CHAPTER 1 The History and Impact of Genetics in Medicine
Presenting historical truth is at least as challenging as the pursuit of scientific truth and our view of human endeavors down the ages is heavily biased in favor of winners—those who have conquered on military, political, or, indeed, scientific battlefields. The history of genetics in relation to medicine is one of breathtaking discovery from which patients and families already benefit hugely, but in the future success will be measured by ongoing progress in translating discoveries into both treatment and prevention of disease. As this takes place, we should not neglect looking back with awe at what our forebears achieved with scarce resources and sheer determination, sometimes aided by serendipity, in order to lay the foundations of this dynamic science. A holistic approach to science can be compared with driving a car: without your eyes on the road ahead, you will crash and make no progress; however, the competent driver will glance in the rear and side mirrors regularly to maintain control.
Gregor Mendel and the Laws of Inheritance
Early Beginnings
Early Greek philosophers and physicians such as Aristotle and Hippocrates concluded, with typical masculine modesty, that important human characteristics were determined by semen, using menstrual blood as a culture medium and the uterus as an incubator. Semen was thought to be produced by the whole body; hence bald-headed fathers would beget bald-headed sons. These ideas prevailed until the seventeenth century, when Dutch scientists such as Leeuwenhoek and de Graaf recognized the existence of sperm and ova, thus explaining how the female could also transmit characteristics to her offspring.
Our present understanding of human genetics owes much to the work of the Austrian monk Gregor Mendel (1822–1884; Figure 1.1) who, in 1865, presented the results of his breeding experiments on garden peas to the Natural History Society of Brünn in Bohemia (now Brno in the Czech Republic). Shortly after, Mendel’s observations were published by that association in the Transactions of the Society, where they remained largely unnoticed until 1900, some 16 years after his death, when their importance was first recognized. In essence, Mendel’s work can be considered as the discovery of genes and how they are inherited. The term gene was first coined in 1909 by a Danish botanist, Johannsen, and was derived from the term ‘pangen’ introduced by De Vries. This term was itself a derivative of the word ‘pangenesis,’ coined by Darwin in 1868. In acknowledgement of Mendel’s enormous contribution, the term mendelian is now part of scientific vocabulary, applied both to the different patterns of inheritance shown by single-gene characteristics and to disorders found to be the result of defects in a single gene.
In his breeding experiments, Mendel studied contrasting characters in the garden pea, using for each experiment varieties that differed in only one characteristic. For example, he noted that when strains bred for a feature such as tallness were crossed with plants bred to be short all of the offspring in the first filial or F1 generation were tall. If plants in this F1 generation were interbred, this led to both tall and short plants in a ratio of 3 : 1 (Figure 1.2). Characteristics that were manifest in the F1 hybrids were referred to as dominant, whereas those that reappeared in the F2 generation were described as being recessive. On reanalysis it has been suggested that Mendel’s results were ‘too good to be true’ in that the segregation ratios he derived were suspiciously closer to the value of 3 : 1 than the laws of statistics would predict. One possible explanation is that he may have published only those results that best agreed with his preconceived single-gene hypothesis. Whatever the truth of the matter, events have shown that Mendel’s interpretation of his results was entirely correct.
An alternative method for determining genotypes in offspring involves the construction of what is known as a Punnett square (Figure 1.3). This is used further in Chapter 8 when considering how genes segregate in large populations.
The Law of Segregation
The law of segregation refers to the observation that each person possesses two genes for a particular characteristic, only one of which can be transmitted at any one time. Rare exceptions to this rule can occur when two allelic genes fail to separate because of chromosome non-disjunction at the first meiotic division (p. 43).
The Law of Independent Assortment
The law of independent assortment refers to the fact that members of different gene pairs segregate to offspring independently of one another. In reality, this is not always true, as genes that are close together on the same chromosome tend to be inherited together, because they are ‘linked’ (p. 136). There are a number of other ways by which the laws of mendelian inheritance are breached but, overall, they remain foundational to our understanding of the science.
The Chromosomal Basis of Inheritance
As interest in mendelian inheritance grew, there was much speculation as to how it actually occurred. At that time it was also known that each cell contains a nucleus within which there are several threadlike structures known as chromosomes, so called because of their affinity for certain stains (chroma = color, soma = body). These chromosomes had been observed since the second half of the nineteenth century after development of cytologic staining techniques. Human mitotic figures were observed from the late 1880s, and it was in 1902 that Walter Sutton, an American medical student, and Theodour Boveri, a German biologist, independently proposed that chromosomes could be the bearers of heredity (Figure 1.4). Subsequently, Thomas Morgan transformed Sutton’s chromosome theory into the theory of the gene, and Alfons Janssens observed the formation of chiasmata between homologous chromosomes at meiosis. During the late 1920s and 1930s, Cyril Darlington helped to clarify chromosome mechanics by the use of tulips collected on expeditions to Persia. It was during the 1920s that the term genome entered the scientific vocabulary, being the fusion of genom (German for ‘gene’) and ome from ‘chromosome’.
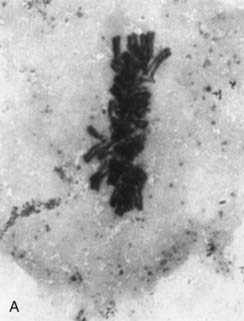
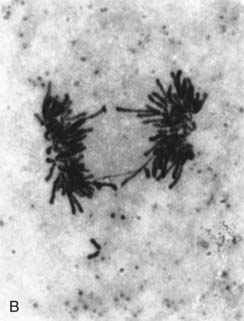
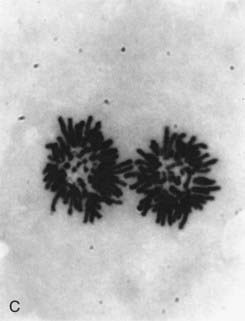
FIGURE 1.4 Chromosomes dividing into two daughter cells at different stages of cell division. A, Metaphase; B, anaphase; C, telophase. The behavior of chromosomes in cell division (mitosis) is described at length in Chapter 3.
(Photographs courtesy Dr. K. Ocraft, City Hospital, Nottingham.)
When the connection between mendelian inheritance and chromosomes was first made, it was thought that the normal chromosome number in humans might be 48, although various papers had come up with a range of figures. The number 48 was settled on largely as a result of a paper in 1921 from Theophilus Painter, an American cytologist who had been a student of Boveri. In fact, Painter himself had some preparations clearly showing 46 chromosomes, even though he finally settled on 48. These discrepancies were probably from the poor quality of the material at that time; even into the early 1950s, cytologists were counting 48 chromosomes. It was not until 1956 that the correct number of 46 was established by Tjio and Levan, 3 years after the correct structure of DNA had been proposed. Within a few years, it was shown that some disorders in humans could be caused by loss or gain of a whole chromosome as well as by an abnormality in a single gene. Chromosome disorders are discussed at length in Chapter 18. Some chromosome aberrations, such as translocations, can run in families (p. 44), and are sometimes said to be segregating in a mendelian fashion.
DNA as the Basis of Inheritance
Whilst James Watson and Francis Crick are justifiably credited with discovering the structure of DNA in 1953, they were attracted to working on it only because of its key role as the genetic material, as established in the 1940s. Formerly many believed that hereditary characteristics were transmitted by proteins, until it was appreciated that their molecular structure was far too cumbersome. Nucleic acids were actually discovered in 1849. In 1928 Fred Griffith, working on two strains of Streptococcus, realized that characteristics of one strain could be conferred on the other by something that he called the transforming principle. In 1944, at the Rockefeller Institute in New York, Oswald Avery, Maclyn McCarty, and Colin MacLeod identified DNA as the genetic material while working on the pneumococcus (Streptococcus pneumoniae