontinued the day before surgery to minimize the risk for perioperative vasoplegia and adverse outcomes.5,6 All oral hypoglycemic agents should be discontinued the night before surgery to avoid hypoglycemia. If possible, metformin should be discontinued the day before surgery to minimize the risks for severe lactic acidosis associated with exposure to iodinated contrast agents or perioperative hypovolemia. If a patient is receiving insulin, up to 50% of the typical morning dose should be given the day of surgery with subsequent close glucose monitoring. Warfarin (Coumadin) should be discontinued for approximately 5 days before surgery for full recovery of coagulation function as verified by a normalized international normalized ratio.7 If this is not possible, then the patient should be admitted for heparinization until shortly before surgery. Patients chronically exposed to low-molecular-weight heparin, aspirin, adenosine diphosphate platelet-receptor inhibitors (clopidogrel and prasugrel), and platelet glycoprotein IIb/IIIa inhibitors (abciximab, eptifibatide, tirofiban) are typically at increased risk for perioperative bleeding. Optimally, aspirin and clopidogrel should be discontinued at least 5 to 7 days before surgery to allow adequate recovery of platelet function for perioperative hemostasis.7

Anesthetic Management
Overall, the anesthetic plan including techniques, drugs, and monitoring should be individualized to enhance the conduct of the procedure including perfusion technique, hemodynamic monitoring, and preservation of organ function (ACC/AHA Class I recommendation; level of evidence C).1 Because thoracic aortic procedures may result in massive bleeding and cardiovascular collapse, it is essential to have immediate availability of packed red blood cells, large-bore vascular access, invasive blood pressure monitoring, and central venous access. Pulmonary artery catheterization assists in the management of cardiac dysfunction associated with CPB, DHCA, and PLHB. Intraoperative TEE is indicated in thoracic aortic procedures, including endovascular interventions, in which it assists in hemodynamic monitoring, procedural guidance, and endoleak detection (ACC/AHA Class IIa recommendation; level of evidence B).1
Large-bore peripheral intravenous cannulation (e.g., two 16-gauge catheters) secures vascular access for rapid intravascular volume expansion. Rapid transfusion is desirable via an intravenous set with a fluid warming device. Alternatively, large-bore central venous cannulation can be utilized for volume expansion. If a pulmonary artery catheter (PAC) is required, a second introducer sheath dedicated to volume expansion also can be placed in the same central vein. Central venous cannulation with ultrasound guidance often increases speed and safety, especially in emergencies.8 Both a urinary and a nasopharyngeal temperature probe are required for monitoring the absolute temperature of the peripheral and core, as well as the rates of change during deliberate hypothermia and subsequent rewarming. The rectum is an alternative site for monitoring peripheral temperature, and the PAC can provide core temperature monitoring.
General anesthetic maintenance is typically with a balanced technique, and neuromuscular blockade is achieved by titration of a nondepolarizing muscle relaxant. Anesthetics can be reduced during moderate hypothermia and then discontinued during deep hypothermia. With concomitant electroencephalographic (EEG) and/or somatosensory-evoked potential (SSEP) monitoring, anesthetic signal interference is minimized with the avoidance of barbiturates, bolus propofol, and doses of inhaled anesthetic greater than 0.5 minimum alveolar concentration. Propofol infusion, narcotics, and neuromuscular blocking drugs do not interfere with SSEP monitoring. With intraoperative motor-evoked potential (MEP) monitoring, high-quality signals are obtained when the anesthetic technique comprises total intravenous anesthesia with propofol and a narcotic such as remifentanil without neuromuscular blockade. Neuromonitoring (EEG, SSEP, MEP) in thoracic aortic procedures is not only compatible with contemporary anesthetic techniques but is also reasonable when the resulting data will guide perioperative management (ACC/AHA Class IIa recommendation; level of evidence B).1 The decision to utilize this monitoring modality should be based on procedural urgency, institutional resources, patient needs, and planned operative technique (ACC/AHA Class IIa recommendation; level of evidence B).1
In most cases, the duration of general anesthesia continues for several hours after admission to the intensive care unit (ICU) to permit a controlled anesthetic emergence. If epidural analgesia is used intraoperatively, a dilute solution of local anesthetic and narcotic is preferred to minimize postoperative hypotension from a concomitant sympathectomy and to minimize motor blockade to permit serial neurologic assessment of lower extremity function. Neuraxial anesthetic techniques are not recommended in patients at risk for neuraxial hematoma in the setting of concomitant thienopyridine antiplatelet therapy, low-molecular-weight heparins, and clinically significant anticoagulation (ACC/AHA Class III recommendation; level of evidence C).1,7
The potential for significant bleeding and rapid transfusion is always relevant in thoracic aortic procedures. Consequently, it is prudent to have fresh frozen plasma and platelets available for ongoing replacement during massive red blood cell transfusion. The time delay associated with standard laboratory testing severely limits the intraoperative relevance of these data to guide transfusion. Strategies to decrease bleeding and transfusion in these procedures include timely preoperative cessation of anticoagulants and platelet blockers, antifibrinolytic therapy, intraoperative cell salvage, biologic glue, activated factor VII, and avoidance of perioperative hypertension. It is reasonable to have an institutional algorithmic approach to the management of bleeding and transfusion for thoracic aortic surgery (ACC/AHA Class IIa recommendation; level of evidence C).1 This algorithm will depend significantly on institutional variations in point-of-care coagulation testing, blood component availability, and access to recombinant factor VII (ACC/AHA Class IIa recommendation; level of evidence C).1
The antifibrinolytic lysine analogs, ε-aminocaproic acid or tranexamic acid, are commonly utilized in thoracic aortic surgery with and without DHCA. The concerns with aprotinin are now historical because it was widely withdrawn from clinical practice after a large randomized trial demonstrated its significant mortality risk in the setting of high-risk cardiac surgery, including thoracic aortic surgery with DHCA.9,10 Even more recently, high-dose tranexamic acid has been correlated with a significantly increased risk for seizures after cardiac surgery.11,12 Based on the lessons from aprotinin, further adequately powered trials should examine the safety of lysine analogs in thoracic aortic surgery.9–12 Recombinant activated factor VII is a synthetic agent that accelerates hemostasis by binding with tissue factor at the site of tissue injury. Although this agent has demonstrated efficacy for hemostatic rescue in massive bleeding during thoracic aortic surgery, recent meta-analysis suggest further study for adequate delineation of its perioperative safety.13,14

Postoperative Care
After completion of the operation, the patient should be transported directly from the OR to the ICU. The continuation of care from the OR to the ICU should be seamless and protocol based.15 In the absence of complications, early anesthetic emergence is preferable for early assessment of neurologic function. If delayed anesthetic emergence is indicated, then sedation and analgesia can be provided. Common early complications include hypothermia, coagulopathy, delirium, stroke, hemodynamic lability, respiratory failure, metabolic disturbances, and renal failure. Frequent clinical and laboratory assessment are essential to manage this dynamic postoperative recovery, including the safe conduct of tracheal extubation. The management of blood glucose levels has been standardized with a recent guideline from the Society of Thoracic Surgeons (STS).16 The chest roentgenogram allows confirmation of endotracheal tube and intravascular catheter position, as well as the diagnosis of acute intrathoracic pathologies. Antibiotic prophylaxis is continued for 48 hours after surgery to minimize surgical infection risk.
Thoracic aortic aneurysm
A thoracic aortic aneurysm is a permanent localized thoracic aortic dilatation that has at least a 50% diameter increase and three aortic wall layers.1 Localized dilatation of the thoracic aorta less than 150% of normal is termed ectasis. Annuloaortic ectasia is defined as isolated dilatation of the ascending aorta, aortic root, and aortic valve annulus. Pseudoaneurysm or a false aneurysm is a localized dilation of the aorta that does not contain all three layers of the vessel wall and instead consists of connective tissue and clot. Pseudoaneurysms are caused by a contained rupture of the aorta or arise from intimal disruptions, penetrating atheromas, or partial dehiscence of the suture line at the site of a previous aortic prosthetic vascular graft.
Thoracic aortic aneurysms are common and are the 15th most common cause of death in people older than 65.16 This disease process is virulent (Box 21-1) but indolent because it typically grows slowly at an approximate rate of 0.1 cm/yr.16 The most common reason for more rapid degeneration is acute aortic dissection. Besides acquired risk factors such as hypertension, hypercholesterolemia, and smoking, current evidence points to the strong influence of genetic inheritance.17,18 Genetic analysis suggests that thoracic aortic aneurysms divide into two groups at the level of the ligamentum arteriosum. Above the ligamentum arteriosum, the disease is not related to typical arterial risk factors and has a smooth, noncalcified wall accompanied by no debris or clot. Below the ligamentum arteriosum, the disease process primarily is atherosclerotic, with an irregular calcified wall accompanied by copious debris and clot. This freedom from atheromatous disease in patients with thoracic aortic aneurysms of the ascending aorta has been called a “silver lining.”17 Inflammatory causes for thoracic aortic aneurysm include syphilis, mycotic aneurysm from endocarditis, giant-cell arteritis, and Takayasu arteritis.1
The aneurysm’s location and extent determine the operative strategy and related perioperative complications. Aneurysms of the aortic root and/or ascending aorta commonly are associated with a bicuspid aortic valve.19 Dilation of the aortic valve annulus, aortic root, and ascending aorta pulls the aortic leaflets apart and causes central aortic regurgitation (AR).18 Aneurysms involving the aortic arch require temporary interruption of cerebral blood flow to accomplish the operative repair. Endovascular stent repair is an established therapy for aneurysms isolated to the descending thoracic aorta.1,20 Repair of descending thoracic aortic aneurysms requires the sacrifice of multiple segmental intercostal artery branches that compromise spinal cord perfusion and results in a significant risk for postoperative paraplegia from spinal cord ischemia.21
Thoracic aortic aneurysms mostly are asymptomatic and frequently are discovered incidentally.1,17 Common symptoms of thoracic aortic aneurysm include chest and back pain caused by aneurysmal dissection, rupture, or bony erosion. The intrathoracic “mass effect” from a large thoracic aortic aneurysm can compress local structures to cause hoarseness (recurrent laryngeal nerve), dyspnea (trachea, mainstem bronchus, pulmonary artery), central venous hypertension (superior vena cava syndrome), and/or dysphagia (esophagus). Rupture of thoracic aortic aneurysms is a surgical emergency and is often accompanied with acute pain with or without hypotension. Although rupture of an ascending aortic aneurysm may cause cardiac tamponade, rupture in the descending thoracic aorta may cause hemothorax, aortobronchial fistula, or aortoesophageal fistula.

Diagnostic Imaging for Thoracic Aortic Aneurysms
The chest radiograph may suggest a thoracic aortic aneurysm with features such as a widened mediastinum, enlarged aortic knob, dilated descending thoracic aorta, aortic calcifications, leftward tracheal deviation, upward deviation of the left mainstem bronchus, and/or new left pleural effusion. Typically, transthoracic echocardiography can provide a reasonable examination of the thoracic aorta, although the acoustic windows are limited by the lungs. The contemporary imaging modalities of choice are CT, MRI, and TEE. Computed tomographic angiography (CTA) images the thoracic aorta during the arterial phase of an intravenous radiocontrast agent injection. It defines vascular anatomy and surrounding nonvascular structures. Aneurysm leak is detected as extravascular contrast extravasation. This imaging modality has multiple advantages such as high resolution, wide availability, rapid acquisition, imaging in patients with metallic implants, and generation of volumetric aortic images for stent design. Because CTA requires iodinated contrast agents, it carries a risk for contrast nephropathy that can be attenuated by administration of acetylcysteine and sodium bicarbonate.22 (See Chapters 2 and 3.)
Contrast-enhanced magnetic resonance angiography with gadolinium also images the entire thoracic aorta in fine detail. Although the spatial resolution of magnetic resonance angiography is slightly inferior to CTA, it does allow for degrees of tissue and fluid characterization. The disadvantages of magnetic resonance angiography include its limited availability, lack of imaging in patients with metallic implants, imaging difficulty in the setting of continuous hemodynamic monitoring, and the time required for image acquisition. Its advantages are the avoidance of ionizing radiation and the lack of renal toxicity.1,17
TEE can image the thoracic aorta from the aortic valve to the distal ascending aorta and from the distal aortic arch to the proximal abdominal aorta. The distal ascending aorta and proximal aortic arch cannot be reliably imaged by TEE because the intervening trachea and left mainstem bronchus obstruct the acoustic window; this is known as the “blind spot” of TEE.23 It is possible to overcome this blind spot with modalities such as imaging across the trachea temporarily filled with a saline-filled balloon (the A-view) and utilizing an expanded aortic view.23,24 The advantages of TEE include its portability, its real-time interpretation, its compatibility at the bedside and in the OR, and its multiple imaging modalities for complete aortic and cardiac assessment. Its disadvantages include the requirement for sedation or general anesthesia and the risks for upper gastrointestinal injury.25

Surgical Considerations for Thoracic Aortic Aneurysms
Surgical repair aims to replace the aortic aneurysm with a tube graft to prevent further aneurysmal complications (Table 21-5). The first indication for thoracic aortic aneurysm resection is whenever the aneurysm is symptomatic regardless of size (ACC/AHA Class I recommendation; level of evidence C).1,17 Symptoms often herald the onset of rupture or dissection and should be interpreted as an urgent indication for surgery. A symptomatic presentation occurs in about 5% of patients. Unfortunately, the first symptom in the remaining 95% of patients often is death.
TABLE 21-5 Indications for Surgical Repair of Thoracic Aortic Aneurysms
Atherosclerotic aneurysm diameter | |
Ascending aorta | ≥5.5 cm |
Descending aorta | ≥6.5 cm |
Marfan’s or familial thoracic aneurysm diameter | |
Ascending aorta | ≥5.0 cm |
Descending aorta | ≥6.0 cm |
Severe aortic regurgitation | |
Aortoannular ectasia with aortic root aneurysm | |
Rupture | |
Refractory pain |
The second indication for resection is aortic diameter. In the ascending aorta, a diameter of 6.0 cm is the critical hinge point after which the risk for aneurysm rupture increases exponentially. Consequently, surgical resection is recommended in the ascending aorta when the diameter reaches 5.5 cm (ACC/AHA Class I recommendation; level of evidence C).1,17 In patients with genetically mediated aortopathies (Marfan syndrome, bicuspid aortic valve, familial thoracic aortic aneurysm or dissection; vascular Ehlers-Danlos syndrome; and Turner syndrome), surgical resection is recommended at a lower ascending aortic diameter of 5.0 cm (ACC/AHA Class I recommendation; level of evidence C).1,17 Ascending aortic aneurysms with diameters less than 5.5 cm but with an annual growth rate in diameter greater than 0.5 cm/yr qualify for surgical resection (ACC/AHA Class I recommendation; level of evidence C).1 It is reasonable to consider prophylactic replacement of the aortic root and ascending aorta in a woman with Marfan syndrome who is planning a pregnancy and who has an aortic root or ascending aortic diameter larger than 4.0 cm (ACC/AHA Class IIa recommendation; level of evidence C).1 In adults with the aggressive aortopathy of the Loeys-Dietz syndrome, it is reasonable to consider proximal thoracic aortic repair when the internal aortic diameter exceeds 4.2 cm (ACC/AHA Class IIa recommendation; level of evidence C).1,26 The ascending aortic aneurysm diameter also must be indexed to body size.27 For example, if the maximal cross-sectional area of the aortic root or ascending aorta (in square centimeters) divided by the patient’s height (in meters) exceeds a ratio of 10, then surgical repair is a reasonable option (ACC/AHA Class IIa recommendation; level of evidence C).1 The rationale behind indexing the aortic dimensions to body size is that shorter adults dissect and rupture their aortas at smaller diameters.1,27 Furthermore, those patients who are undergoing open aortic valve procedures and who have an aortic root or ascending aortic diameter larger than 4.5 cm should be considered for concomitant aortic replacement resection (ACC/AHA Class I recommendation; level of evidence C).1
The hinge point for rupture in the descending thoracic aorta is a diameter of 7.0 cm.17 Consequently, surgical resection is recommended in thoracoabdominal aneurysms when the aortic diameter exceeds 6.0 cm or less when it is associated with a connective tissue disorder such as Marfan syndrome (ACC/AHA Class I recommendation; level of evidence C).1 In patients who have aneurysmal degeneration of the descending thoracic aorta associated with prior dissection and/or a connective tissue disorder, surgical resection is recommended when the aortic diameter is more than 5.5 cm (ACC/AHA Class I recommendation; level of evidence B).1 Patients with aneurysms of the descending thoracic aorta should be considered for thoracic endovascular aortic repair (TEVAR) when technically feasible (ACC/AHA Class I recommendation; Level of Evidence B).1,20

Surgical Repair of Ascending Aortic and Arch Aneurysms
The type of surgical repair depends on aortic valve function and the aneurysm extent. Perioperative TEE can evaluate the aortic valve structure and function to guide and assess the surgical intervention (reimplantation, repair, replacement). Furthermore, TEE can assess the diameters of the aortic root, ascending aorta, and aortic arch to guide intervention. The most common aortic valve diseases associated with ascending aortic aneurysm are bicuspid aortic valve or AR caused by dilation of the aortic root (Figure 21-1). If the aortic valve and aortic root are normal, a simple tube graft can be used to replace the ascending aorta. If the aortic valve is diseased but the sinuses of Valsalva are normal, an aortic valve replacement combined with a tube graft for the ascending aorta without need for reimplantation of the coronary arteries can be performed (Wheat procedure; Figure 21-2; ACC/AHA class I recommendation; level of evidence C).1
If disease also involves the aortic valve and the aortic root, the patient requires aortic root replacement and aortic valve intervention. If technically feasible, the aortic valve can be reimplanted with a modified David technique, which includes graft reconstruction of the aortic root with reimplantation of the coronary arteries (ACC/AHA Class I recommendation; level of evidence C).1,28 If not feasible, aortic root replacement with a composite valve-graft conduit is indicated (Bentall procedure; Figure 21-3; ACC/AHA Class I recommendation; level of evidence C).1 Aortic root replacement requires coronary reimplantation or aortocoronary bypass grafting (Cabrol technique; Figure 21-4).
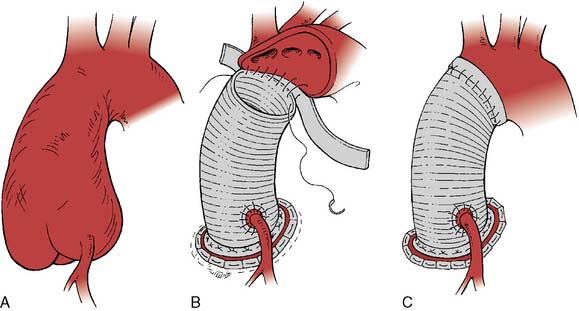
Figure 21-3 Replacement of the entire aortic root with a composite valved conduit for ascending aortic aneurysm.
(Adapted from Griepp RB, Ergin A: Aneurysms of the aortic arch. In Edmunds LH [ed]: Cardiac Surgery in the Adult. New York, McGraw-Hill, 1997, p 1209, by permission.)
Repairing aortic aneurysms that extend into or involve the aortic arch requires CPB with DHCA with or without perfusion adjuncts. For ascending aortic aneurysms that involve only the proximal aortic arch, partial arch replacement (hemiarch technique) is reasonable in which a tubular graft is interposed between the ascending aorta or aortic root and the underside of the aortic arch (ACC/AHA Class IIa recommendation; level of evidence B).1 Ascending aorta with hemiarch reconstruction often is performed using DHCA with or without ACP/RCP to make the distal anastomosis feasible without cross-clamping (“open technique”). In patients who have isolated aortic arch aneurysms and who have a low operative risk, arch replacement is reasonable when the arch diameter exceeds 5.5 cm (ACC/AHA Class IIa recommendation; level of evidence B).1 Total aortic arch replacement is reasonable in aneurysms that involve the entire arch (ACC/AHA Class IIa recommendation; level of evidence B).1 Ascending aortic aneurysms that extend through the aortic arch into the descending aorta can be repaired with the “elephant trunk” technique (Figure 21-5; ACC/AHA Class IIa recommendation; level of evidence B).1,29 Aortic arch aneurysms that extend into the aortic branch vessels may require repair with branched or trifurcated tube grafts to permit separate anastomosis to the innominate, left carotid, and left subclavian arteries.30 In patients with aortic arch aneurysms and concomitant severe comorbidity, recent guidelines support an endovascular repair technique (Class IIb recommendation; level of evidence C).1,20 However, in patients who have aortic arch aneurysms and who have reasonable surgical risk, the recent guidelines advise against an endovascular repair technique (Class III recommendation; level of evidence A).1,20

Anesthetic Management for Ascending Aorta and Arch Aneurysms
The conduct of general anesthesia in this setting has specific concerns. The imaging studies should be reviewed for aneurysm compression of mediastinal structures such as the right pulmonary artery and left mainstem bronchus (Figure 21-6). Prevention of hypertension increases forward flow in AR and minimizes the risk for aneurysm rupture. A right radial arterial catheter is preferred for most cases. If arterial cannulation of the right axillary, subclavian, or innominate artery is planned for CPB and ACP, bilateral radial arterial catheters often are required to measure cerebral and systemic perfusion pressures. Nasopharyngeal, tympanic, and bladder temperatures are important for estimating brain and core temperatures for monitoring the conduct of DHCA. Monitoring of jugular bulb venous oxygen saturation and the EEG may reflect cerebral metabolic activity to guide the conduct of DHCA. Intraoperative TEE is essential to guide and assess the surgical interventions. In patients with AR, TEE can assist in the conduct of CPB by guiding placement of cannulae such as the retrograde cardioplegia cannula (coronary sinus) and by monitoring left ventricular (LV) volume to ensure that the LV drainage cannula keeps the ventricle collapsed. Intraoperative TEE is reasonable in thoracic aortic procedures, including endovascular interventions, in which it assists in hemodynamic monitoring, procedural guidance, and endoleak detection (ACC/AHA Class IIa recommendation; level of evidence B).1

Neuroprotection Strategies for Temporary Interruption of Cerebral Blood Flow
The risk for stroke is substantial during the cerebral ischemia that accompanies aortic arch reconstruction.31 The first mechanism is cerebral ischemia due to hypoperfusion or temporary circulatory arrest during aortic arch repair. The second mechanism is cerebral ischemia due to embolization secondary to CPB and atheroma. Arterial emboli causes include air introduced into the circulation from open cardiac chambers, vascular cannulation sites, or arterial anastomosis. Atherosclerotic particulate debris may be released during clamping and unclamping of the aorta, the creation of anastomoses in the ascending aorta and aortic arch, or the excision of severely calcified and diseased cardiac valves. CPB may result in the microparticulate aggregates of platelets and fat. The turbulent high-velocity blood flow out of the aortic cannula used for CPB also may dislodge atherosclerotic debris within the aorta. Retrograde blood flow through a diseased descending thoracic aorta as a consequence of CPB conducted with femoral artery cannulation may cause retrograde cerebral embolization. For all these reasons, strategies to provide neurologic protection are essential in thoracic aortic operations (Box 21-2).
Deep Hypothermic Circulatory Arrest
The brain is exquisitely susceptible to ischemic injury within minutes after the onset of circulatory arrest because it has a high metabolic rate, continuous requirement for metabolic substrate, and limited reserves of high-energy phosphates. The physiologic basis for deep hypothermia as a neuroprotection strategy is to decrease cerebral metabolic rate and oxygen demands to increase the period that the brain can tolerate circulatory arrest.32 Existing evidence indicates that autoregulation of cerebral blood flow is maintained during deliberate hypothermia with alpha-stat blood gas management without compromise of clinical outcome.33 Direct measurement of cerebral metabolites and brainstem electrical activity in adults undergoing DHCA with RCP at 14°C indicated the onset of cerebral ischemia after only 18 to 20 minutes (Figure 21-7).34 Despite this observation, the large body of experimental evidence and clinical experience with the deliberate hypothermia suggest that it is the single most important intervention for preventing neurologic injury in response to circulatory arrest.
Despite the proven efficacy of hypothermia for operations that require circulatory arrest, no consensus exists on an optimal protocol for the conduct of deliberate hypothermia for circulatory arrest. A strategy to protect the brain during aortic arch surgery must be a high priority in the perioperative management of these procedures to prevent stroke and optimize cognitive function (ACC/AHA Class I recommendation; level of evidence C).1 Although the average nasopharyngeal temperature for DHCA may be about 18° C, the optimal temperature for DHCA has not been established.31,32 A challenge in the selection of the ideal temperature for DHCA is the inability to directly measure the brain temperature. In an EEG-based approach to this question, the median nasopharyngeal temperature for electrocortical silence was 18° C, although a nasopharyngeal temperature of 12.5° C or cooling on CPB for at least 50 minutes achieved electrocortical silence in 99.5% of cases (Figure 21-8).35 Although the EEG functions well as a physiologic end point for cerebral metabolic suppression during cooling for DHCA as part of an institutional protocol, its outcome benefit remains to be demonstrated in a randomized trial.15,31,36 A jugular bulb venous oxygen saturation greater than 95% measured using an oximetric catheter represents an alternative physiologic end point to detect maximum cerebral metabolic suppression for DHCA.37 It is important to note that deep hypothermia to a set temperature (mean = 19°C) as an end point for DHCA without EEG or jugular bulb venous oxygen saturation has been associated with excellent neurologic outcomes in recent series.38,39 In addition to systemic hypothermia produced by extracorporeal circulation, topical hypothermia by packing the head in ice also has been incorporated in leading institutional DHCA protocols to minimize passive warming of the head.38,39 When providing topical hypothermia, care should be exercised to protect the eyes, nose, and ears from frostbite by protecting these vulnerable areas. Recent clinical studies support the practice of limiting the duration of straight DHCA to shorter than 45 minutes to avoid the associated significant increases in stroke and mortality risks.31 The technique of DHCA alone is a reasonable approach for neuroprotection during aortic arch surgery in the setting of adequate institutional experience (ACC/AHA Class IIa recommendation; level of evidence B).1
The conduct of DHCA extends CPB duration with consequent risks for coagulopathy and embolization. Rewarming increases cerebral metabolic rate and can aggravate neuronal injury during ischemia/reperfusion. Consequently, it is important to rewarm gradually by maintaining a temperature gradient of no more than 10° C in the heat exchanger and avoiding cerebral hyperthermia (nasopharyngeal temperature > 37.5°C). The current guidelines advise against cerebral hyperthermia in aortic arch procedures (ACC/AHA Class III recommendation; level of evidence B).1

Retrograde Cerebral Perfusion
RCP is performed by infusing cold oxygenated blood into the superior vena cava cannula at a temperature of 8° C to 14° C via CPB (Figure 21-9). The internal jugular venous pressure is maintained at less than 25 mm Hg to prevent cerebral edema. Internal jugular venous pressure is measured from the introducer port of the internal jugular venous cannula at a site proximal to the superior vena cava perfusion cannula and zeroed at the level of the ear. The patient is positioned in 10 degrees of Trendelenburg to decrease the risk for cerebral air embolism and prevent trapping of air within the cerebral circulation in the presence of an open aortic arch. RCP flow rates of 200 to 600 mL/min usually can be achieved. The potential benefits of RCP include partial supply of cerebral metabolic substrate, cerebral embolic washout, and maintenance of cerebral hypothermia.40 Although RCP has been associated with excellent clinical results in aortic arch repair, it has not become the standard technique for neuroprotection in DHCA.15,41 A recent large, single-center study (1991–2007; N = 1107; RCP in 82%) evaluated the role of RCP in proximal thoracic aortic repair. The perioperative rates for mortality and stroke in this series were 10.4% and 2.8%, respectively. The application of RCP was significantly protective against mortality (odds ratio, 0.42; 95% confidence interval, 0.25-0.70; P = 0.0009) and stroke (odds ratio, 0.35; 95% confidence interval, 0.15-0.81; P = 0.02).42 Despite the lack of randomized trials, RCP is safe and easily implemented in aortic arch repair as an adjunct to maintain cerebral hypothermia, provide partial metabolic substrate delivery, and decrease the risk for cerebral embolization.36–42 The technique of DHCA with RCP is a reasonable approach for neuroprotection during aortic arch surgery in the setting of adequate institutional experience (ACC/AHA Class IIa recommendation; level of evidence B).1
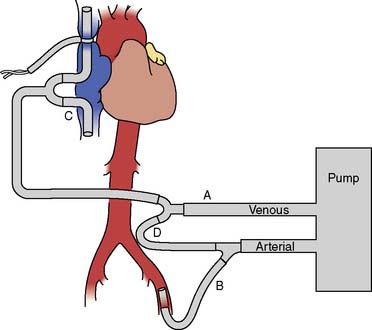
Figure 21-9 Extracorporeal perfusion circuit used to deliver retrograde cerebral perfusion.
(Adapted from Bavaria JE, Woo YJ, Hall RA, et al: Retrograde cerebral and distal aortic perfusion during ascending and thoracoabdominal aortic operations. Ann Thorac Surg 60:347, 1995, by permission of the Society of Thoracic Surgeons.)
Selective Antegrade Cerebral Perfusion
Selective ACP should be considered for aortic arch repairs longer than 45 minutes.31 ACP typically is initiated during DHCA by selective cannulation of the right axillary artery, right subclavian artery, innominate artery, or left common carotid artery (Figure 21-10).43 In transverse aortic arch reconstruction procedures, ACP can be accomplished by inserting individual perfusion cannulae into the open end of the aortic branch vessels after opening the aortic arch. After reattachment of the aortic arch branch vessels to the vascular graft, ACP can be provided through a separate arm of the vascular graft or by direct cannulation of the graft. A functional circle of Willis may provide contralateral brain perfusion during interruption of antegrade perfusion in the innominate, left carotid, or left subclavian arteries during construction of the vascular anastomoses. ACP with oxygenated blood at 10° C to 14° C at flow rates in the range of 250 to 1000 mL/min typically achieves a cerebral perfusion pressure in the range of 50 to 80 mm Hg.
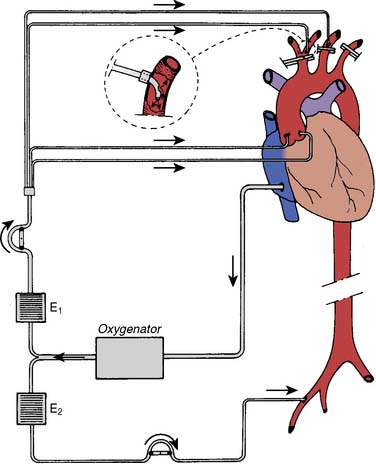
Figure 21-10 Extracorporeal perfusion circuit used for selective antegrade cerebral perfusion.
(From Bachet J, Teodori G, Goudot B, et al: Replacement of the transverse aortic arch during emergency operations for type A acute aortic dissection. J Thorac Cardiovasc Surg 96:878, 1988.)
Unilateral ACP via right axillary arterial cannulation is a popular technique for adult aortic repair.43 This technique assumes an adequate circle of Willis; however, the anatomic completeness of the circle of Willis does not guarantee adequate cerebral cross-perfusion during aortic arch repair.44,45 Consequently, it remains essential to monitor the contralateral hemisphere in unilateral ACP with modalities such as cerebral oximetry, carotid artery scanning, and transcranial Doppler.46–48
Given that ACP may be unilateral or bilateral, there remains controversy about which ACP technique is superior.49 A recent literature review combined 17 studies for a total sample size of 3548 patients; 83.1% with bilateral ACP and 16.9% with unilateral ACP.50 Although the stroke rates were less than 5% regardless of technique, the period of safe ACP was significantly prolonged with bilateral ACP (86–164 minutes) compared with unilateral ACP (30–50 minutes). The evidence favors bilateral ACP in the setting of aortic arch repair times longer than 60 minutes.50
Pilot clinical series in adult aortic arch repair also have been undertaken in the setting of ACP with moderate hypothermic circulatory arrest (MHCA; systemic temperature = 25° C).51,52 A large, single-center study (1999–2006; N = 501 [36.1% emergency cases]; median age, 64 years; 63.9% male sex) evaluated perioperative outcomes with this technique.53 With a perioperative mortality rate of 11.6%, multivariate predictors for mortality included age and CPB time. The stroke rate was 9.6% with operative time and renal dysfunction as its multivariate predictors for stroke. The rate of temporary neurologic dysfunction was 13.4%, its multivariate predictors including MHCA duration (odds ratio, 1.015; p = 0.01). Although MHCA with cold ACP appears to be an adequate technique for adult aortic arch repair, its safety is limited in the settings of the elderly, multiple comorbidities, and extended operative time. The safety of aortic arch repair recently was further demonstrated with bilateral ACP and a greater mean MHCA temperature of 28° C (2002–2008; N = 229; mean age, 70.8 ± 9.7 years; 68.1% male sex).54 Although MHCA with ACP appears to be a reasonable technique for adult aortic arch repair, its safety for ischemic protection of the spinal cord and kidney are still questioned.55,56 The technique of DHCA with ACP is a reasonable approach for neuroprotection during aortic arch surgery in the setting of adequate institutional experience (ACC/AHA Class IIa recommendation; level of evidence B).1
Pharmacologic Neuroprotection Strategies for Deep Hypothermic Circulatory Arrest
There are no proven pharmacologic regimens that have demonstrated effectiveness for decreasing the risk or severity of neurologic injury in the setting of thoracic aortic operations.36 The agents that have been reported in aortic arch series include thiopental, propofol, steroids, magnesium sulfate, and lidocaine.15,32,57 Furthermore, there is considerable variation in practice with these agents in aortic arch repair.57 In general, the existing evidence suggests that pharmacologic neuroprotection should be considered as a neuroprotective adjunct and not a substitute for hypothermia to protect against cerebral ischemia in the setting of hypoperfusion. The technique of DHCA with pharmacologic adjuncts is a reasonable approach for neuroprotection during aortic arch surgery in the setting of an institutional protocol and adequate institutional experience (ACC/AHA Class IIa recommendation; level of evidence B).1

Descending Thoracic and Thoracoabdominal Aortic Aneurysms
Surgical therapy for thoracic and TAAAs is to replace aneurysmal aorta with a prosthetic tube graft. Surgical access is via lateral thoracotomy or thoracoabdominal incision. Despite recent advances, major surgical challenges remain because the typical patient is elderly with multiple significant comorbidities (Table 21-6). The risks for spinal, mesenteric, renal, and lower extremity ischemia are significant due to thromboembolism, loss of collateral vascular networks, temporary interruption of blood flow, and reperfusion injury. The risks for wound dehiscence and respiratory failure remain significant because of the large incisions and diaphragmatic division, as well as injuries to the phrenic and recurrent laryngeal nerves. Consequently, TAAA repair is high risk (Table 21-7).58
TABLE 21-6 Preoperative Features in Patients with Thoracoabdominal Aortic Aneurysm (N = 1220)
Characteristic | No. of Patients (%) |
---|---|
Crawford extent | |
Extent I | 423 (34.7) |
Extent II | 371 (30.4) |
Extent III | 201 (16.5) |
Extent IV | 225 (18.4) |
Acute dissection | 46 (3.8) |
Chronic dissection | 272 (22.3) |
Marfan syndrome | 72 (5.9) |
Symptomatic aneurysms | 855 (70.1) |
Acute presentation | 112 (9.2) |
Rupture | 76 (6.2) |
Preoperative paraplegia or paraparesis | 16 (1.3) |
Concurrent aneurysm | 224 (18.4) |
Prior aneurysm repair | 502 (41.2) |
Prior thoracic aortic aneurysm repair | 281 (23.0) |
Diabetes | 69 (5.7) |
Hypertension | 940 (77.1) |
Coronary artery disease | 435 (35.7) |
Prior coronary artery bypass or angioplasty | 202 (16.6) |
Cerebrovascular disease | 135 (11.1) |
Renal arterial occlusive disease | 312 (25.6) |
Renal insufficiency | 151 (12.4) |
Chronic obstructive lung disease | 491 (40.3) |
Peptic ulcer disease | 83 (6.8) |
Data from Coselli JS, LeMaire SA, Miller CC 3rd, et al: Mortality and paraplegia after thoracoabdominal aortic aneurysm repair: A risk factor analysis. Ann Thorac Surg 69:409, 2000.
TABLE 21-7 Clinical Outcomes of Thoracoabdominal Aortic Aneurysm (TAAA) Repair (United States: 1988–1998)
Complication | Intact TAAA (n = 1542) | Ruptured TAAA (n = 321) |
---|---|---|
Cardiac | 14.8% | 18.1% |
Pulmonary | 19.0% | 12.7% |
Hemorrhage | 12.4% | 10.9% |
Acute renal failure | 14.2% | 28.0% |
Paraplegia | —* | 3.4% |
Any complication | 55.2% | 51.7% |
In-hospital mortality | 22.3% | 53.8% |
TAAA, thoracoabdominal aortic aneurysm.
* Incidence of paraplegia not reported.
Data from Cowan JA, Dimick JB, Henke PK, et al: Surgical treatment of intact thoracoabdominal aortic aneurysms in the United States: Hospital and surgeon volume-related outcomes. J Vasc Surg 37:1169, 2003; and Cowan JA, Dimick JB, Wainess RM, et al: Ruptured thoracoabdominal aortic aneurysm treatment in the United States: 1988 to 1998. J Vasc Surg 38: 312, 2003.
Aneurysms of the descending thoracic aorta are classified by considering which third(s) of the descending thoracic aorta is (are) involved.1 Extent A involves the proximal third, extent B involves the middle third, and extent C involves the distal third. If more than one third is involved, then the extent is classified according to which thirds are involved, for example, an aneurysm involving the proximal two-thirds is classified as extent AB. Essentially, multisegment aneurysms can be classified as proximal or distal because these extents influence the risk for spinal cord ischemia after surgical repair, whether open or endovascular.
Aneurysms of the thoracoabdominal aorta typically are defined by the Crawford classification (Figure 21-11). Extent I TAAA begins at the left subclavian artery and ends below the diaphragm, but above the renal arteries. Extent II TAAA involves the entire descending thoracic aorta and ends below the diaphragm at the aortic bifurcation. Extent III TAAA begins in the lower half of the descending thoracic aorta and ends below the diaphragm at the aortic bifurcation. Extent IV TAAA is confined to the entire abdominal aorta. If an extent I or extent II TAAA involves the distal aortic arch, its surgical replacement often requires DHCA for the proximal anastomosis. The Crawford classification stratifies operative risk and guides perioperative management (Table 21-8). Open repair of TAAA typically is accomplished by one of three major techniques; (1) aortic cross-clamping, (2) aortic cross-clamping with a Gott shunt, and (3) aortic cross-clamping with PLHB or partial CPB (Figure 21-12).
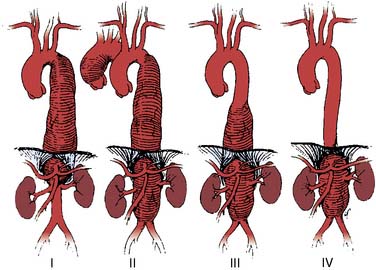
Figure 21-11 Crawford classification of thoracoabdominal aortic aneurysm extent.
(From Coselli JS: Descending thoracoabdominal aortic aneurysms. In Edmunds LH [ed]: Cardiac Surgery in the Adult. New York, McGraw-Hill, 1997, p 1232.)