Chapter 191 Surgical Approaches to the Cervicothoracic Junction
Cervicothoracic pathologies are relatively uncommon but include bacterial and tuberculous infections, fractures from primary bone disease, primary bone tumors, meningeal tumors, vascular malformations, congenital connective tissue and skeletal disorders, trauma, and thoracic disc herniations (Table 191-1). Up to 15% of patients with spinal neoplasms have lesions of the upper thoracic vertebrae, and 10% of spinal metastases arise from the T1 to the T4 region.1 One group reported that 8% of patients undergoing tumor resection required posterior instrumentation at the cervicothoracic junction.2 Additionally, as many as 9% of traumatic injuries can involve the cervicothoracic junction.3
TABLE 191-1 Differential Diagnosis of Lesions of the Cervicothoracic Junction
Metastatic tumors |
Primary tumors of bone |
Primary lymphoma |
Intradural, extramedullar tumors |
Intradural, intramedullary tumors |
Bacterial infections |
Tuberculous infections |
Vascular malformations |
Pathologic fracture (primary metabolic disease of bone) |
Connective tissue and skeletal disorders |
Traumatic vertebral fractures |
Disc herniations |
Up to 80% of unstable cervicothoracic pathologies can have neurologic compromise, and many of these lesions require surgical treatment. Unfortunately, injuries to this area are often overlooked on routine radiographic studies.4,5 The surgical goals include neural decompression, immediate stabilization, restoration of anatomic spinal alignment, and early rehabilitation. Appropriate treatment is necessary because acute unstable lesions of the cervicothoracic junction can have severe consequences including poor rates of recovery and significant mortality from cardiopulmonary-related complications.6
Posterior approaches, such as laminectomy and pediculectomy, are common approaches to many diseases of the spine. When treating anterior spinal element diseases, these techniques might not be adequate, can have a higher complication rate, and can disrupt spinal stability.7,8 For these reasons, a variety of anterior and posterolateral surgical approaches have been developed.
The first detailed description of a posterolateral approach to the anterior elements was the costotransversectomy.9 Although this technique gave adequate exposure of the middle and lower thoracic spine, it was less useful in the upper thoracic region. In 1954, Capener10 described a more-extensive posterolateral exposure, the lateral rhachotomy. In 1976, Larson and colleagues11 reported a modification of the lateral rhachotomy, the lateral extracavitary approach, which provided improved exposure of the middle and lower thoracic spine with less morbidity. However, these new additions were still limited at the cervicothoracic junction owing to the shoulder girdle. The lateral parascapular extrapleural operation, a modification of the lateral extracavitary approach, eliminates those obstructions and provides exposure of the thoracic vertebrae up to the inferior end plate of C7.12
Purely anterior, supraclavicular approaches to the cervicothoracic junction were initially described by Jonnesco13 and Brunig14 in 1923 and later used by Royle15 for spastic paralysis, by Gask16 for Raynaud’s disease, and by Ochsner and DeBakey17 for thoracic sympathectomy. However, exposure of the thoracic area was restricted by the clavicle, so the transmanubrial and transclavicular approach was developed by Sundaresan and colleagues18 in 1984 and modified by Birch and colleagues19 in 1990.
Another approach to the anterior thoracic vertebral elements, the anterolateral thoracotomy, was first described by Hodgson and colleagues.20 This approach involves resection of the third rib and requires transpleural mobilization of the lung with ligation and division of the intercostal arteries, intercostal veins, and the hemiazygos vein.
The following sections discuss the clinical features of cervicothoracic junction diseases, including preoperative evaluation, anesthetic considerations, relevant regional surgical anatomy, biomechanics of the cervicothoracic junction, surgical approaches, and options for surgical reconstruction and stabilization.
Clinical Features
The differential diagnosis for cervicothoracic pathologies is listed in Table 191-1. Disease processes in this region can manifest as pain without neurologic deficit, thoracic myelopathy, C7 or T1 radiculopathy, or a combination of these signs and symptoms. Table 191-2 lists the presenting findings in our series of patients with pathologic processes of the cervicothoracic junction (C7 to T4).1 Of these patients, 93% had decreased sensation, 83% presented with generalized upper thoracic back pain, and 58% had leg weakness. C7 and T1 lesions were less common, and consequently, 33% of patients presented with radicular symptoms and 35% demonstrated hand weakness on examination. Other, less common findings included ataxia (25%) and bowel or bladder dysfunction (17%).
TABLE 191-2 Signs and Symptoms at Presentation of Patients with C7-T4 Pathologic Processes (17 Patients)
Signs and Symptoms | Percentage of Patients with Signs or Symptoms |
---|---|
Back pain | 83 |
Radicular pain | 33 |
Leg weakness | 58 |
Decreased sensation | 92 |
Hand weakness | 35 |
Bowel or bladder dysfunction | 17 |
Ataxia | 25 |
Babinski sign | 58 |
Preoperative Evaluation
Radiographic Evaluation
Infections such as osteomyelitis or discitis have a variety of characteristics on plain radiographs. The earliest and most consistent finding of infection is narrowing of the disc space, which is present in 74% of patients.21 After 3 to 6 weeks, destructive changes in the body can be noted, which usually begin as lytic areas in the anterior aspect of the body adjacent to the disc and end plate. Active bone formation and sclerosis is also present in 11% of patients.
Metastatic disease of the bone has several classic signs including unilateral erosion of a pedicle, fishmouthing (cephalad and caudad end plate concavity within the vertebral body), osteoblastic changes, and vertebral body collapse. These findings can occur late in the disease process because 30% to 70% of the bone must be destroyed before changes are visible on plain radiographs.22
Radionucleotide bone scintigraphy is a sensitive but not specific method for detecting metastatic disease and infections.23,24 However, false negatives have been reported with lung cancer, renal cell carcinoma, myeloproliferative diseases, and regional ischemia, and in patients with leukopenia.25–27
Magnetic resonance imaging (MRI) is the most important diagnostic tool for evaluating diseases of the thoracic spine. MRI permits early diagnosis of infection and recognition of paravertebral or intraspinal abscesses without the risk associated with myelography.28 MRI is also effective in demonstrating the extent of metastatic disease, primary tumors of bone, primary lymphoma, intradural tumors, thoracic disc herniations, and vertebral fractures. Additionally, this modality may be helpful in spinal vascular malformations, and when assessing metastatic disease, MRI has been found to be at least as sensitive and accurate as gallium and bone scanning combined.29 Finally, MRI provides anatomic detail, especially of soft tissue and nervous structures, not available with any other imaging studies.
Approach-Related Surgical Anatomy
Lateral Parascapular Extrapleural Approach and Anterolateral Thoracotomy Approach
The lateral parascapular extrapleural approach is a posterolateral surgery that allows nearly lateral access to the cervicothoracic junction vertebral bodies. The anterolateral thoracotomy is a transthoracic approach through the third rib that allows access to the anterior and lateral aspects of the vertebrae and for control of the mediastinal vasculature. The relevant anatomy may be summarized in three major areas: scapula and parascapular anatomy, posterior thoracic cage, and retromediastinal space and spinal anatomy.
Scapular and Parascapular Anatomy
Posterolateral access to the thoracic cage and vertebral elements is hindered by the scapular and the parascapular shoulder musculature (Fig. 191-1). Mobilization of the scapula anterolaterally is necessary and requires the disruption of the posteromedial shoulder musculature.
Posterior Thoracic Cage
The deep or intrinsic muscles including the erector spinae and transversospinalis muscles are next encountered (Fig. 191-2). The erector spinae muscles originate from the sacrum as a dense aponeurotic band and divide into three columns below the last rib as they proceed superiorly. The iliocostalis muscle is located laterally and inserts from the angles of the ribs and into the cervical transverse processes from C4 through C6 as series of related muscular bundles. Each bundle extends over approximately six segments, with the more medial bundles extending more cephalad.
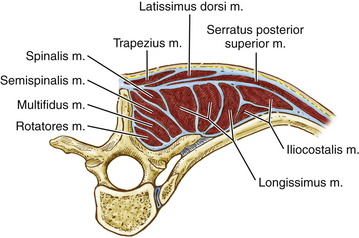
FIGURE 191-2 Cross-sectional anatomy of the deep or intrinsic muscle layer of the upper thoracic spine. M., muscle.
The transversospinalis muscle group passes cephalad from the transverse processes to the spinous processes immediately deep to the erector spinae muscles in three layers. The most superficial layer, the semispinalis, arises from the tips of the transverse processes and inserts at the tips of the spinous processes approximately five vertebral levels cephalad. At the cervicothoracic junction, this muscle is primarily composed of the semispinalis capitis. This muscle passes from the upper thoracic transverse processes and lower cervical articular processes (C4 to T4) to the occipital bone between the superior and inferior nuchal lines. The muscle fibers run vertically and attach to the ligamentum nuchae. The intermediate layer, the multifidus, originates from the erector spinae aponeurosis and from the transverse processes up to C4 and extends to the lower border of each spinous process. This muscle spans approximately three levels. The deepest muscles of this group, the rotatores, are muscles that bridge one interspace. They pass from one transverse process root to the spinous process root immediately above.
Each rib articulates with its own vertebral body and transverse process, the vertebra above, and the intervertebral disc between them (Fig. 191-3), but the first rib articulates only with its own vertebral body. Each of these articulations forms separate synovial joints, and the joints of the posterolateral vertebral body surface are separated by an intra-articular ligament that attaches to the intervertebral disc. An articular capsule surrounds the joints and attaches to the vertebral body anteriorly via the radiate ligament.
Transmanubrial Approach
The transmanubrial approach is an anterior approach that allows direct access to the vertebral bodies and associated pathologies. This approach consists of three major steps: the thoracic inlet, the visceral and vascular compartments of the superior mediastinum, and the retromediastinal space.
Cervicothoracic Biomechanics
The cervicothoracic junction extends from C7 through T4 and includes the lower brachial plexus, the thoracic outlet, and the superior mediastinum. This region also has a narrow spinal canal and narrow pedicles, and it represents a transition from the lateral masses of the cervical vertebra to the transverse processes of the thoracic spine. The structures affecting spinal stability include the vertebral bodies and intervertebral discs, anterior and posterior longitudinal ligaments, and the interarticulating facet joints and ligamentous complex posteriorly.