Chapter 21 The Electrophysiological Laboratory
Technologic Advances and Future Development
Newly Designed or “Retrofit”?
Many considerations need to be taken into account when deciding to either design a state-of-the-art cath lab in a newly constructed area or renovating an older lab with up-to-date technologies (Figure 21-1). One of the biggest problems is having enough space to fit not only all the equipment necessary for the procedure (Figure 21-2) but also equipment needed only in an emergency. In addition, ample space for moving the patient into and out of the room via stretcher or patient bed and sufficient in-room storage are essential. Normal items such as telephone lines, a data point for the hospital information system (HIS), sufficient lighting, and easy access from the sink to the sterile field are involved as well. The placement of gases and suction outlets in the procedure room should also be given attention.
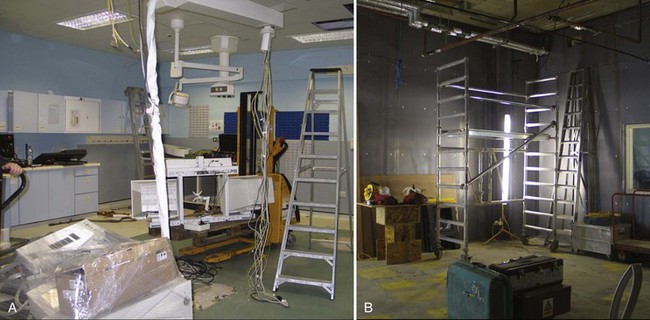
FIGURE 21-1 Retrofit of a conventional catheter laboratory. A, The old equipment is removed. B, The examination room’s walls are fitted with special shielding.
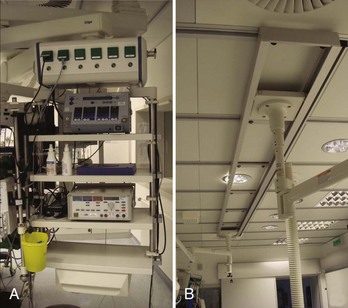
FIGURE 21-2 A, Ceiling-suspended racks allow plenty of storage for generators, patient interface units, and more equipment. Power outlets on top and electrical cabling through cabling tunnels inside the ceiling are seen. B, Rails along the ceiling allow for flexible solutions for monitors and racks as well as radiation protection shield and operation lamp.
Minimal Standards for Invasive Electrophysiology (“The Must Have”)
To visualize catheter positioning, a fluoroscopic imaging system with at least a single C-arm is necessary. The system should be programmable to a certain extent to control radiation exposure (Figure 21-3).1 Because comparably less radiation exposure is needed to image the metal electrodes of a catheter compared with the resolution required for lesion assessment in coronary artery interventions, a special setting should be reserved for EP procedures. The C-arm should be rotatable by at least 90 degrees in both the right anterior oblique and the left anterior oblique positions, and cranial or caudal angulations are not often necessary. To reduce radiation exposure to the operator, proper Plexiglas lead protection, both “under the table” (closest position to the x-ray beam) and “over the table” against scattered radiation from the patient is a legal requirement. In addition, the image should, whenever possible, be centered on the chamber of interest and the table should be locked in the optimal position for all angulations to avoid unnecessary radiation exposure. Finally, collimation and “fencing in” of the lung fields should be routine, and any unnecessary metallic devices should be removed from the area to be imaged because they result in an automatic intensification of radiation exposure (e.g., transesophageal echocardiography probes should be removed from view). European laws require the documentation of both total exposure time and dosage of the overall deployed radiation. In regard to applied fluoroscopy, the ALARA (as low as reasonably achievable) principle should be followed, and unnecessary imaging should be avoided.1 If available, the “last image hold” option should be used.2
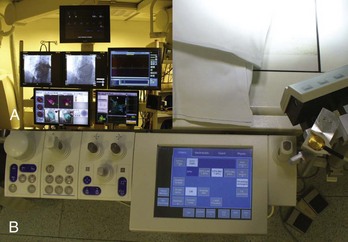
FIGURE 21-3 A, Monitor gantry with six monitors arranged to allow the best orientation for the operator, with live monitors for fluoroscopy and intracardiac signals side by side. B, Controls of the fluoroscopy system (AXIOMS Artis; Siemens, Munich, Germany) positioned at the table side to allow rapid navigation through recorded scenes, again supporting single-operator functionality.
Electrophysiology Recording System (Including Intracardiac Pressure Recordings)
The minimal requirements for EP recording systems are fairly subjective. Although some operators work with only 32 channels, at least 64 channels seems to be the current standard. In addition to the standard display of the 12-lead electrocardiogram (ECG), bipolar and unipolar signals should be programmable.3 The gain applied to any given recording should be individually adjustable, and electrodes belonging to one catheter are usually grouped (e.g., by color). Although the order of the displayed signals is a question of personal preference and training, the signal accuracy is of utmost importance. Too large a noise level is unacceptable because it could mislead the operator during the mapping process. Signal quality during energy application also is important, and every effort should be made to achieve clean signals to allow drag lesions rather than sequential point-by-point applications. In addition to the electrical signals, the recording of at least one invasive pressure should be possible (e.g., for emergency percutaneous transluminal coronary angioplasty or hemodynamic monitoring).