Chapter 145 Subsidence and Dynamic Cervical Spine Stabilization
Fundamental Concepts
The loss of vertical height with aging has been observed for centuries. This process, resulting in humans losing height after the achievement of adult status, is multifactorial. It essentially involves the loss of disc height and vertebral body collapse.1 Both of these processes may involve deformation along the neutral axis (axial deformation), but often deformation around an axis of rotation (angular deformation) is also involved. Both angular and axial deformities result in loss of vertical height. Gravity and repetitive axial loading contribute to the deformation. Angular deformation most often occurs in the sagittal plane, resulting in kyphosis. This kyphosis results in an applied moment arm. As kyphosis progresses, the moment arm is lengthened, and deformity progresses further.1 This situation is portrayed by the phrase, “kyphosis begets kyphosis.” Kyphosis is usually undesirable. In the cervical region, it places the cervical musculature at a biomechanical disadvantage and may lead to mechanical neck pain. It may also accelerate adjacent segment degenerative changes.2,3 If kyphosis becomes severe, forward gaze and respiration may be adversely affected. Although subsidence occurs in all regions of the spine, subsidence after surgery is most evident in the cervical spine.
Graft Resorption and Remodeling
As a result of all of the aforementioned processes, bone grafts first partially resorb before being replaced by new living bone. The process results in subsidence of the bone graft after surgery. This subsidence is part of the normal biology of bone healing and not a pathologic process. It often is not appreciated in noninstrumented constructs. However, in studies that specifically measure construct height, subsidence is routinely observed.4–6 The amount of subsidence varies with the type of graft used and number of levels fused. Bishop et al.4 showed that in uninstrumented ventral cervical discectomies that used iliac crest autograft, the average settling was 1.4 mm for a single-level construct and 1.8 mm for two-level procedures. The amount of settling increased to 2.4 mm for single levels and 3.0 mm for two levels when iliac crest allograft was used.
Graft Collapse
A cervical interbody graft can collapse before its incorporation, also resulting in subsidence. Graft collapse occurs for numerous reasons. If the graft is inadequately sized to handle the loads placed on it, it may collapse. Collapse can occur if the graft is too narrow in width or depth with respect to the adjacent vertebrae. This size mismatch increases the load placed on a graft. A larger graft spreads the load over a larger area, reducing the load per unit of surface area, and the graft may be able to withstand axial loads more effectively. Finally, if there is a good match between the contours of the surface of the vertebrae and the graft (so-called gapless fusion), the weight-bearing forces are evenly distributed over the region of contact, diminishing the chance of graft collapse.
Pistoning (Subsidence)
Some surgeons have attempted to devise methods of interlocking the grafts and vertebrae when fixation is not used. Other surgeons advocate mortising the graft into the vertebrae, that is, countersinking the graft into the cancellous region of the vertebrae beyond the end plates. This approach minimizes the chance of graft migration or expulsion but at the expense of increasing the chance of subsidence. Other surgeons perforate the end plate in various patterns to encourage vascular ingrowth. However, extensive violation of the end plate, especially when used with dense cortical bone, is likely to increase pistoning. Lim et al.7 showed that it is important to preserve as much end plate as possible to prevent graft subsidence into the vertebral body. They found that making one central hole to increase vascular access to the graft rather than multiple smaller holes reduced stresses on the end plate.
Cervical Plating and Evolution of Dynamic Fixation
First-Generation Systems
The use of such plates helps preserve or restore lordosis, reduces graft extrusion, and improves graft union rates. This use also facilitates the performance of more extensive procedures when indicated by the disease process.8–13 Ventral cervical plating allows the surgeon to use allograft more liberally instead of autograft. Allograft success rates have been shown to equal success rates of autograft when ventral plating was used.14 Use of allograft helped avoid donor site complications, which can be a significant source of patient morbidity (i.e., pain at the graft harvest site).
The initial ventral cervical implants were not perfect, and implant-related complications were observed. The initial Caspar plating system used parallel slots for the screws, which were not locked or constrained to the plate. This was a truly axially dynamic implant. The implant required bicortical screw placement for optimal stability. Despite bicortical placement, screw backout was observed sometimes. The dynamic nature of the implant permitted axial subsidence. However, this subsidence was not recognized at the time as part of the normal biology of bone healing (as already discussed) and was thought to be undesirable. The plate was modified so that holes replaced one half of the slots (Fig. 145-1). Rather than preventing subsidence, this modification led to screw breakage. The screw breakage virtually always involved the screws placed in the holes, which were subjected to excessive bending moments (Fig. 145-2). Fixed in the cortex dorsally and the hole in the plate ventrally, the screws typically fractured in the middle at the point of both the maximum bending moment application and the maximum stress application (stress = bending moment/strength). The screws placed into the slots were able to toggle or slide along the slots and did not fracture.
Second-Generation Systems
In an attempt to prevent the aforementioned complications (presumed pathologic settling, screw backout, and screw fracture) and to avoid the need for bicortical screw purchase, a second generation of cervical fixation systems emerged. These devices featured screws fixed to the implant and permitted screw convergence on placement. The cervical spine locking plate (CSLP) from Synthes was the first such device. The ability to “toe-in” the screws secured the plate in part by triangulation and acted to reduce the chance of screw pull-out (Fig. 145-3). These devices were initially successful, but failures caused by plate or screw fracture, construct pull-out, and delayed union or nonunion were increasingly recognized. Attempts were made to remedy this situation by increasing the strength of the plate; examples are the Orion plate from Sofamor-Danek and the Codman plate (Fig. 145-4). These more rigid implants reduced hardware fracturing but may have increased the incidence of delayed union and pseudarthrosis caused by stress shielding (see later discussion).
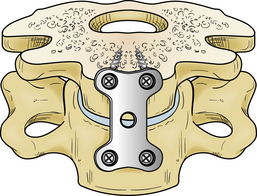
FIGURE 145-3 CSLP (Synthes). Triangulation of the locked unicortical screws was used to increase pull-out strength.
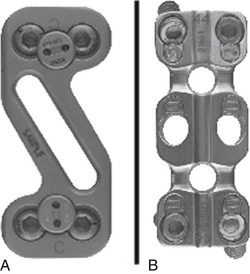
FIGURE 145-4 Orion plate (Sofamor-Danek) (A) and Codman plate (B) were made stronger to try to reduce hardware failures.
Despite their shortcomings, second-generation plating systems have achieved better results than noninstrumented fusions, and they have become quite popular. Multiple variations on this basic theme have been developed as other manufacturers entered the market. Despite the popularity and ease of use of these systems, failures continued to occur. At the time (early 1990s), the spine community did not fully appreciate the reason for these nonunions and failures. Because these were often late sequelae, many were not likely recognized or at least were underreported. Figure 145-5