Chapter 109 Staging, Classification, and Oncologic Approaches for Metastatic Tumors Involving the Spine
The early diagnosis and management of metastatic spine tumors is essential to reducing pain, preserving or improving neurologic function, and improving quality of life. The three primary treatment modalities are radiation therapy (RT), surgery, and chemotherapy. Although these treatment modalities are all essential for treating metastatic spine tumors, prospective trials and prognostic guidelines have yet to definitively delineate the role of any single modality as first-line therapy. The evolution of advanced radiation techniques, such as image-guided (intensity-modulated) radiation therapy (IGRT), and noninvasive percutaneous cement augmentation procedures have added significantly to the armamentarium in the treatment of metastatic spine tumors and increased the complexity of decision making for this patient population. One recent randomized prospective study identified a subpopulation of patients who may benefit from surgery.1 Although decision making for an individual patient remains largely based on institutional experience and the individual preferences of the treating physician,2–3 clinical data and new decision frameworks can help guide therapy.
Decision Frameworks
Traditionally, assessment systems such as the Tomita and Tokuhashi scoring systems have been used to derive treatment for metastatic spine tumors.2 The Tomita score takes into consideration the grade of malignancy and visceral and bone metastases. A low score indicates a good prognosis. The Tokuhashi scoring system is useful for predicting survival and evaluates performance status, tumor histology, neurologic impairment, and the numbers of bone, vertebral body, and visceral metastases.3–5 These scoring systems were useful and validated for surgical outcomes, but the evolution of stereotactic radiosurgery has dramatically changed tumoral responses, making the surgical recommendations from these systems somewhat outdated.
At Memorial Sloan-Kettering Cancer Center (MSKCC), treatment decisions for patients with spine tumors are driven by a conceptual framework referred to as NOMS, which incorporates four fundamental considerations: neurologic (N), oncologic (O), mechanical (M), and systemic (S) disease.6 Neurologic considerations include the presence of myelopathy or functional radiculopathy, as well as the degree of radiographic epidural spinal cord compression. Oncologic issues primarily reflect the ability to achieve local, durable tumor control, and, thus, reflect the radiosensitivity and/or chemosensitivity of the tumor. Mechanical instability is a separate assessment that evaluates the stability of the spine based on the presence of pathologic fractures, lytic bone destruction, deformity, and the presence of movement-related pain. Finally, the extent of systemic disease and medical comorbidities reflect the patient’s ability to tolerate an operation, radiation, or chemotherapy and the patient’s overall prognosis.
In general, the NOMS-based analysis is not intended as an algorithmic approach to patient care. Rather, this classification scheme offers a framework for discussion at multimodality treatment conferences (Fig. 109-1). Details regarding each of these four considerations are discussed later in the chapter.
Presentation
Back pain, the most common presenting symptom in patients with metastatic tumor to the bone or epidural space, often precedes the development of other neurologic symptoms by weeks or months. Back pain in a cancer patient is metastatic disease until otherwise proven. Two distinct types of back pain are encountered in patients with spine tumors: (1) biologic (i.e., tumor-related) pain and (2) mechanical pain.7 Biologic pain is the most common presenting symptom in metastatic cancer patients. This pain syndrome is predominantly nocturnal or early morning pain that generally improves with activity during the day. A variety of causes have been proposed, including periosteal irritation, stimulation of intraosseous nerves, and increased pressure or mass effect from tumor tissue in the bone.8 The likely genesis of biologic pain reflects the diurnal variation in endogenous steroid secretion from the adrenal gland. At night, steroid production is reduced, resulting in increased inflammatory pain caused by cytokines released by the tumor. During the day, steroid levels rise, eliminating this biologic pain. This inflammatory component of biologic pain generally responds to the administration of low-dose steroids (e.g., dexamethasone [Decadron] 4 mg three times daily). Definitive treatment of the underlying tumor with radiation or surgery often relieves this pain. Recurrence of biologic pain following treatment may be a harbinger of locally recurrent tumor.
Mechanical pain results from a structural abnormality of the spine, such as a lytic destruction of the vertebral body, resulting in instability (Fig. 109-2). Clinical symptoms and radiographic correlation are important for establishing the diagnosis of instability. As opposed to biologic pain, patients with mechanical instability present with pain that is worse with movement and that is referable to the level of spinal involvement. For example, although pathologic fractures of the atlantoaxial spine may present with severe pain in flexion-extension, they virtually always have a rotational component. In the subaxial cervical spine, mechanical pain is worse with flexion and extension. Counterintuitively, patients with thoracic or thoracolumbar compression fractures often have severe pain when lying flat as opposed to sitting or standing, presumably because of extension of an unstable kyphosis (Fig. 109-3). The most common symptom of instability in the lumbar spine is mechanical radiculopathy. This lumbar pain syndrome results from an axial load narrowing the neural foramen, thus causing compression of the exiting nerve root. Mechanical pain does not typically respond to steroids but may be relieved with narcotics or an external orthosis, pending definitive therapy. Patients with intractable mechanical pain are often considered strong candidates for surgery or percutaneous cement augmentation procedures (Fig. 109-4).
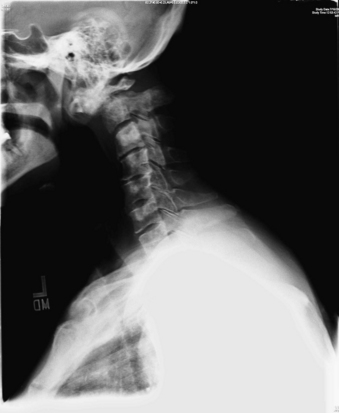
FIGURE 109-2 Mechanical instability. Lateral cervical spine plain radiograph showing fracture-subluxation of C2 due to metastatic renal cell carcinoma. (See also Figs. 109-6 and 109-15.)
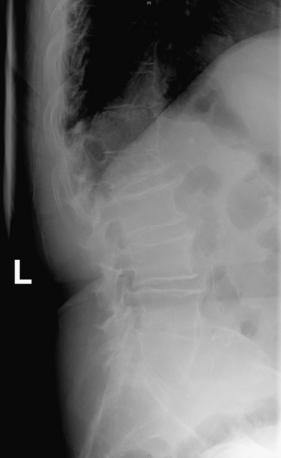
FIGURE 109-3 Pathologic compression fracture. Lateral thoracolumbar plain film showing L1 compression fracture due to multiple myeloma. Lesions at other levels, including T4 and T12, are not visualized on this film. (See also Figs. 109-4 and 109-5.)
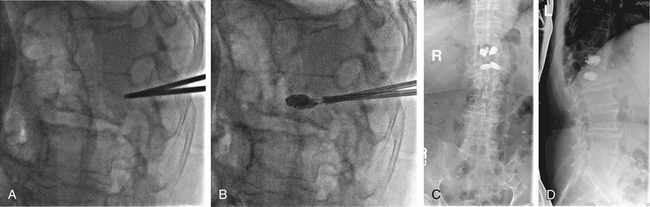
FIGURE 109-4 Kyphoplasty. Plain radiographs obtained during (A and B) and after (C and D) kyphoplasty procedure for the patient shown in Figure 109-3. A, Lateral plain radiograph showing percutaneous placement of bilateral Jamshidi needles into the pedicles of L1. B, Balloon inflation prior to instilling cement. Anteroposterior (C) and lateral plain radiographs (D) showing bilateral kyphoplasty of T12 and L1. (See also Figs 109–3 and 109-5.)
Neurologic signs and symptoms often begin with radiculopathy (nerve root symptoms) and are followed by the development of myelopathy (spinal cord compression). Radiculopathy in the cervical or lumbar spine causes pain or weakness in the classic dermatomal distributions. However, thoracic radiculopathy occurs as bandlike pain at a segmental level. Myelopathy often presents with a pain level secondary to compression of the spinothalamic tracts followed by motor loss related to corticospinal tract involvement. This may be related to the pattern of tumor arising from the vertebral body compressing the anterolateral spinal cord. Loss of proprioception from involvement of the dorsal columns is often a late finding in myelopathy and results in difficulty regaining normal ambulation. Autonomic dysfunction, principally of bowel and bladder, are typically very late findings in myelopathy. The exception is compression at the level of the conus medullaris or diffuse sacral replacement where autonomic dysfunction can be a very early finding. Neurogenic bowel and bladder symptoms are almost universally associated with perineal numbness and are most often painless. In the absence of sensory changes, one should seek other causes for urinary or bowel incontinence, such as narcotics, prostatic hypertrophy, or excessive use of laxatives.
Staging and Classification
The examination of spinal patients should include a pain assessment, quantitative neurologic score, general performance score, and quality-of-life assessment. Pain assessment can be most readily performed with a visual analogue scale. The score can be converted to reflect mild (0–4), moderate (5–6), and severe (7–10) pain.9 The two most commonly used neurologic scales include the Frankel grading system and the American Spinal Injury Association (ASIA) score10,11(Table 109-1). Both assess motor function, with a score of “E” being normal and “A” being complete paralysis. Performance status reflects ambulation, medical comorbidities, and extent of disease. A patient may have normal motor strength but be unable to ambulate due to loss of proprioception, severe mechanical pain, lower extremity fracture, poor nutritional status, or poor pulmonary function. We have used the Eastern Cooperative Oncology Group (ECOG) performance status as a functional assessment12 (Table 109-2). It is important to include both neurologic and performance status when reviewing outcomes in cancer patients.
Grade | Description |
---|---|
A | Complete: No motor or sensory function below the level |
B | Incomplete: Sensory but no motor function |
C | Incomplete: Some motor function is preserved, but a majority of the muscle groups below the lesion have a grade <3 |
D | Incomplete: Some motor function preserved, but a majority of the muscle groups below the lesion have a grade >3 |
E | Normal sensory and motor function |
ASIA, American Spinal Injury Association.
TABLE 109-2 ECOG Performance Status
Grade | Description |
---|---|
0 | Fully active, able to carry on all predisease performance without restriction |
1 | Restricted in physically strenuous activity but ambulatory and able to perform light work |
2 | Ambulatory and capable of all self-care but unable to perform work activities (bedridden <50% of the time) |
3 | Capable of only limited self-care (bedridden >50% of the time) |
4 | Completely disabled, not capable of any self-care (bedridden 100% of the time) |
ECOG, Eastern Cooperative Oncology Group.
Metastatic tumors to the spine are classified based on numerous features, including histology, location, and pattern of tumor. The most common spine metastases are listed in Table 109-3. These tumors are further classified into relatively radioresistant and radiosensitive groups (Table 109-4), which influences the decision to use radiation as first-line therapy. Tumors may further be divided by the level and extent of spinal element involvement (e.g., vertebral body, dorsal element, or circumferential) and degree of epidural compression (see later discussion). Thorough radiographic imaging is essential for treatment decisions.
TABLE 109-3 Review of Primary Cancers with Spinal Metastases seen at M.D. Anderson Cancer Center, 1984–1994
Primary Site | Percentage of All Spine Metastases (n = 11,884) |
---|---|
Breast | 30.2 |
Lung | 20.3 |
Blood | 10.2 |
Prostate | 9.6 |
Urinary tract | 4.0 |
Skin | 3.1 |
Unknown primary | 2.9 |
Colon | 1.6 |
Other | 18.1 |
Adapted from Gokaslan ZL, York JE, Walsh GL, et al: Transthoracic vertebrectomy for metastatic spinal tumors. J Neurosurg 89(4):599–609, 1998.
TABLE 109-4 Radiosensitivity of Various Metastatic Spine Tumors
Sensitivity | Tumor Histology |
---|---|
High | Lymphoma |
Myeloma | |
Intermediate | Breast |
Prostate | |
Low | Sarcoma |
Renal Cell | |
Lung | |
Colon |
Imaging
Plain radiographs are often ordered as the first test to evaluate the cancer in a patient with new-onset back pain; however, plain films are relatively poor screening tests for metastases (Fig. 109-5). Visualization of a radiolucent defect on plain radiographs typically requires at least 50% destruction of the vertebral body. Additionally, metastatic tumor often infiltrates the bone marrow of the vertebral body without destroying the cortical bone. Compression and burst fractures are readily identified. Plain radiographs can identify sagittal (kyphosis) and coronal (scoliosis) plane deformities in a weight-bearing state, whereas spinal deformities imaged in a supine position by MRI or CT may be reduced and, thus, remain undetected. Dynamic flexion and extension films may be used to detect instability, although in our experience they are rarely necessary and may put the patient at risk for progressive spinal cord injury. Following surgery, plain films are the best imaging modality for assessing spinal alignment and structural integrity of the instrumentation.
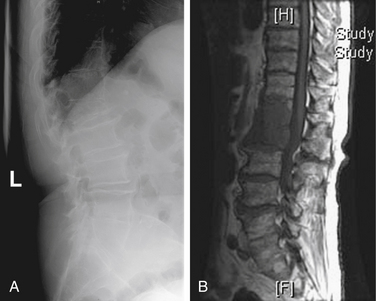
FIGURE 109-5 Comparison of plain film to MRI. A, Lateral plain radiograph of thoracolumbar spine showing a compression fracture at L1. This is the same film as Figure 109-3. B, MRI showing disease at T12 and L1. The T12 disease could not be appreciated on the plain film. (See also Figs. 109-3 and 109-4.)
Bone scans (using technetium-99m methylene diphosphonate [99mTc-MDP]) are more sensitive than plain radiographs for detecting spinal metastases13 (Fig. 109-6). The advantage of a bone scan is its ability to screen the entire skeleton with a single image. Patients with spinal tumors often have other bone involvement that may be causing symptoms or require intervention. For example, a patient with L2 vertebral body disease causing nerve root compression may have a concomitant, symptomatic tumor in the pelvis, hip, or femur. However, bone scans rely on an osteoblastic reaction or bone deposition to detect spinal metastases so that rapidly progressive, destructive tumors may not be detected.13,14 Bone scans are relatively insensitive for multiple myeloma and tumors confined to the bone marrow and have a low specificity for tumor.14 Fractures, degenerative disease, and benign disorders of the spine (Schmorl nodes, hemangioma) all may be positive. Additionally, paraspinal tumors that enter the epidural space through the neuroforamen can result in back pain and progressive neurologic symptoms that often are not detected on bone scan. In a review by Avrahami et al., 21 out of 40 patients (52%), with previously diagnosed tumor and symptoms referable to the spine had a negative CT and bone scan, but tumor was seen on MRI.15 Frank et al. reviewed a series of 95 patients, 28% of whom had a negative bone scan with MRI scan showing tumor and a discordance rate between the two imaging modalities of 31%.16
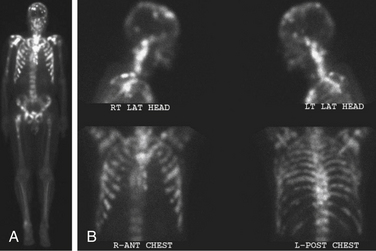
FIGURE 109-6 The bone scan is more sensitive than plain films for detecting metastatic disease. The images are total body (A) and head and chest views (B) showing numerous osseous metastases involving the skull, spine, ribs, pelvis, and long bones. Comparison of the lateral head and neck bone scan views to the plain radiograph of the same patient shown in Figure 109-2 demonstrates the increased sensitivity of bone scans for detecting osseous metastases. (See also Figs. 109-2 and 109-15.)
Until MRI became widely available, myelogram and CT were the best diagnostic modalities for assessing acute spinal cord compression. Risks associated with myelography, including acute neurologic decompensation in patients with high-grade blocks, have diminished its role.17,18 CT continues to be useful both for assessing the degree of bone destruction and for determining when bone rather than tumor is causing spinal cord compression. For patients who have had spinal reconstruction with placement of metallic instrumentation, including titanium, it may be difficult to obtain accurate images of the spinal canal with MRI, and CT myelogram may be helpful for ruling out recurrent epidural disease and spinal cord compression19–21 (Fig. 109-7). Myelography and postmyelogram CT images continue to be used for imaging for these patients. Also, CT myelograms are currently used for radiosurgery treatment planning to specifically identify the location of the spinal cord or cauda equina.22
MRI is the most sensitive and specific modality for imaging spinal metastases. Sagittal screening images of the entire spine reveal bone, epidural, and paraspinal tumor.23 The extent and degree of spinal cord compression can be readily appreciated, especially on T2-weighted images (Table 109-5). Hybrid scans of the brachial or lumbosacral plexus may reveal tumor in patients with extremity weakness that is not entirely related to spinal cord or root involvement. Leptomeningeal metastases and intradural metastases are often well visualized but require the use of contrast agents (gadolinium diethylene triamine pentaacetic acid [Gd-DPTA])24 (Fig. 109-8).
TABLE 109-5 Memorial Sloan-Kettering Cancer Center Epidural Spinal Cord Compression Grading Scale
Grade* | Description |
---|---|
0 | No subarachnoid space compression |
1 | Subarachnoid space partially obliterated without spinal cord compression |
2 | Subarachnoid space partially obliterated with spinal cord compression |
3 | Subarachnoid space completely obliterated with cord compression |
* Determined at level of worst compression.
Common imaging sequences used to evaluate spinal metastases are T1- and T2-weighted MRI.25 Tumor on a T1-weighted image is hypointense relative to the normal marrow signal (Fig. 109-9A) and typically enhances after administration of gadolinium (Fig. 109-9B). The ports from prior spinal radiation can be discerned on T1-weighted images as hyperintense signal change and may assist in making acute therapeutic decisions when radiation port films are not available. Tumor is hyperintense relative to marrow on standard T2-weighted imaging and produces a myelogram effect with cerebrospinal fluid appearing hyperintense (Fig. 109-9C). Unfortunately, using the recently developed timesaving fast-spin echo, T2 techniques may decrease tumor conspicuity. This decreased conspicuity can be compensated for using short-tau inversion recovery (STIR) techniques. STIR images show enhanced contrast between the lipid marrow (hypointense) and tumor (hyperintense)26–28 (Fig. 109-9D). They may be the most sensitive screening modality for tumor but give less anatomic detail than standard T1 or fast spin echo T2 images.29 Because of the high rate of multiple noncontiguous lesions, we suggest screening the entire spine with sagittal sequences followed by axial cuts through any areas of abnormality. At our institution, a screening assessment of the entire spine is obtained specifically to evaluate the T1- and T2-weighted STIR images. The degree of compression is based on the axial T2- and/or axial T1-weighted postcontrast images.
The conversion of spine tumor assessment from CT-myelogram to MRIs left a void in describing the degree of spinal cord compression. For instance, no correlate existed on MRI for a complete myelographic block. NOMS decision making is often made based on the neurologic assessment of the degree of spinal cord compression in combination with relative radioresistance of the tumor.6 A recent review by the Spine Oncology Study Group showed greater interrelater and intrarelater reliability using T2-weighted images than with T1-weighted pre- or postcontrast images in the assessment of spinal cord compression.29A The newly revised epidural spinal cord compression (ESCC) grading system assesses tumors on T2-weighted axial images and assigns a score from 0 to 3. Grade 0 indicates tumor within bone only without any involvement of the epidural space. Grade 1 is subarachnoid space impingement by tumor extending from the bone, but no compression or deformation of the spinal cord. For radiosurgery planning purposes, ESCC grade 1 was subdivided into 1a (epidural abutment), 1b (epidural impingement), or 1c (epidural impingement with spinal cord abutment). Grade 2 indicates spinal cord compression and deformation, but spinal fluid is still visualized at the level of compression. Grade 3 is spinal cord compression with obliteration of all cerebrospinal fluid space at the level of cord compression. Grade 3 is the magnetic resonance (MR) radiographic equivalent of a complete block on myelogram (Fig. 109-10;see also Table 109-5).
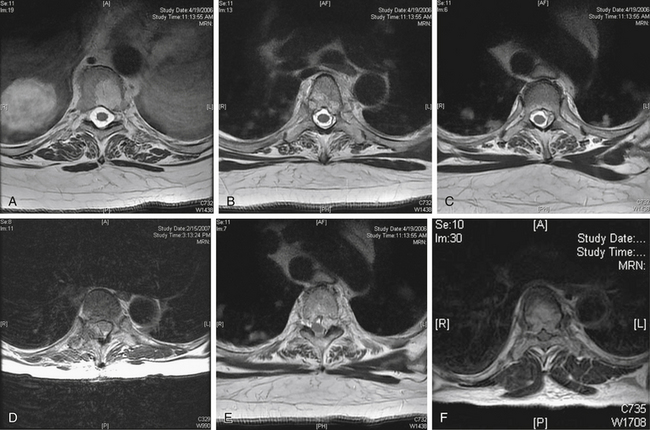
FIGURE 109-10 The epidural spinal cord compression grading scheme described in Table 109-5 is illustrated here with representative axial T2-weighted MRI. Grade 1 is epidural disease without spinal cord compression and is divided into three grades. A, Grade 0—bone involvement only. B, Grade 1a—abutment of the thecal sac. C, Grade 1b—indentation of the thecal sac. D, Grade 1c—abutment of the spinal cord. E, Spinal cord compression or deformation, but cerebrospinal fluid (CSF) is still found at this level. F, Spinal cord compression with obliteration of all CSF space at this level.
Although MRI is an excellent screening tool for metastatic tumor spread to bone, differentiating tumor from osteomyelitis, osteoporotic compression fractures, and previously treated tumor may be difficult. The T1- and T2-signal characteristics are similar in all of these conditions. Osteomyelitis is more likely to cause changes in the end plate and disc space, whereas tumor rarely, if ever, involves the disc space. Based on these imaging characteristics, osteomyelitis can be differentiated from tumor with 97% accuracy.30 Unfortunately, patients with tumor may secondarily become infected, rendering the imaging patterns unreliable in these situations.31
Osteoporotic compression fractures are extremely common in the cancer population and have been differentiated from pathologic fractures with 94% accuracy based on T1-weighted imaging characteristics.32 Osteoporotic fractures are more commonly thoracic, lack signal change, have bandlike abnormality and do not involve the pedicle, or have contour abnormality. Pathologic fractures show homogeneously decreased signal and have convex vertebral contours. Pathologic fractures may involve the pedicles and are more commonly located in the lumbar spine.
Oncologists often rely on imaging changes to determine the efficacy of treatment; however, response to RT or chemotherapy is difficult to assess in bone tumors because of the lack of signal change on MRI. On T1-weighted images, both treated and viable tumors appear hypointense relative to normal marrow signal. In a study of breast cancer patients, only 3% had a reduction in the volume or number of vertebral bodies involved on imaging, and there was no correlation between changes in signal intensity and clinical response to therapy.33 In a palliative care situation, clinical response to therapy (resolution of tumor-related pain) may suffice despite the absence of radiographic change. Therapeutic decisions for some metastatic tumors (e.g., Ewing sarcoma, neuroblastoma, and seminoma) rely on differentiating viable from necrotic tumor. Traditional MRI sequences do not change significantly after treatment. Current investigations are examining the use of MR perfusion to differentiate viable from necrotic tumor.
Recent work has explored the use of 2-[18F] fluoro-2-deoxy-d-glucose (FDG-PET) for differentiating osteoporotic or traumatic fractures from pathologic compression fractures and to determine viability of previously treated bone tumors.34–36 Additionally, on T1-weighted images, bone edema may appear hypointense similar to tumor signal and FDG-PET may be useful in directing the biopsy to a specific hypermetabolic site in the vertebral body, thereby increasing the chance of successfully making a diagnosis. Laufer et al. reviewed 82 patients with hematologic and solid tumor malignancies. All patients underwent biopsy within 6 weeks of their PET scan. The mean standard uptake values (SUVs) were 7.1 for malignant tumors compared with 2.1 for benign lesions (p < .02). A 100% concordance was identified with an SUV cutoff of 2 in solid tumor malignancies with lytic or mixed lytic sclerotic bone involvement. Sclerotic bone lesions often have low SUVs secondary to the paucity of tumor cell, and PET is less predictive in differentiating osteoblastic tumors from benign pathology.37 Similar work relating SUVs to the presence of tumor found a threshold cutoff of 2.5 that predicted tumor.38 Improved diagnostic accuracy can be achieved by combining PET with CT since 18(F)-FDG PET/CT has a greater specificity for detecting spine metastases than either 18(F)-FDG PET or CT alone39 (Fig. 109-11). Other radionuclide scans may be helpful for screening specific tumor types, including iodine-109 scans for papillary thyroid cancer, meta-iodobenzylguanidine (MIBG) scans for neuroblastoma, and somatostatin scans for neuroendocrine tumors.
Metabolic and Physiologic Issues
Cancer patients are prone to numerous metabolic and physiologic abnormalities either as the result of their disease process or due to side effects of previous treatments. Therefore, assessment for many of these abnormalities must be performed prior to considering treatment. Hypercalcemia occurs in approximately 10% to 20% of all cancer patients, with lung and breast tumors being the most common primaries.40 The pathophysiologic abnormalities that lead to this condition are believed to be secondary to the multifactorial effects of increased bone turnover and increased calcium reabsorption in the proximal renal tubules. However, immobilization and dehydration have also been shown to be contributing factors, especially in patients with end-stage disease.40 These homeostatic abnormalities are now thought to be the result of secretion of a parathyroid-related protein as well as secretion of cytokines such as transforming growth factor-beta (TGF-β), interleukin-1 (IL-1), and tumor necrosis factor (TNF).41 Hypercalcemia is commonly treated with IV fluid rehydration and bisphosphonate administration; left untreated, it can result in cardiac or kidney dysfunction, and even death in extreme cases.42
Coagulation abnormalities also occur commonly in this patient population. This can be attributed both to their cancer diagnosis as well as to an association with neurosurgical procedures.43 Coagulopathies can result from metastatic tumor spread to the liver or more commonly from the toxic side effects of chemotherapeutic agents. In addition, frequent blood transfusions that some of these patients receive may result in antiplatelet antibodies, which may be resistant to replacement transfusions. Thrombocytopenia may result from diffuse bone marrow replacement or wide field irradiation, but commonly results from chemotherapy or common medications, such as heparin. A blood panel for heparin-induced thrombocytopenia (HIT) is obtained for patients taking heparin or heparin analogues who are thrombocytopenic, and the medication should be stopped. Treatment for coagulopathies depends primarily on the underlying cause.44
Cancer patients are also at an increased risk for developing deep venous thrombosis (DVT).45 The etiology is thought to be multifactorial and not simply a result of immobility. Many solid tumors release cytokines and other tissue factors that have procoagulant effects. We have found perioperative prophylaxis with pneumatic compression boots and subcutaneous dalteparin (Fragmin, 5000 units SQ bid) to be helpful in decreasing the rate of postoperative DVT, but not foolproof. Patients immobilized with paresis or pain, routinely undergo Doppler ultrasounds prior to surgery. Any DVT identified preoperatively is managed with inferior vena cava filter placement. Leon et al. showed a benefit to prophylactic filter placement for patients undergoing major spine surgery.46 Risk factors for pulmonary embolism included malignancy, prior history of thromboembolism, bedridden more than 2 weeks prior to surgery, staged or multilevel procedures, and prolonged surgery (>8 hours). Consideration for placement of removable vena cava filters should be given to any tumor patient with a significant paresis or plegia who will need to intermittently discontinue full-dose anticoagulation. Postoperatively, DVTs are treated with either inferior vena cava filters or anticoagulation.
Estimating Tumor Burden in Other Regions
At MSKCC, tumor staging is usually performed in conjunction with the primary oncologists, who have a better appreciation of the patient’s disease in terms of overall aggressiveness and pace of progression.6 This workup is typically performed with radiographic studies, including CT with and without contrast of the chest, abdomen, and pelvis. PET-CT is more commonly used as a screening method. Serum markers can also be used to screen for the presence or progression of tumors, such as prostate-specific antigen (PSA, prostate carcinoma), CA-125 (breast carcinoma), and carcinoembryonic antigen (CEA, colon carcinoma). These markers are remarkably sensitive and may be an early indicator of tumor recurrence. In hormone-refractory prostate carcinoma with spine involvement, the PSA often serves as a marker for tumor recurrence well in advance of scheduled surveillance MRI.
Pain Control
The adequate control of cancer-related and postoperative pain can be very challenging in this population. A significant number of these patients may have chronic pain syndromes and require large doses of narcotics, typically in the form of delayed-release oral or transdermal preparations.47,48 This makes postoperative pain control difficult because of tolerance to these agents. At our institution, all patients receive narcotics via patient-controlled analgesia (PCA: morphine, fentanyl, or hydromorphone [Dilaudid]) postoperatively for several days with frequent dosing modifications until pain is adequately controlled. Following this, they are switched to equianalgesic doses of oral or transdermal medications. It is often helpful to obtain the input from pain management specialists for those patients with significant preoperative symptoms or high dose requirements.
Treatment
Antitumor Chemotherapy
Antitumoral chemotherapy currently plays a relatively limited role in the treatment of spinal metastases. However, antitumor chemotherapy has an important role in the treatment of chemosensitive tumors, such as neuroblastoma, Ewing sarcoma (primitive neuroectodermal tumor [PNET]), osteogenic sarcoma, germ cell tumors, and lymphoma.49 At MSKCC, chemotherapy is often considered the primary treatment for patients with these tumors even in the presence of epidural compression (Fig. 109-12). Chemotherapy and hormones may be useful in the treatment of prostate and breast carcinoma, but local therapy with radiation and/or surgery is commonly used for symptomatic metastases.
Steroids
Steroids are typically used for two indications in patients with metastatic spine tumors. The first is for the control of biologic pain. Typically, the administration of low doses will have profound effects on the level of pain in patients with this type of symptoms. The second indication is for the control of vasogenic edema to help reduce or stabilize neurologic symptoms in preparation for definitive radiation or surgical treatment. The optimal dose used to treat patients with acute spinal cord compression is controversial.50–54 Doses range from moderate (16 mg/day in divided doses) to high (96 mg/day in divided doses) with or without a 10- to 100-mg load. It is unclear whether higher-dose steroids improve neurologic outcomes compared with moderate-dose steroids, but significantly more complications result from the higher doses.55 In a case-control series from a single institution, total side effects seen in the moderate- and high-dose steroids were 8% and 29%, respectively.55 Complications from steroids include hyperglycemia, gastrointestinal hemorrhage, intestinal perforation, and avascular necrosis of the hip. The use of steroids during radiation is also controversial, but these drugs are commonly used in symptomatic patients with high-grade spinal cord compression to prevent spinal cord swelling and neurologic decompensation. In patients fully ambulatory without spinal cord compression, steroids may not be needed. In a case series of 20 patients treated with conventional radiation, all maintained ambulation without toxicity.56
In a patient with an undiagnosed spinal mass, steroids should be withheld prior to biopsy because of the oncolytic effect of these drugs on certain tumors such as lymphoma and thymoma.57 The exception may be patients with high-grade spinal cord compression and myelopathy in whom a delay in steroid administration may affect neurologic outcome.
Bisphosphonates
Bone destruction that occurs secondary to bone metastases results from a combination of osteoclast activation and osteoblast inhibition. Tumor cells secrete substances such as parathyroid hormone-related protein, TGF-β, cytokines, and interleukins that affect the balance between osteoblastic bone formation and osteoclastic bone turnover.58–60 These substances lead to osteoclast activation and thus increased bone turnover. Therefore, these cells are thought to play a central role in the development of bone metastasis, and inhibition of these cells may limit the progression of such metastases.61–64 In multiple myeloma, the molecular mechanisms of osteoclast activation have been extensively studied. Multiple myeloma cells induce stromal cells to secrete cytokines, such as IL-6, TNF-β, and insulin-like growth factor. These cytokines up-regulate the receptor activator for nuclear factor κ B ligand (RANKL) expression, inducing the creation and activation of osteoclasts.65–68 Osteoprotegerin normally inhibits RANK-RANKL signaling, but the level of osteoprotegerin is markedly decreased in multiple myeloma patients. This inhibition leads to increased osteoclast activation and lytic bone destruction.69 In addition to osteoclast activation, multiple myeloma results in osteoblast inhibition. This inhibition prevents osteogenesis, adding significantly to the resultant lytic destruction of bone. Recent evidence suggests that gene expression of the secreted glycoprotein dickkopf 1 (DKK) inhibits the Wnt signaling pathway, which is essential for the growth and differentiation of osteoblasts.70,71 Increased expression of DKK1 has been associated with lytic bone destruction in multiple myeloma patients.72
Other work has further characterized the extracellular cues, intracellular signaling cascade, and gene expression profiles that mediate osteolytic metastasis in various types of cancer, primarily breast cancer.73,74 This work helps to explain the tendency of certain cancers to metastasize to bone, particularly the spine.
Bisphosphonates are a class of drugs recently studied as a potential treatment for cancer patients. Bisphosphonates are synthetic derivatives of pyrophosphates that reduce skeletal complications in cancer patients.75–77 These agents inhibit osteoclast activity and, thus, reduce or inhibit further bone resorption; however, bisphosphonates do not promote the deposition of new bone.78 This occurs as a result of down-regulation and apoptosis of osteoclasts, which decreases bone turnover.58
Oral bisphosphonates are not generally effective. The IV preparations used to prevent skeletal events in cancer patients include zoledronic acid (4 mg) and pamidronate (90 mg) administered every 3 to 4 weeks. Although zoledronic acid is more expensive, the injection can be given in 15 minutes compared with the 2 hours required for pamidronate. Clodronate and ibandronate are two additional bisphosphonates that have been explored in European studies for multiple myeloma patients, but are not currently approved in the United States. Multiple randomized clinical studies have shown that the use of these medications can significantly decrease the rate of skeletal-related events, such as pathologic fractures, compression fractures, hypercalcemia, and need for radiation to bone metastases in patients with breast cancer and multiple myeloma.77,79–93 In addition, some studies in patients with breast cancer have shown that use of these compounds prior to development of bony metastasis may decrease the rate of future bone metastasis and skeletal-related complications when compared to the group receiving the placebo.94–97 Other work does not show benefit in breast cancer patients in terms of bone metastases or fractures.98 Berenson et al. reported the results of the Myeloma Aredia Study Group, in which 392 patients were randomized to receive placebo or pamidronate every 4 weeks for 21 cycles.81 Skeletal-related events (SREs) were defined as pathologic fractures, the need for radiation therapy or surgery, or progression to spinal cord compression. At 21 months’ follow-up, the mean number of annual SREs was 2.1 in the placebo group compared with 1.1 in the pamidronate group (P = .008). The median time to first SRE was 10 months in the placebo group and 21 months in the pamidronate group (P < .001).
These findings have been confirmed by laboratory experiments, which have shown that these agents decrease the adherence of tumor cells to bone structures.99–101 However, studies evaluating the efficacy of these compounds for prostate cancer have shown mixed results.102–108 In addition to protecting against skeletal complications, these medications have also been found to be useful in controlling bone pain.109–115 Despite their ability to minimize bone metastasis and control tumor-related pain, none of these compounds have ever been shown to improve survival.77,90,92,96
The American Society of Clinical Oncology has published practice guidelines for the administration of bisphosphonates in patients with multiple myeloma.116 Zoledronic acid or pamidronate is recommended for patients with lytic bone destruction or compression fracture from osteopenia. Additionally, for patients with diffuse osteopenia based on plain radiographs or a bone mineral density test in the absence of lytic disease or compression fractures, the recommendation is to start therapy. Patients on bisphosphonates are monitored for renal failure with monthly serum creatinine levels prior to administration of therapy. Patients are also monitored every 3 to 6 months for proteinuria. Other routine blood tests include measuring calcium, magnesium, and hemoglobin. Adverse side effects from bisphosphonates include transient myalgias, arthralgias, and flulike symptoms.77–91 Side effects from bisphosphonates are uncommon, but may be debilitating. Gastrointestinal side effects predominate, especially esophagitis and diarrhea; however, administration with a full glass of water may limit many of these complaints. Other problems such as fever, fatigue, or headache have also been described.91,92 Recently, osteonecrosis of the jaw (ONJ) has been described in conjunction with bisphosphonates. Zervas et al.117 reported a 9.5-fold increase in this complication using zoledronic acid compared with pamidronate. To date, the role of bisphosphonates remains unclear in the genesis of ONJ as most patients are additionally receiving chemotherapy, radiation, and steroids. A number of patients with ONJ have poor dentition or recent dental work. Patients may benefit from pretreatment comprehensive dental care.
Treatment Decisions
Although chemotherapies and hormonal therapies play a role in the treatment of metastatic spine tumors, radiation therapy or surgery is considered the principle initial treatment. Technologic advances in both radiation and surgery have dramatically changed decision making over the past 10 years. From a surgical perspective, ventral transcavitary and dorsolateral approaches as well as segmental ventral and dorsal instrumentation improved outcomes for tumor patients.118–140 Percutaneous cement augmentation provides significant pain relief in the presence of pathologic fracture. Additionally, the evolution of spine stereotactic radiosurgery (SSRS) provided unprecedented local tumor control for radioresistant tumors. The NOMS framework integrates these technologic advances into the decision making for metastatic spine tumors.
The neurologic and oncologic decisions regarding surgery versus radiation are typically made in combination. The essential considerations from a neurologic perspective are the degree of spinal cord compression with or without myelopathy and, from an oncologic perspective, the radiosensitivity of the tumor. Historically, variable radiosensitivity based on tumor histology was recognized but was not part of the decision-making process, because the results from surgery were so poor. In the 1970s, RT replaced laminectomy without instrumentation as first-line therapy for patients with spinal metastasis with epidural compression. This was based on several comparative studies that showed no difference in outcome between patients undergoing external photon beam RT versus those receiving laminectomy without dorsal segmental fixation (often in combination with RT).17,141–157 In these older series, approximately 75% of patients were nonambulatory at the time of presentation.158 Patients undergoing RT alone showed a 79% rate of maintaining ambulation, and paraparetic patients showed a 42% rate of return to ambulation. Both ambulatory and nonambulatory patients had a 21% risk of neurologic decompensation during RT. Patients undergoing laminectomy alone, or those receiving postoperative RT, had a 48% to 67% rate of maintaining ambulation, and paraparetic patients had a 33% rate of recovering ambulatory status. A range of 17% to 52% of patients showed neurologic decompensation following surgery with or without the addition of postoperative RT. The posttreatment morbidity was significantly less from RT than from surgery.
Radiosensitive Tumors
As advanced surgical approaches provided the ability to decompress and stabilize the spine with acceptable morbidity, the differential in radiation responsiveness became essential for decision making. Patients with radiosensitive tumors can be treated successfully with conventional external beam radiation (cEBRT), often delivered as 30-Gy doses in 10 fractions, regardless of the degree of epidural spinal cord compression. These tumors often respond dramatically, so that even with high-grade ESCC and myelopathy, radiation will decompress the spinal cord. Maranzano and Latini have the best functional results after radiation therapy, with 76% of patients ambulating after radiation and 51% of nonambulatory patients regaining ambulation.159 The study included 209 evaluable cases that underwent 30-Gy RT using two different fractionated dosing schedules. Patients with favorable tumor histology (lymphoma, myeloma, and seminoma) received 10 fractions in 2 weeks. Patients with less favorable histology received 5 Gy daily for 3 days followed by five daily doses of 3 Gy after a 4-day rest. The results depended greatly on tumor histology, with liver, bladder, and RCC showing significantly less recovery than did breast and hematologic malignancies (i.e., lymphoma and myeloma). For example, breast carcinoma demonstrated an 80% response rate compared with hepatocellular carcinoma with a 20% response rate.
Radioresistant Tumors
As noted, traditionally radioresistant tumors respond poorly to cEBRT, but SSRS has changed the concept of radioresponsiveness even for tumors such as RCC, lung adenocarcinoma, colon carcinoma, and melanoma. As initial therapy, high-dose single-fraction radiation can be effectively delivered to tumors in the absence of high-grade ESCC (ESCC 0 to 1b). Yamada et al. reported outcomes in 103 solid-tumor metastases treated in a dose escalation from 18 to 24 Gy in a single fraction. All tumors were resistant to cEBRT with the exception of six breast carcinomas. The local control was 90%, with failures occurring at a median of 9 months. Minimal toxicity was noted with grade 1 and 2 skin or esophageal tumors. No evidence of myelopathy or functional radiculopathy was encountered. Dose constraints were maintained at less than 14 Gy to a single voxel (single point in a three-dimentional image set) on the spinal cord and less than 16 Gy to the cauda equina.160 In a recent update of 248 treated lesions in 229 patients with a median follow-up of 17 months (range, 6–58 months), patients who received 24 Gy had a radiographic tumor control of 95%, whereas those who received 18 to 23 Gy demonstrated a local control of only 80% (P = .048) (see Fig. 109-1). Tumor local control was not affected by traditional factors that influence outcome for cEBRT, such as tumor histology or size. Other investigators have found that even traditionally radioresistant tumors, such as melanoma and RCC, respond as well as radiosensitive histologies such as breast cancer when SSRS was given.161–163 However, others have found that histology does make a difference in tumor control when SSRS is utilized, with RCC and melanoma doing significantly worse.164
Surgery
Surgery is currently indicated as initial therapy in patients with high-grade spinal cord compression from radioresistant tumors with myelopathy and spinal instability (Box 109-1). From a practical standpoint, symptomatic spinal cord compression requires expeditious decompression of the epidural space. As noted, most solid tumors are relatively radioresistant to cEBRT, with response rates of 20%, thus making cEBRT unreliable in terms of spinal cord and neurologic salvage. SSRS can treat radioresistant tumors but requires a margin on the spinal cord to deliver a cytotoxic dose. In all of the surgical spine literature, only 12 publications described the outcome of surgical decompression and stabilization of spinal cord or root compression secondary to metastatic tumors.1,130–131,165–174 Patchell et al. provide the only prospective randomized trial with evidence of the efficacy of surgical decompression for high-grade spinal cord compression.1 The remaining four prospective cohort studies and seven retrospective chart reviews fall under very low quality evidence. Generally, the results of surgical decompression in patients with spinal cord compression are superior to those for a radiation series, and this is particularly applicable to radioresistant tumors.175 In the surgical series, 74% to 100% of patients were ambulatory after decompression, with 57% to 82% of nonambulatory patients recovering the ability to ambulate.118,120,126–129,131–139
Patchell et al. conducted a randomized, multi-institutional trial comparing external beam radiation (30 Gy in 10 fractions) to decompressive surgery and instrumentation followed by external beam radiation therapy in symptomatic patients with spinal cord compression.1 Spinal cord compression was defined as displacement of the spinal cord, and neurologic symptoms ranged from pain only to myelopathy resulting in loss of ambulation. Hematologic malignancies and markedly radiosensitive tumors, such as germ cell tumors, were excluded, as were patients with other neurologic comorbidities (e.g., brain metastases). Other exclusion criteria included discontiguous epidural disease, an expected survival time of less than 3 months, and the inability to tolerate the proposed treatment. The protocol defined instability according to the Cybulski176 criteria or bone in the spinal canal, but instability was not an indication for exclusion.
Improved surgical outcomes have been seen using techniques that provide exposure for more radical tumor resection than laminectomy. Reconstruction following these aggressive approaches is now possible using rigid dorsal segmental fixation and ventral instrumentation.177 These include ventral transcavitary and dorsolateral transpedicular approaches. The decision to use a particular surgical approach depends on the location of the bone, epidural, and paraspinal tumor, the type of reconstruction required, patient comorbidities, the extent of disease, and the surgeon’s experience. Regardless of the approach, the goal is decompression of the spinal canal with the maximal possible cytoreduction of the tumor.
As with RT, factors that affect outcome include preoperative neurologic and functional status and favorable tumor histology. Kelkamp and Samii reviewed 101 patients operated on for metastatic spinal tumor prior to receiving adjuvant therapy (RT or chemotherapy) for their spinal tumor.126 The operations included dorsolateral (79%), ventral transcavitary (12%), and ventral and dorsal approaches (9%). Ninety-six percent of patients who were ambulating preoperatively maintained ambulation for at least 3 months. Only 22% of nonambulatory patients regained ambulation over the same duration. This maintenance or recovery of function is similar to the RT data presented by Maranzano and Latini.159 Additionally, 89% of patients maintained continence for 3 months, but only 31% regained autonomic function. Patients with favorable tumor histology (e.g., breast, kidney, thyroid, and prostate) had significantly better neurologic outcome and survival than those with unfavorable histologies (lung, gastrointestinal tract, and unknown primary). As has been seen in other studies, local recurrence rates are significant.131 In this study, 58% recurred after 6 months, 69% at 1 year, and 96% after 4 years. Factors predictive of low recurrence rates included preoperative ambulatory status, favorable tumor histology, cervical level, low number of affected vertebral bodies, complete resection, and elective surgery.
A review of multiple series shows complication rates from surgery ranging from 10% to 52%.118,120–137,178 Complications include DVT, myocardial infarction, and pneumonia. Surgical complications include failed fixation requiring revision and postoperative hematoma. Wound dehiscence and infection are complications predominantly seen with dorsolateral approaches in up to 15% of cases. We have found that trapezius or latissimus dorsi rotation flaps provide excellent soft tissue coverage and markedly reduce the morbidity from this complication.179 Mortality rates are as high as 13%178 and are frequently related to the patient’s medical or oncologic condition.
The second indication for surgery is mechanical instability because radiation treatment has no ability to stabilize an unstable spine. Definitions of instability are still being developed for pathologic fractures. In general, patients with unstable fractures demonstrate severe movement-related pain that is characteristic of specific spinal levels in correlation with radiographic findings. Burst or compression fractures of the thoracic and lumbar spine often present with axial load pain but do not require instrumented fixation. The pain from these fractures frequently improves after a few days of bedrest and limited activity. Alternatively, the fractures may respond to percutaneous cement augmentation, using vertebroplasty or kyphoplasty.180–182 Definitive therapy with radiation provides local tumor control without the need for surgery.
Patients requiring instrumented fusion of the atlantoaxial region have pain with flexion, extension, and rotation. However, patients presenting with C1-2 pain and normal spinal alignment or minimal subluxation respond well to external beam RT. These patients can be placed in a hard collar during treatment and for 6 weeks following the completion of RT. Patients with fracture subluxations greater than 5 mm or greater than 3.5 mm subluxation and 11 degrees of angulation between C1 and C2 with movement-related neck pain require instrumented spine fixation.183,184
Systemic Disease Assessment
The systemic disease assessment reflects what the patient can tolerate physiologically from the standpoint of the systemic disease and medical comorbidities. The systemic disease assessment is typically aimed at survival prediction and whether the patient is likely to withstand surgery with an acceptable risk of medical complications. Several authors have developed scoring systems that attempt to predict survival and potential benefit in patients with metastatic disease to the spine.185–187 However, these scores are based on historical data without the benefit of current adjuvant therapies, and there remains a high variability in the outcome of individual patients. As such, a poor “score” on these instruments should be interpreted with caution and should not preclude surgical intervention in otherwise satisfactory candidates.
Treatment Decision Making
At MSKCC, radiation therapy is the first-line treatment for most patients who present with metastatic spine tumors. Surgery is reserved for a variety of indications based on the four categories described earlier as NOMS: neurologic, oncologic, biomechanical, and systemic disease (see Fig. 109-1).6 Patients with radioresistant tumors (e.g., sarcoma, RCC), spinal instability, and/or a pathologic fracture with bone in the spinal canal are considered for surgery prior to radiation therapy.7 Patients with significant mechanical or radicular pain complaints may only marginally improve with radiation therapy alone and may benefit from surgical decompression and stabilization. Another frequently reported indication for surgery is lack of a diagnosis, but this can often be accomplished with CT-guided needle biopsy or thoracoscopic biopsy.
After RT that has reached spinal cord tolerance, patients are considered for surgery based on progression of neurologic symptoms, radiographic progression of tumor, and spinal instability. Patients with residual radiographic tumor following radiation or chemotherapy may be considered for curative surgery (e.g., osteogenic sarcoma, Ewing sarcoma, germ cell tumor). Contraindications to surgery include a limited life expectancy, significant medical comorbidities, and extensive disease.3 Additionally, paraplegic patients are rarely operated on because of the significantly low rate of recovery, particularly after 24 hours.
Case Presentations
Mechanical Back Pain Requiring Dorsolateral Decompression
Case 1
A 62-year-old male is newly diagnosed with RCC. He presented with progressive neck and low back pain. By the time he was admitted to the hospital, he had severe mechanical back pain centered at his upper thoracic spine that was worse with movement and when he was supine but better when he was sitting up. MRI showed numerous osseous metastases to his spine (Fig. 109-13). These lesions were associated with minimal spinal canal involvement except for the C7 and T3-4 lesions. The patient underwent embolization of these lesions followed by a dorsolateral decompression and instrumentation. His pain was significantly reduced (mechanical), and he underwent radiation to treat the remaining lesions.
Biologic Pain Treated with Image-Guided Radiation Therapy
Case 2
A 63-year-old male presented with low back pain that was worse at night and improved during the day. He had been treated previously with surgery for a thoracic spine lesion and had recently been removed from a clinical trial due to worsening symptoms and generalized poor health. He underwent IGRT to adjacent lesions in the lower thoracic and upper lumbar spine and ultimately achieved significant reduction in pain (oncologic) and improvement in his quality of life (Fig. 109-14).
Mechanical Instability
Case 3
A 72-year-old male presented with a 4-week history of worsening neck pain. He had a known history of widely metastatic RCC. The pain worsened with any head movement. Workup revealed metastatic disease causing a fracture-subluxation at C2. The patient underwent occipitocervical fusion followed by IGRT. Two months after treatment, his tumor showed no radiographic evidence of progression, and his pain was significantly reduced (Fig. 109-15; see also Figs. 109-2 and 109-6).
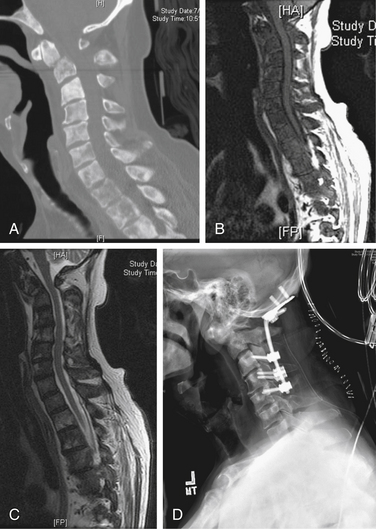
FIGURE 109-15 Case 3. A patient with renal cell carcinoma (same patient as Figs. 109-2 and 109-6) presented with neck pain. A, Sagittal reconstruction of a cervical spine CT shows the fracture-dislocation at C2, which can also be visualized on T1-weighted MRI (B) and T2-weighted MRI (C). D, Dorsal instrumentation from the occiput to C5 shown in this lateral plain radiograph of the cervical spine provided stabilization and improved his pain.
Surgical Decision Making
Once the decision to operate has been made, several other issues must be still considered. The first is whether preoperative embolization is indicated. At MSKCC, embolization is performed for vascular tumors (e.g., RCC, papillary thyroid carcinoma, leiomyosarcoma) 1 or 2 days prior to surgery as this has been shown to dramatically reduce intraoperative blood loss (Fig. 109-16).188–190 In our series of 140 patients operated on via a dorsolateral approach with circumferential instrumentation, embolization successfully reduced operative blood loss for vascular tumors (i.e., renal, thyroid, angiosarcoma) to the same level as those not requiring embolization (1900 mL and 1620 mL, respectively).191
The next issue is whether instrumentation and bone grafting are necessary. Instrumentation following surgical decompression is indicated for a majority of patients undergoing surgical intervention for metastatic spine disease.192 This is based on the fact that many of these patients have preexisting mechanical instability as a result of tumor-related bone destruction, iatrogenic instability as a result of intraoperative bone removal, or are at risk for delayed instability secondary to tumor progression. The addition of instrumentation prevents the delayed development of mechanical back pain and often only adds 30 to 60 minutes to the case. Many of these patients require longer fusion segments as opposed to patients with degenerative disease because of tumor involvement in surrounding bone, the risk of adjacent segment progression, and the high probability of osteoporosis. Autogenic bone grafting is routinely performed in cases of dorsal decompression and instrumentation, but the expectation of arthrodesis is limited due to the limited life expectancy, as well as the effects of radiation and chemotherapy.193 The routine use of external orthoses is not supported because these can compromise pulmonary function and wound healing in patients with minimal reserves.
Another issue concerns whether the type of tumor resection should be either en bloc or intralesional. En bloc spondylectomy as described by Tomita et al. is a technique based on sound oncologic principles.194 The intent of this surgery is en bloc resection of a tumor with negative histologic margins. This surgery is feasible as a one- or two-stage procedure, but is technically quite demanding and has higher morbidity rates.195–198 However, results are encouraging, both in terms of functional outcome and local control.
In most cases, though, surgery for spinal metastases is palliative, and in few cases will attempts at negative histologic margins influence clinical outcome.199 Based on anatomic considerations, the majority of patients with metastatic tumors are not candidates for this type of surgery because of extensive epidural disease, multilevel vertebral body involvement, and large paraspinal masses. The evolution of SSRS has largely obviated the need to consider en bloc resection even in patients with solitary metastases and a long life expectancy. SSRS provides superb durable control that is independent of histology.
Thoracoscopic vertebral body resection for tumor has been reported in small series.200,201 This relatively noninvasive approach has proven useful for removing ventral thoracic discs and ventral releases for scoliosis corrections. The potential use for most tumors requiring resection is probably limited. We currently reserve this technique for when CT-guided biopsies fail.
Bilsky M., Smith M. Surgical approach to epidural spinal cord compression. Hematol Oncol Clin North Am. 2006;20(6):1307-1317.
Gerszten P.C., Burton S.A., Ozhasoglu C., et al. Stereotactic radiosurgery for spinal metastases from renal cell carcinoma. J Neurosurg Spine. 2005;3(4):288-295.
Maranzano E., Latini P. Effectiveness of radiation therapy without surgery in metastatic spinal cord compression: final results from a prospective trial. Int J Radiat Oncol Biol Phys. 1995;32(4):959-967.
Patchell R.A., Tibbs P.A., Regine W.F., et al. Direct decompressive surgical resection in the treatment of spinal cord compression caused by metastatic cancer: a randomised trial. Lancet. 2005;366(9486):643-648.
Wang J.C., Boland P., Mitra N., et al. Single-stage posterolateral transpedicular approach for resection of epidural metastatic spine tumors involving the vertebral body with circumferential reconstruction: results in 140 patients. Invited submission from the Joint Section Meeting on Disorders of the Spine and Peripheral Nerves, March 2004. J Neurosurg Spine. 2004;1(3):287-298.
Yamada Y., Bilsky M.H., Lovelock D.M., et al. High-dose, single-fraction image-guided intensity-modulated radiotherapy for metastatic spinal lesions. Int J Radiat Oncol Biol Phys. 2008;71(2):484-490.
1. Patchell R.A., Tibbs P.A., Regine W.F., et al. Direct decompressive surgical resection in the treatment of spinal cord compression caused by metastatic cancer: a randomised trial. Lancet. 2005;366(9486):643-648.
2. Leithner A., Radl R., Gruber G., et al. Predictive value of seven preoperative prognostic scoring systems for spinal metastases. Eur Spine J. 2008;17(11):1488-1495.
3. Tokuhashi Y., Ajiro Y., Umezawa N. Outcome of treatment for spinal metastases using scoring system for preoperative evaluation of prognosis. Spine (Phila Pa 1976). 2009;34(1):69-73.
4. Tokuhashi Y., Matsuzaki H., Oda H., et al. A revised scoring system for preoperative evaluation of metastatic spine tumor prognosis. Spine (Phila Pa 1976). 2005;30(19):2186-2191.
5. Tokuhashi Y., Matsuzaki H., Toriyama S., et al. Scoring system for the preoperative evaluation of metastatic spine tumor prognosis. Spine (Phila Pa 1976). 1990;15(11):1110-1113.
6. Bilsky M., Smith M. Surgical approach to epidural spinal cord compression. Hematol Oncol Clin North Am. 2006;20(6):1307-1317.
7. Bilsky M.H., Lis E., Raizer J., et al. The diagnosis and treatment of metastatic spinal tumor. Oncologist. 1999;4(6):459-469.
8. Gokaslan Z.L., York J.E., Walsh G.L., et al. Transthoracic vertebrectomy for metastatic spinal tumors. J Neurosurg. 1998;89(4):599-609.
9. Serlin R.C., Mendoza T.R., Nakamura Y., et al. When is cancer pain mild, moderate or severe? Grading pain severity by its interference with function. Pain. 1995;61(2):277-284.
10. Frankel H.L., Hancock D.O., Hyslop G., et al. The value of postural reduction in the initial management of closed injuries of the spine with paraplegia and tetraplegia. I. Paraplegia. 1969;7(3):179-192.
11. International Standards for Neurological and Functional Classifications of Spinal Cord Injury. Chicago: American Spinal Injury Association; 1982. Revised 1996.
12. Eastern Cooperative Oncology Group. Eastern Cooperative Oncology Group Performance Status Scale. March 25, 1998. Revised
13. Algra P., Heimans J., Valk J., et al. Do metastases in vertebrae begin in the body or the pedicles? Imaging study in 45 patients. Am J Roentgenol. 1992;158(6):1275-1279.
14. Gosfield E.III, Alavi A., Kneeland B. Comparison of radionuclide bone scans and magnetic resonance imaging in detecting spinal metastases. J Nucl Med. 1993;34(12):2191-2198.
15. Avrahami E., Tadmor R., Kaplisky N. The role of T2-weighted gradient echo in MRI demonstration of spinal multiple myeloma. Spine (Phila Pa 1976). 1993;18(13):1812-1815.
16. Frank J., Ling A., Patronas N., et al. Detection of malignant bone tumors: MR imaging vs scintigraphy. Am J Roentgenol. 1990;155(5):1043-1048.
17. Wild W.O., Porter R.W. Metastatic epidural tumor of the spine: a study of 45 cases. AMA Arch Surg. 1963;87(5):825-830.
18. Mullan J., Evans J.P. Neoplastic disease of the spinal extradural space: a review of fifty cases. AMA Arch Surg. 1957;74(6):900-907.
19. Hsu C.J., Chou W.Y., Chang W.N., et al. Clinical follow up after instrumentation-augmented lumbar spinal surgery in patients with unsatisfactory outcomes. J Neurosurg Spine. 2006;5(4):281-286.
20. Ortiz O., Pait T.G., McAllister P., et al. Postoperative magnetic resonance imaging with titanium implants of the thoracic and lumbar spine. Neurosurgery. 1996;38(4):741-745.
21. Gundry C.R., Fritts H.M. Magnetic resonance imaging of the musculoskeletal system: the spine. Part 8, section 3. Clin Orthop Relat Res. 1998;346:262-278.
22. Thariat J., Castelli J., Chanalet S., et al. CyberKnife stereotactic radiotherapy for spinal tumors: value of computed tomographic myelography in spinal cord delineation. Neurosurgery. 2009;64(Suppl 2):A60-A66.
23. Schiff D., O’Neill B.P., Wang C-H., et al. Neuroimaging and treatment implications of patients with multiple epidural spinal metastases. Cancer. 1998;83(8):1593-1601.
24. Moulopoulos L.A., Kumar A.J., Leeds N.E. A second look at unenhanced spinal magnetic resonance imaging of malignant leptomeningeal disease. Clin Imaging. 1997;21(4):252-259.
25. Guillevin R., Vallee J.N., Lafitte F., et al. Spine metastasis imaging: review of the literature. J Neuroradiol. 2007;34(5):311-321.
26. Traill Z., Richards M.A., Moore N.R. Magnetic resonance imaging of metastatic bone disease. Clin Orthop Relat Res. 1995;312:76-88.
27. Jones K., Schwartz R., Mantello M., et al. Fast spin-echo MR in the detection of vertebral metastases: comparison of three sequences. AJNR Am J Neuroradiol. 1994;15(3):401-407.
28. Mehta R., Marks M., Hinks R., et al. MR evaluation of vertebral metastases: T1-weighted, short-inversion-time inversion recovery, fast spin-echo, and inversion-recovery fast spin-echo sequences. AJNR Am J Neuroradiol. 1995;16(2):281-288.
29. Dwyer A.J., Frank J.A., Sank V.J., et al. Short-Ti inversion-recovery pulse sequence: analysis and initial experience in cancer imaging. Radiology. 1988;168(3):827-836.
29A. Bilsky M.H., Laufer I., Fourney D.R., et al. Reliability analysis of the epidural spinal cord compression scale. J Neurosurg Spine. 2010;13(3):324-328.
30. An H.S., Vaccaro A.R., Dolinskas C.A., et al. Differentiation between spinal tumors and infections with magnetic resonance imaging. Spine (Phila Pa 1976). 1991;16(8):S339.
31. Thajeb P., Huang K.M., Jeng C.M., et al. MRI appearance of spinal lesions: metastatic tumors or infections? Chin Med J. 1995;108(11):839-843.
32. Moulopoulos L.A., Yoshimitsu K., Johnston D.A., et al. MR prediction of benign and malignant vertebral compression fractures. J Magn Reson Imaging. 1996;6(4):667-674.
33. Brown A.L., Middleton G., Macvicar A.D., et al. T1-weighted magnetic resonance imaging in breast cancer vertebral metastases: changes on treatment and correlation with response to therapy. Clin Radiol. 1998;53(7):493-501.
34. Kato K., Aoki J., Endo K. Utility of FDG-PET in differential diagnosis of benign and malignant fractures in acute to subacute phase. Ann Nucl Med. 2003;17(1):41-46.
35. Dehdashti F., Siegel B.A., Griffeth L.K., et al. Benign versus malignant intraosseous lesions: discrimination by means of PET with 2-[F-18]fluoro-2-deoxy-D-glucose. Radiology. 1996;200(1):243-247.
36. Bredella M.A., Essary B., Torriani M., et al. Use of FDG-PET in differentiating benign from malignant compression fractures. Skeletal Radiol. 2008;37(5):405-413.
37. Laufer I., Lis E., Pisinski L., et al. The accuracy of [(18)F]fluorodeoxyglucose positron emission tomography as confirmed by biopsy in the diagnosis of spine metastases in a cancer population. Neurosurgery. 2009;64(1):107-113. discussion 113–114
38. Duarte P.S., Zhuang H., Castellucci P., et al. The receiver operating characteristic curve for the standard uptake value in a group of patients with bone marrow metastasis. Mol Imaging Biol. 2002;4(2):157-160.
39. Metser U., Lerman H., Blank A., et al. Malignant involvement of the spine: assessment by 18F-FDG PET/CT. J Nucl Med. 2004;45(2):279-284.
40. Mundy G.R. Hypercalcemia of malignancy revisited. J Clin Invest. 1988;82(1):1.
41. Paterson A.H.G. The potential role of bisphosphonates as adjuvant therapy in the prevention of bone metastases. Cancer. 2000;88(S12):3038-3046.
42. Kanis J.A., Percival R.C., Yates A.J.P., et al. Effects of diphosphonates in hypercalcaemia due to neoplasia. Lancet. 1986;327(8481):615-616.
43. Gerlach R., Krause M., Seifert V., et al. Hemostatic and hemorrhagic problems in neurosurgical patients. Acta Neurochir (Wien). 2009;151(8):873-900. discussion 900
44. DeLoughery T.G. Management of acquired bleeding problems in cancer patients. Emerg Med Clin North Am. 2009;27(3):423-444.
45. Streiff M.B. Diagnosis and initial treatment of venous thromboembolism in patients with cancer. J Clin Oncol. 2009;27(29):4889-4894.
46. Leon L., Rodriguez H., Tawk R.G., et al. The prophylactic use of inferior vena cava filters in patients undergoing high-risk spinal surgery. Ann Vasc Surg. 2005;19(3):442-447.
47. Gibbs M. The role of transdermal fentanyl patches in the effective management of cancer pain. Int J Palliat Nurs. 2009;15(7):354-359.
48. Gatti A., Reale C., Occhioni R., et al. Standard therapy with opioids in chronic pain management: ORTIBER study. Clin Drug Invest. 2009;1(Suppl 29):17-23.
49. Grubb M.R., Currier B.L., Pritchard D.J., et al. Primary Ewing’s sarcoma of the spine. Spine (Phila Pa 1976). 1994;19(3):309.
50. Byrne T. Spinal cord compression from epidural metastases. N Engl J Med. 1992;327(9):614-619.
51. Greenberg H.S., Kim J-H., Posner J.B. Epidural spinal cord compression from metastatic tumor: results with a new treatment protocol. Ann Neurol. 1980;8(4):361-366.
52. Kim R.Y., Spencer S.A., Meredith R.F., et al. Extradural spinal cord compression: analysis of factors determining functional prognosis—prospective study. Radiology. 1990;176(1):279-282.
53. Loblaw D., Laperriere N. Emergency treatment of malignant extradural spinal cord compression: an evidence-based guideline. J Clin Oncol. 1998;16(4):1613-1624.
54. Sørensen P.S. Effect of high-dose dexamethasone in carcinomatous metastasic spinal cord compression treated with radiotherapy: a randomised trial. Eur J Cancer. 1994;30(1):22.
55. Heimdal K., Hirschberg H., Slettebø H., et al. High incidence of serious side effects of high-dose dexamethasone treatment in patients with epidural spinal cord compression. J Neurooncol. 1992;12(2):141-144.
56. Maranzano E., Latini P., Beneventi S., et al. Radiotherapy without steroids in selected metastatic spinal cord compression patients: a phase II trial. Am J Clin Oncol. 1996;19(2):179-183.
57. Posner J.B., Howieson J., Cvitkovic E. “Disappearing” spinal cord compression: oncolytic effect of glucocorticoids (and other chemotherapeutic agents) on epidural metastases. Ann Neurol. 1977;2(5):409-413.
58. Yoneda T., Michigami T., Yi B., et al. Actions of bisphosphonate on bone metastasis in animal models of breast carcinoma. Cancer. 2000;88(S12):2979-2988.
59. Yin J.J., Selander K., Chirgwin J.M., et al. TGF-β signaling blockade inhibits PTHrP secretion by breast cancer cells and bone metastases development. J Clin Invest. 1999;103(2):197-206.
60. Thomas R.J., Guise T.A., Yin J.J., et al. Breast cancer cells interact with osteoblasts to support osteoclast formation. Endocrinology. 1999;140(10):4451-4458.
61. Mundy G.R., Yoneda T. Facilitation and suppression of bone metastasis. Clin Orthop Relat Res. 1995;312:34-44.
62. Mundy G.R. Bisphosphonates as anticancer drugs. N Engl J Med. 1998;339(6):398.
63. Yoneda T. Mechanisms of preferential metastasis of breast cancer to bone (Review). Int J Oncol. 1996;9(1):103-109.
64. Yoneda T. Cellular and molecular mechanisms of breast and prostate cancer metastasis to bone. Eur J Cancer (Oxford, England: 1990). 1998;34(2):240-245.
65. Wu S., Dahut W.L., Gulley J.L. The use of bisphosphonates in cancer patients. Acta Oncol. 2007;46(5):581-591.
66. Nakagawa N., Kinosaki M., Yamaguchi K., et al. RANK is the essential signaling receptor for osteoclast differentiation factor in osteoclastogenesis. Biochem Biophys Res Commun. 1998;253(2):395-400.
67. Dougall W.C., Glaccum M., Charrier K., et al. RANK is essential for osteoclast and lymph node development. Genes Dev. 1999;13(18):2412-2424.
68. Simonet W.S., Lacey D.L., Dunstan C.R., et al. Osteoprotegerin: a novel secreted protein involved in the regulation of bone density. Cell. 1997;89(2):309-319.
69. Pearse R.N., Sordillo E.M., Yaccoby S., et al. Multiple myeloma disrupts the TRANCE/ osteoprotegerin cytokine axis to trigger bone destruction and promote tumor progression. Proc Natl Acad Sci U S A. 2001;98(20):11581-11586.
70. Grotewold L., Ruther U. The Wnt antagonist Dickkopf-1 is regulated by Bmp signaling and c-Jun and modulates programmed cell death. EMBO J. 2002;21(5):966-975.
71. Mukhopadhyay M., Shtrom S., Rodriguez-Esteban C., et al. Dickkopf1 is required for embryonic head induction and limb morphogenesis in the mouse. Dev Cell. 2001;1(3):423-434.
72. Tian E., Zhan F., Walker R., et al. The role of the Wnt-signaling antagonist DKK1 in the development of osteolytic lesions in multiple myeloma. N Engl J Med. 2003;349(26):2483-2494.
73. Kang Y., Siegel P.M., Shu W., et al. A multigenic program mediating breast cancer metastasis to bone. Cancer Cell. 2003;3(6):537-549.
74. Kang Y., He W., Tulley S., et al. Breast cancer bone metastasis mediated by the Smad tumor suppressor pathway. Proc Natl Acad Sci U S A. 2005;102(39):13909-13914.
75. Boonekamp P.M., van der Wee-Pals L.J., van Wijk-van Lennep M.M., et al. Two modes of action of bisphosphonates on osteoclastic resorption of mineralized matrix. Bone Miner. 1986;1(1):27-39.
76. Fleisch H. Bisphosphonates—history and experimental basis. Bone. 1987;8(Suppl 1):S23-S28.
77. Lipton A. Bisphosphonates and breast carcinoma. Cancer. 1997;80(S8):1668-1673.
78. Terpos E., Rahemtulla A. Bisphosphonate treatment for multiple myeloma. Drugs Today (Barc.). 2004;40(1):29-40.
79. Hortobagyi G.N., Theriault R.L., Porter L., et al. Efficacy of pamidronate in reducing skeletal complications in patients with breast cancer and lytic bone metastases. Protocol 19 Aredia Breast Cancer Study Group. N Engl J Med. 1996;335(24):1785-1791.
80. Diel I.J., Solomayer E-F., Costa S.D., et al. Reduction in new metastases in breast cancer with adjuvant clodronate treatment. N Engl J Med. 1998;339(6):357-363.
81. Berenson J., Lichtenstein A., Porter L., et al. Long-term pamidronate treatment of advanced multiple myeloma patients reduces skeletal events. Myeloma Aredia Study Group. J Clin Oncol. 1998;16(2):593-602.
82. Terpos E., Palermos J., Tsionos K., et al. Effect of pamidronate administration on markers of bone turnover and disease activity in multiple myeloma. Eur J Haematol. 2000;65(5):331-336.
83. van Holten-Verzantvoort A.T., Bijvoet O.L., Cleton F.J., et al. Reduced morbidity from skeletal metastases in breast cancer patients during long-term bisphosphonate (APD) treatment. Lancet. 1987;2(8566):983-985.
84. Paterson A., Powles T., Kanis J., et al. Double-blind controlled trial of oral clodronate in patients with bone metastases from breast cancer. J Clin Oncol. 1993;11(1):59-65.
85. McCloskey E.V., Spector T.D., Eyres K.S., et al. The assessment of vertebral deformity: a method for use in population studies and clinical trials. Osteoporosis Int. 1993;3(3):138-147.
86. Delmas P.D. The use of clodronate in multiple myeloma. Bone. 1991;12(Suppl 1):S31-S34.
87. Clemens M.R., Fessele K., Heim M.E. Multiple myeloma: effect of daily dichloromethylene bisphosphonate on skeletal complications. Ann Hematol. 1993;66(3):141-146.
88. Berenson J.R., Lichtenstein A., Porter L., et al. Efficacy of pamidronate in reducing skeletal events in patients with advanced multiple myeloma. N Engl J Med. 1996;334(8):488-493.
89. Theriault R.L., Lipton A., Hortobagyi G.N., et al. Pamidronate reduces skeletal morbidity in women with advanced breast cancer and lytic bone lesions: a randomized, placebo-controlled trial. Protocol 18. Aredia Breast Cancer Study Group. J Clin Oncol. 1999;17(3):846-854.
90. Lipton A., Theriault R.L., Hortobagyi G.N., et al. Pamidronate prevents skeletal complications and is effective palliative treatment in women with breast carcinoma and osteolytic bone metastases. Cancer. 2000;88(5):1082-1090.
91. Berenson J.R., Rosen L.S., Howell A., et al. Zoledronic acid reduces skeletal-related events in patients with osteolytic metastases. Cancer. 2001;91(7):1191-1200.
92. Major P.P., Lipton A., Berenson J., et al. Oral bisphosphonates. Cancer. 2000;88(1):6-14.
93. Kanis J.A. A high incidence of vertebral fracture in women with breast cancer. Br J Cancer. 1999;79(7/8):1179.
94. Fuleihan Gel H., Salamoun M., Mourad Y.A., et al. Pamidronate in the prevention of chemotherapy-induced bone loss in premenopausal women with breast cancer: a randomized controlled trial. J Clin Endocrinol Metab. 2005;90(6):3209-3214.
95. Kanis J.A., Powles T., Paterson A.H.G., et al. Clodronate decreases the frequency of skeletal metastases in women with breast cancer. Bone. 1996;19(6):663-667.
96. Powles T.J., Paterson A.H.G., Neventaus S., et al. Adjuvant clodronate reduces the incidence of bone metastases in patients with primary operable breast cancer. Proc Am Soc Clin Oncol. 17, 1998. Abstract 468, 1998
97. Elomaa I., Blomqvist C., Grohn P., et al. Long-term controlled trial with diphosphonate in patients with osteolytic bone metastases. Lancet. 1983;1(8317):146-149.
98. Kristensen B., Ejlertsen B., Mouridsen H.T., et al. Bisphosphonate treatment in primary breast cancer: results from a randomised comparison of oral pamidronate versus no pamidronate in patients with primary breast cancer. Acta Oncol. 2008;47(4):740-746.
99. Boissier S., Magnetto S., Frappart L., et al. Bisphosphonates inhibit prostate and breast carcinoma cell adhesion to unmineralized and mineralized bone extracellular matrices. Cancer Res. 1997;57(18):3890-3894.
100. Van der Pluijm G. Bisphosphonates inhibit the adhesion of breast cancer cells to bone matrices in vitro. J Clin Invest. 1996;98(3):698.
101. Magnetto S. Additive antitumor activities of taxoids in combination with the bisphosphonate ibandronate against invasion and adhesion of human breast carcinoma cells to bone. Int J Cancer. 1999;83(2):263.
102. Smith J.A. Palliation of painful bone metastases from prostate cancer using sodium etidronate: results of a randomized, prospective, double-blind, placebo-controlled study. J Urol. 1989;141(1):85-87.
103. Elomaa I., Kylmälä T., Tammela T., et al. Effect of oral clodronate on bone pain. Intl Urol Nephrol. 1992;24(2):159-166.
104. Kylml T., Tammela T., Risteli L., et al. Evaluation of the effect of oral clodronate on skeletal metastases with type 1 collagen metabolites. A controlled trial of the Finnish Prostate Cancer Group. Eur J Cancer. 1993;29A(6):821-825.
105. Adami S., Salvagno G., Guarrera G., et al. Dichloromethylene-diphosphonate in patients with prostatic carcinoma metastatic to the skeleton. J Urol. 1985;134(6):1152-1154.
106. Kylml T., Tammela T.L., Lindholm T.S., et al. The effect of combined intravenous and oral clodronate treatment on bone pain in patients with metastatic prostate cancer. Ann Chir Gynaecol. 1994;83(4):316-319.
107. Cresswell S.M., English P.J., Hall R.R., et al. Pain relief and quality-of-life assessment following intravenous and oral clodronate in hormone-escaped metastatic prostate cancer. Br J Urol. 1995;76(3):360-365.
108. Lee M.V. Bisphosphonate treatment inhibits the growth of prostate cancer cells. Cancer Res. 2001;61(6):2602.
109. Glover D., Lipton A., Keller A., et al. Intravenous pamidronate disodium treatment of bone metastases in patients with breast cancer. A dose-seeking study. Cancer. 1994;74(11):2949-2955.
110. Flanagan A., Chambers T. Inhibition of bone resorption by bisphosphonates: interactions between bisphosphonates, osteoclasts, and bone. Calcif Tissue Int. 1991;49(6):407-415.
111. Fleisch H. Bisphosphonates: a new class of drugs in diseases of bone and calcium metabolism. Rec Results Cancer Res. 1989;116:1-28.
112. Ernst D.S., MacDonald R.N., Paterson A.H., et al. A double-blind, crossover trial of intravenous clodronate in metastatic bone pain. J Pain Symptom Manage. 1992;7(1):4-11.
113. Ernst D.S., Penny B., Neil H., et al. A randomized, controlled trial of intravenous clodronate in patients with metastatic bone disease and pain. J Pain Symptom Manage. 1997;13(6):319-326.
114. van Holten-Verzantvoort A.T., Zwinderman A.H., Aaronson N.K., et al. The effect of supportive pamidronate treatment on aspects of quality of life of patients with advanced breast cancer. Eur J Cancer. 1991;27(5):544-549.
115. Coleman R.E., Woll P.J., Miles M., et al. Treatment of bone metastases from breast cancer with (3-amino-1-hydroxypropylidene)-1,1-bisphosphonate (APD). Br J Cancer. 1988;58(5):621-625.
116. Kyle R.A., Yee G.C., Somerfield M.R., et al. American Society of Clinical Oncology 2007 clinical practice guideline update on the role of bisphosphonates in multiple myeloma. J Clin Oncol. 2007;25(17):2464-2472.
117. Zervas K., Verrou E., Teleioudis Z., et al. Incidence, risk factors and management of osteonecrosis of the jaw in patients with multiple myeloma: a single-centre experience in 303 patients. Br J Haematol. 2006;134(6):620-623.
118. Graham G.P., Dent C.M., Burgess N., et al. Urinary retention in prostatic carcinoma: obstructive or neurogenic? Br J Hosp Med. 1993;49(10):733-734.
119. Tanaka M., Nakahara S., Ito Y., et al. Surgical treatment of metastatic vertebral tumors. Acta Med Okayama. 2009;63(3):145-150.
120. Cooper P.R., Errico T.J., Martin R., et al. A systematic approach to spinal reconstruction after anterior decompression for neoplastic disease of the thoracic and lumbar spine. Neurosurgery. 1993;32(1):1-8.
121. DeWald R.L., Bridwell K.H., Prodromas C., et al. Reconstructive spinal surgery as palliation for metastatic malignancies of the spine. Spine (Phila Pa 1976). 1985;10(1):21-26.
122. Hosono N., Yonenobu K., Fuji T., et al. Vertebral body replacement with a ceramic prosthesis for metastatic spinal tumors. Spine (Phila Pa 1976). 1995;20(22):2454-2462.
123. Harrington K. The use of methylmethacrylate for vertebral-body replacement and anterior stabilization of pathological fracture-dislocations of the spine due to metastatic malignant disease. J Bone Joint Surg [Am]. 1981;63(1):36-46.
124. Harrington K.D. Anterior cord decompression and spinal stabilization for patients with metastatic lesions of the spine. J Neurosurg. 1984;61(1):107-117.
125. Harrington K.D. Anterior decompression and stabilization of the spine as a treatment for vertebral collapse and spinal cord compression from metastatic malignancy. Clin Orthop Relat Res. 1988;233:177-197.
126. Klekamp J., Samii H. Surgical results for spinal metastases. Acta Neurochir (Wien). 1998;140(9):957-967.
127. Kostuik J.P. Anterior spinal cord decompression for lesions of the thoracic and lumbar spine, techniques, new methods of internal fixation results. Spine (Phila Pa 1976). 1983;8(5):512-531.
128. Perrin R.G., McBroom R.J. Anterior versus posterior decompression for symptomatic spinal metastasis. Can J Neurol Sci. 1987;14(1):75-80.
129. Perrin R.G., McBroom R.J. Spinal fixation after anterior decompression for symptomatic spinal metastasis. Neurosurgery. 1988;22(2):324-327.
130. Siegal T. Surgical decompression of anterior and posterior malignant epidural tumors compressing the spinal cord: a prospective study. Neurosurgery. 1985;17(3):424-432.
131. Sundaresan N., Digiacinto G.V., Hughes J.E., et al. Treatment of neoplastic spinal cord compression: results of a prospective study. Neurosurgery. 1991;29(5):645-650.
132. Sundaresan N., Choi I.S., Hughes J.E.O., et al. Treatment of spinal metastases from kidney cancer by presurgical embolization and resection. J Neurosurg. 1990;73(4):548-554.
133. Sundaresan N., DiGiacinto G., Krol G., et al. Spondylectomy for malignant tumors of the spine. J Clin Oncol. 1989;7(10):1485-1491.
134. Walsh G.L., Gokaslan Z.L., McCutcheon I.E., et al. Anterior approaches to the thoracic spine in patients with cancer: indications and results. Ann Thorac Surg. 1997;64(6):1611-1618.
135. Akeyson E.W., McCutcheon I.E. Single-stage posterior vertebrectomy and replacement combined with posterior instrumentation for spinal metastasis. J Neurosurg. 1996;85(2):211-220.
136. Bauer H.C.F., Wedin R. Survival after surgery for spinal and extremity metastases: prognostication in 241 patients. Acta Orthop. 1995;66(2):143-146.
137. Bridwell K.H., Jenny A.B., Saul T., et al. Posterior segmental spinal instrumentation (PSSI) with posterolateral decompression and debulking for metastatic thoracic and lumbar spine disease: limitations of the technique. Spine (Phila Pa 1976). 1988;13(12):1383-1394.
138. Cahill D.W., Kumar R. Palliative subtotal vertebrectomy with anterior and posterior reconstruction via a single posterior approach. J Neurosurg Spine. 1999;90(1):42-47.
139. Sundaresan N., Steinberger A.A., Moore F., et al. Indications and results of combined anterior–posterior approaches for spine tumor surgery. J Neurosurg. 1996;85(3):438-446.
140. Magerl F., Coscia M.F. Total posterior vertebrectomy of the thoracic or lumbar spine. Clin Orthop Relat Res. 1988;232:62-69.
141. Cobb C.A., Leavens M.E., Eckles N. Indications for nonoperative treatment of spinal cord compression due to breast cancer. J Neurosurg. 1977;47(5):653-658.
142. Constans J.P., de Divitiis E., Donzelli R., et al. Spinal metastases with neurological manifestations. Review of 600 cases. J Neurosurg. 1983;59(1):111-118.
143. Dunn R.C., Kelly W.A., Wohns R.N., et al. Spinal epidural neoplasia. A 15-year review of the results of surgical therapy. J Neurosurg. 1980;52(1):47-51.
144. Friedman M., Kim T.H., Panahon A.M. Spinal cord compression in malignant lymphoma: treatment and results. Cancer. 1976;37(3):1485-1491.
145. Gilbert R.W., Kim J-H., Posner J.B. Epidural spinal cord compression from metastatic tumor: diagnosis and treatment. Ann Neurol. 1978;3(1):40-51.
146. Hall A.J. The results of laminectomy for compression of the cord or cauda equina by extradural malignant tumour. J Bone Joint Surg [Br]. 1973;55(3):497.
147. Khan F.R., Glicksman A.S., Chu F.C., et al. Treatment by radiotherapy of spinal cord compression due to extradural metastases. Radiology. 1967;89(3):495-500.
148. Kleinman W.B., Kiernan H.A., Michelsen W.J. Metastatic cancer of the spinal column. Clin Orthop Relat Res. 1978;136:166-172.
149. Livingston K.E., Perrin R.G. The neurosurgical management of spinal metastases causing cord and cauda equina compression. J Neurosurg. 1978;49(6):839-843.
150. Marshall L.F., Langfitt T.W. Combined therapy for metastatic extradural tumors of the spine. Cancer. 1977;40(5):2067-2070.
151. Nicholls P.J., Jarecky T.W. The value of posterior decompression by laminectomy for malignant tumors of the spine. Clin Orthop Relat Res. 1985;201:210-213.
152. Brice J., McKissock W. Surgical treatment of malignant extradural spinal tumours. Br Med J. 1965;1(5446):1341-1344.
153. Smith R. An evaluation of surgical treatment for spinal cord compression due to metastatic carcinoma. J Neurol Neurosurg Psychiatry. 1965;28(2):152-158.
154. Vieth R.G., Odom G.L. Extradural spinal metastases and their neurosurgical treatment. J Neurosurg. 1965;23(5):501-508.
155. White W.A., Patterson R.H.Jr., Bergland R.M. Role of surgery in the treatment of spinal cord compression by metastatic neoplasm. Cancer. 1971;27(3):558-561.
156. Wright R.L. Malignant tumors in the spinal extradural space: results of surgical treatment. Ann Surg. 1963;157(2):227.
157. Young R.F., Post E.M., King G.A. Treatment of spinal epidural metastases. J Neurosurg. 1980;53(6):741-748.
158. Bilsky M.H., Lis E., Raizer J., et al. The diagnosis and treatment of metastatic spinal tumor. Oncologist. 1999;4(6):459-469.
159. Maranzano E., Latini P. Effectiveness of radiation therapy without surgery in metastatic spinal cord compression: final results from a prospective trial. Int J Radiat Oncol Biol Phys. 1995;32(4):959-967.
160. Yamada Y., Bilsky M.H., Lovelock D.M., et al. High-dose, single-fraction image-guided intensity-modulated radiotherapy for metastatic spinal lesions. Int J Radiat Oncol Biol Phys. 2008;71(2):484-490.
161. Gerszten P.C., Burton S.A., Ozhasoglu C., et al. Stereotactic radiosurgery for spinal metastases from renal cell carcinoma. J Neurosurg Spine. 2005;3(4):288-295.
162. Gerszten P.C., Burton S.A., Quinn A.E., et al. Radiosurgery for the treatment of spinal melanoma metastases. Stereotact Funct Neurosurg. 2005;83(5–6):213-221.
163. Gerszten P.C., Burton S.A., Welch W.C., et al. Single-fraction radiosurgery for the treatment of spinal breast metastases. Cancer. 2005;104(10):2244-2254.
164. Gerszten P.C., Burton S.A., Ozhasoglu C., et al. Radiosurgery for spinal metastases: clinical experience in 500 cases from a single institution. Spine (Phila Pa 1976). 2007;32(2):193-199.
165. Manabe S., Tateishi A., Abe M., et al. Surgical treatment of metastatic tumors of the spine. Spine (Phila Pa 1976). 1989;14(1):41-47.
166. Moore A.J., Uttley D. Anterior decompression and stabilization of the spine in malignant disease. Neurosurgery. 1989;24(5):713-717.
167. King G.J., Kostuik J.P., McBroom R.J., et al. Surgical management of metastatic renal carcinoma of the spine. Spine. 1991;16(3):265-271.
168. Sundaresan N., Sachdev V.P., Holland J.F., et al. Surgical treatment of spinal cord compression from epidural metastasis. J Clin Oncol. 1995;13(9):2330-2335.
169. Wang J.C., Boland P., Mitra N., et al. Single-stage posterolateral transpedicular approach for resection of epidural metastatic spine tumors involving the vertebral body with circumferential reconstruction: results in 140 patients. Invited submission from the Joint Section Meeting on Disorders of the Spine and Peripheral Nerves, March 2004. J Neurosurg Spine. 2004;1(3):287-298.
170. Ernstberger T., Bruning T., Konig F. Vertebrectomy and anterior reconstruction for the treatment of spinal metastases. Acta Orthop Belg. 2005;71(4):459-466.
171. Jansson K.A., Bauer H.C. Survival, complications and outcome in 282 patients operated for neurological deficit due to thoracic or lumbar spinal metastases. Eur Spine J. 2006;15(2):196-202.
172. Street J., Fisher C., Sparkes J., et al. Single-stage posterolateral vertebrectomy for the management of metastatic disease of the thoracic and lumbar spine: a prospective study of an evolving surgical technique. J Spinal Disord Tech. 2007;20(7):509-520.
173. Chen Y.J., Chang G.C., Chen H.T., et al. Surgical results of metastatic spinal cord compression secondary to non-small cell lung cancer. Spine (Phila Pa 1976). 2007;32(15):E413-E418.
174. Mannion R.J., Wilby M., Godward S., et al. The surgical management of metastatic spinal disease: prospective assessment and long-term follow-up. Br J Neurosurg. 2007;21(6):593-598.
175. Feiz-Erfan I., Rhines L.D., Weinberg J.S. The role of surgery in the management of metastatic spinal tumors. Semin Oncol. 2008;35(2):108-117.
176. Cybulski G.R. Methods of surgical stabilization for metastatic disease of the spine. Neurosurgery. 1989;25(2):240-252.
177. Melcher R.P., Harms J. Biomechanics and materials of reconstruction after tumor resection in the spinal column. Orthop Clin North Am. 2009;40(1):65-74. vi
178. Mazel C., Balabaud L., Bennis S., et al. Cervical and thoracic spine tumor management: surgical indications, techniques, and outcomes. Orthop Clin North Am. 2009;40(1):75-92. vi-vii
179. Vitaz T.W., Oishi M., Welch W.C., et al. Rotational and transpositional flaps for the treatment of spinal wound dehiscence and infections in patient populations with degenerative and oncological disease. J Neurosurg. 2004;100(Suppl 1 Spine):46-51.
180. Burton A.W., Mendel E. Vertebroplasty and kyphoplasty. Pain Physician. 2003;6(3):335-341.
181. Hentschel S.J., Burton A.W., Fourney D.R., et al. Percutaneous vertebroplasty and kyphoplasty performed at a cancer center: refuting proposed contraindications. J Neurosurg Spine. 2005;2(4):436-440.
182. Bartolozzi B., Nozzoli C., Pandolfo C., et al. Percutaneous vertebroplasty and kyphoplasty in patients with multiple myeloma. Eur J Haematol. 2006;76(2):180-181.
183. Sundaresan N., Galicich J.H., Lane J.M., et al. Treatment of odontoid fractures in cancer patients. J Neurosurg. 1981;54(2):187-192.
184. Bilsky M.H., Shannon F.J., Sheppard S., et al. Diagnosis and management of a metastatic tumor in the atlantoaxial spine. Spine (Phila Pa 1976). 2002;27(10):1062-1069.
185. Tokuhashi Y., Matsuzaki H., Oda H., et al. A revised scoring system for preoperative evaluation of metastatic spine tumor prognosis. Spine (Phila Pa 1976). 2005;30(19):2186-2191.
186. Tomita K., Kawahara N., Kobayashi T., et al. Surgical strategy for spinal metastases. Spine (Phila Pa 1976). 2001;26(3):298-306.
187. Rades D., Fehlauer F., Sheikh-Sarraf M., et al. Toxicity of two cisplatin-based radiochemotherapy regimens for the treatment of patients with stage III/IV head and neck cancer. Head Neck. 2008;30(2):235-241.
188. Olerud C., Jónsson H., Löfberg A-M., et al. Embolization of spinal metastases reduces peroperative blood loss: 21 patients operated on for renal cell carcinoma. Acta Orthop. 1993;64(1):9-12.
189. Sundaresan N., Galicich J.H., Bains M.S., et al. Vertebral body resection in the treatment of cancer involving the spine. Cancer. 1984;53(6):1393-1396.
190. Guzman R., Dubach-Schwizer S., Heini P., et al. Preoperative transarterial embolization of vertebral metastases. Eur Spine J. 2005;14(3):263-268.
191. Bilsky M.H., Boland P., Lis E., et al. Single-stage posterolateral transpedicle approach for spondylectomy, epidural decompression, and circumferential fusion of spinal metastases. Spine (Phila Pa 1976). 2000;25(17):2240-2249. discussion 2250
192. Di Martino A., Vincenzi B., Denaro L., et al. “Internal bracing” surgery in the management of solid tumor metastases of the thoracic and lumbar spine. Oncol Rep. 2009;21(2):431-435.
193. Liu J.K., Apfelbaum R.I., Chiles B.W.3rd, et al. Cervical spinal metastasis: anterior reconstruction and stabilization techniques after tumor resection. Neurosurg Focus. 2003;15(5):E2.
194. Tomita K., Kawahara N., Baba H., et al. Total en bloc spondylectomy for solitary spinal metastases. Int Orthop. 1994;18(5):291-298.
195. Boriani S., Bandiera S., Donthineni R., et al. Morbidity of en bloc resections in the spine. Eur Spine J. 2010;19(2):231-241.
196. Boriani S., Biagini R., De Iure F., et al. Resection surgery in the treatment of vertebral tumors. Chir Organi Mov. 1998;83(1-2):53-64.
197. Fidler M. Radical resection of vertebral body tumours. A surgical technique used in ten cases. J Bone Joint Surg [Br]. 1994;76(5):765-772.
198. Heary R.F., Vaccaro A.R., Benevenia J., et al. “En-bloc” vertebrectomy in the mobile lumbar spine—diagnosis and clinical management. Surg Neurol. 1998;50:548-556.
199. Bauer H.C. Indications for surgical treatment of spinal metastases. Orthop Traumatol Rehabil. 2003;5(2):164-166.
200. Dickman C.A., Rosenthal D., Karahalios D.G., et al. Thoracic vertebrectomy and reconstruction using a microsurgical thoracoscopic approach. Neurosurgery. 1996;38(2):279-293.
201. Rosenthal D., Marquardt G., Lorenz R., et al. Anterior decompression and stabilization using a microsurgical endoscopic technique for metastatic tumors of the thoracic spine. J Neurosurg. 1996;84(4):565-572.