CHAPTER 397 Spinal Vascular Malformations
Patients with spinal arteriovenous malformations (AVMs) may have an insidious, subacute, or acute onset of symptoms, depending on the type of AVM and the mechanism of cord injury. Before recommending treatment, one must consider the expected clinical course based on the natural history of the type of vascular abnormality and the potential risks and benefits of the proposed treatment. However, knowledge of the natural history of spinal vascular abnormalities is incomplete. As with other rare disorders for which therapy is attempted, the natural history is known only from retrospective studies, and additional factors mitigate the usefulness of previous studies. Most information on the natural history of spinal AVMs was acquired when they were all considered to be congenital AVMs of the spinal cord. In the past 2 decades there have been significant advances in the understanding and treatment of spinal AVMs. Fundamental observations have been made regarding their true anatomy and pathophysiology, which in turn have enabled more effective and safer treatment. It is now generally recognized that the spinal vascular abnormalities are not a single entity but consist of several biologically distinct forms. Based on an understanding of the epidemiology, anatomy, pathophysiology, mechanism of origin, clinical findings, and prognosis, four major types of spinal vascular abnormalities are now recognized: dural arteriovenous fistulas (AVFs), AVMs of the spinal cord, perimedullary (pial) AVFs, and cavernous angiomas (Table 397-1).
TABLE 397-1 Classification of Spinal Vascular Abnormalities Based on Distinct Biologic Features
Adapted from Oldfield E, Doppman J. Spinal arteriovenous malformations. Clin Neurosurg. 1988;34:161-183.
Although the clinical manifestations suggest a specific pathophysiologic process, which implies the particular type and location of an AVM, a definite diagnosis requires a methodical imaging evaluation. Before embarking on treatment, the clinician must consider (1) the type of vascular abnormality affecting the patient, (2) the probable clinical course based on the natural history of that type of lesion in relation to the age and overall medical condition of the patient, (3) the specific vascular anatomy of the lesion and its relationship to the vessels supplying the spinal cord (Fig. 397-1), and (4) the relative risks and benefits of the proposed treatment.
History and Classification
Elsberg performed the first successful operation for a spinal AVM in 1914.1 Before surgery his patient was densely paraparetic and bedridden and had sensory loss below the T9 dermatomal level. Operative exploration revealed an abnormal posterior “dilated spinal vein” that entered the spinal dura adjacent to the dural penetration of the posterior root of the ninth thoracic spinal nerve. Elsberg excised 2 cm of the abnormal vessel as it penetrated the spinal dura. Postoperatively, the patient improved dramatically, with complete neurological recovery by 3 months after surgery.1,2
The classification of spinal AVMs has evolved with and been limited by the technology available to study them. The earliest analyses of spinal AVMs were based solely on postmortem histopathologic examination. In 1925, Sargent reviewed the 21 previously reported cases of spinal AVM and concluded that 19 of them were “venous angiomas.”3 In his 1943 review of 110 cases, Wyburn-Mason classified spinal cord vascular malformations into two histologic groups, arteriovenous angiomas and purely venous angiomas, the latter accounting for approximately two thirds of all cases.4 The venous angioma type was described as a mass of distended, blue pial vessels on the surface of the spinal cord. For consistency with Virchow’s original classification of vascular anomalies, Wyburn-Mason called this type “angioma racemosum venosum.” Thus, these early classifications suggested that a majority of spinal AVMs were venous lesions on the surface of the cord.5
The introduction of spinal aortography and then selective spinal arteriography by Doppman, Djindjian, and their coworkers in the 1960s allowed clinicians to confirm the presence of spinal AVMs and to define their anatomy in living patients for the first time. Demonstration of the radiographic anatomy of spinal AVMs in vivo resulted in a more precise arteriographic classification of these lesions based on their vascular anatomy and pattern of blood flow rather than on postmortem pathology.6–10 Hence, spinal AVMs were classified radiographically into three categories.6,7 Type I, the “single coiled vessel type,” constituted 80% to 85% of all spinal AVMs. Type II, or “glomus,” and type III, or “juvenile,” AVMs accounted for 15% to 20% of all lesions. Although investigators were able to demonstrate the site of the AVF only with type II and III lesions, in all three types the nidus was thought to be within the spinal cord.5
The single coiled vessel lesion, the most common of the three types, was described as a single, continuous, tightly coiled vessel on the surface of the spinal cord. Blood flow through this lesion was generally slow, with nearly 20 seconds often required for clearance of contrast material. These spinal AVMs were usually supplied by one or two feeding vessels that were thought to supply the AVM but not the spinal cord. In contrast to the juvenile and glomus types, in this type the nidus of the arteriovenous shunt was not identified. The arteriographic classification was adopted by surgeons, who theorized, but were never able to demonstrate, that the nidus of the AVM was located in multiple penetrating parenchymal vessels between the dorsolateral arterial plexus and the single coiled vessel on the cord surface. This theory, concomitant with the advent of microneurosurgery in the 1960s, led to surgical stripping of the engorged, thin-walled vessels from the surface of the cord as the preferred treatment.11,12
In 1977, Kendall and Logue identified AVFs in the dural sleeve of spinal nerve roots in nine patients who had radiographic findings otherwise completely consistent with the single coiled vessel type of spinal AVM.13 After simple surgical excision of the dural AVF, the patients improved. Hence, lesions that were previously considered pial venous angiomas or single coiled vessel AVMs by earlier classifications were now recognized to be dural AVFs in the spinal nerve root sleeve and adjacent spinal dura. The typical single coiled vessel morphology of these lesions is now acknowledged to be the result of chronic metamorphosis of the vessels of the coronal venous plexus by arterialization of normal pial veins into dilated, serpentine, thin-walled vessels on the surface of the spinal cord.
Two additional advances in the understanding and classification of spinal AVMs have occurred in the past 3 decades. First was the recognition by Djindjian and colleagues in 1977 that some intradural lesions that were previously considered to be AVMs of the spinal cord are actually simple AVFs in the pia (perimedullary AVFs).14 Second was the recognition of an additional important category of vascular lesion that was previously thought to be extremely rare because there was no diagnostic study that could reveal it before the introduction of computed tomographic (CT) scanning. This lesion is the cavernous angioma (also called cavernous malformation), which has a predisposition to hemorrhage repeatedly. Its true incidence has become evident only since the introduction of magnetic resonance imaging (MRI).
With the recognition that former type I AVMs (the “single coiled vessel” type) were actually dural AVFs, identification of perimedullary AVFs, recognition of the clinical importance of cavernous angiomas, and increased understanding of the pathophysiology underlying the natural history and clinical manifestations of the different types of spinal AVMs, it has become clear that each major type is a distinct biologic entity (Figs. 397-2 to 397-7).14–34 Thus, reclassification of spinal AVMs was necessary to provide terminology that accurately described the lesion and allowed logical categorization based on biologically distinct categories rather than on the use of old terms and categorization schemes that were based on a mistaken understanding of the true nature of the lesions, used abstract terms (e.g., type I spinal AVM) rather than comprehensible and clearly descriptive terms for the various lesions, and permitted different authors to use the same term in substantially different ways.6,7 Hence, four types of spinal vascular malformations with definite biologic differences (e.g., anatomy, pathogenesis, pathophysiology, epidemiology, clinical features) are now recognized: dural AVFs, intradural vascular malformations (which include AVMs of the spinal cord and intradural AVFs), and cavernous angiomas of the spinal cord (see Table 397-1 and Fig. 397-7). In dural AVFs, the arteriovenous nidus is embedded in the dura, usually in the spinal nerve root sleeve and contiguous dura (see Fig. 397-2). In intradural vascular malformations, the arteriovenous shunt is either buried in the substance of the spinal cord (AVMs; see Figs. 397-3 and 397-4) or in the pia or subarachnoid space (perimedullary AVFs; see Fig. 397-5). Cavernous angiomas (see Fig. 397-6), which are not demonstrated by spinal arteriography but have highly characteristic MRI findings, are rarely mistaken for the other types of spinal vascular abnormalities.
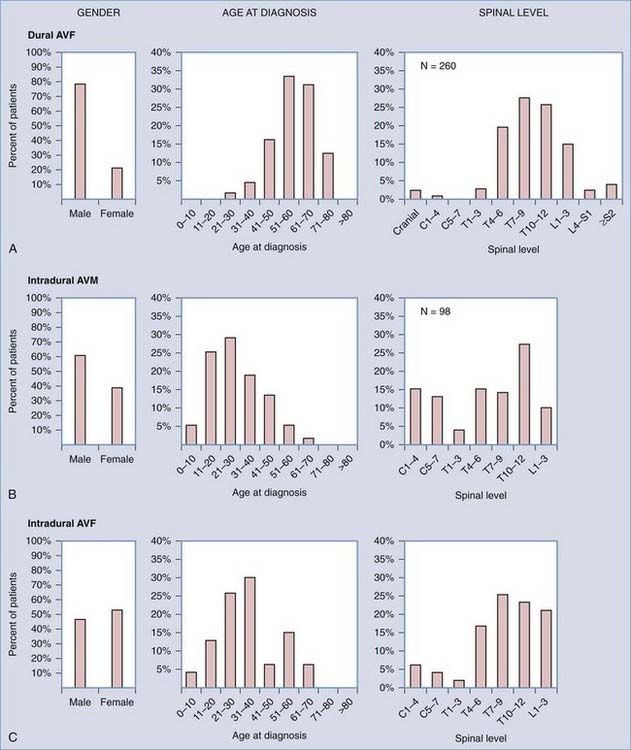
FIGURE 397-7 Distribution of patients with spinal dural arteriovenous fistulas (AVFs) (A), intradural spinal arteriovenous malformations (AVMs) (B; most reports include AVMs and AVFs), and perimedullary AVFs (C) by gender, age at diagnosis, and level of AVF along the long axis of the spine. The distinct differences in the distribution of patients with these three types of spinal vascular abnormalities indicate that they are biologically discrete disorders and support the general classification depicted in Table 397-1.
(Data compiled from the following references: dural AVFs—gender, 15–28 age, 15–17,20,24,25 and level 14–19,21,23,24–28; intradural AVMs—gender, 16,27,28,29,30 and age and level16,27,28,30; and intradural AVFs—gender, age, and level. 27,31–34 From Oldfield EH, Bennett A, Chen MY, et al. Successful management of patients with spinal dural arteriovenous fistulas and negative arteriography. J Neurosurg. 2002;9(2 suppl)6:220-229.
Vascular anatomy and Pathogenesis
Normal Vascular Anatomy of the Spinal Cord and Dura
Arterial Anatomy
The spinal cord is supplied by two arterial networks, the anterior and posterior arterial systems (see Fig. 397-1). The anterior plexus is derived from the anterior spinal artery, which extends along the entire length of the spinal cord in the anterior median fissure and is the origin of the sulcal arteries, which leave the anterior spinal artery at a 90-degree angle to perfuse the anterior two thirds of the spinal cord. The anterior horns, corticospinal tracts, and spinothalamic tracts are perfused by this anterior spinal arterial distribution. The posterior system is a network of plexiform collaterals between two posterolateral arteries. It supplies the posterior third of the spinal cord, including a portion of the corticospinal tracts and the entire dorsal column.7,8,10,31,35,36 Both the anterior and the posterior arterial systems are supplied by medullary arteries.
During the first 6 months of gestation, paired bilateral medullary arteries supply the anterior and posterolateral arteries at each segmental level of the spinal cord. By the third trimester, however, most medullary arteries have regressed, and in adults, only 6 to 10 remain to supply the spinal cord.31 The medullary arteries in the cervical region are derived from the vertebral arteries and branches of the thyrocervical trunk. The medullary arteries in the thoracic and lumbar regions arise from a branch of the segmental vessels from the aorta and iliac arteries, specifically the intervertebral segment of the spinal ramus of the posterior segmental (intercostal) arteries (see Fig. 397-1).
The largest and most important of these 6 to 10 medullary arteries is the arteria radicularis magna, or the artery of Adamkiewicz. It serves as the major blood supply to the mid and lower thoracic and lumbar segments of the spinal cord and typically originates on the left side between T8 and L2; however, it may arise from T3 to L4 and may be on either side.37 The upper thoracic portion of the spinal cord, above the region perfused by the artery of Adamkiewicz and below the relatively well collateralized cervical region, is a “watershed” or arterial border zone. It is particularly susceptible to hemodynamic ischemia and is the site of watershed infarcts of the spinal cord induced by severe hypotension.
In addition to the medullary arteries, which supply only the spinal cord, there are two other important terminal branches of the intervertebral artery (the posterior spinal ramus of each segmental artery): the radicular arteries (which supply the nerve roots) and the dural arteries (which supply the dural root sleeves and spinal dura). These arteries persist at each segmental level into adulthood (see Fig. 397-1).
Venous Anatomy
The spinal cord venous system, like the arterial system, is composed of two radially arranged vascular networks (see Fig. 397-1). The sulcal veins in the anterior median fissure and the radial veins in the dorsal and anterolateral portions of the spinal cord drain to the coronal venous plexus on the cord surface.38 This pial venous plexus is drained by medullary veins to the epidural venous plexus. The medullary veins—which like the medullary arteries are not present at each segmental level but arise sporadically along the long axis of the spinal cord—cross the subarachnoid space and penetrate the dura adjacent to the dural penetration of the nerve roots.38 Venous structures within the intrathecal space lack valves, but functional valves at the level of the dural penetration of the medullary veins prevent retrograde venous flow from the epidural (Batson’s) plexus to the intradural space.35,38 In the region of the cervicomedullary junction, the venous system of the brainstem and the spinal cord communicate freely.
Vascular Anatomy of Spinal Vascular Abnormalities
Dural Arteriovenous Fistulas
Figures 397-2 and 397-8 illustrate the typical pathologic and arteriographic anatomy of spinal dural AVFs. The AVF, a low-flow shunt in the dural sleeve of a spinal nerve root, is supplied by the dural branch of the intervertebral artery. A medullary vein, the sole venous outflow from the dural AVF, carries the shunted arterial blood retrograde to the normal direction of venous flow to the coronal venous plexus.2 Microarteriography of specimens in which the spinal dural AVF was excised en bloc demonstrates that the nidus in the dura is in the dural root sleeve and the adjacent spinal dura and that it is a simple network of a small number of separate vessels that converge into the medullary vein just beneath the inner layer of dura (Fig. 397-9).39
The absence of other routes of regional venous drainage,15,40 normally provided by the medullary veins, to carry excess blood flow from the coronal venous plexus through the dura and into the extradural venous system results in rostral flow of the arterialized blood in the coronal venous plexus, which reaches the cranial venous system in most patients. The diversion of blood under high pressure by the arterialized medullary vein from the dural AVF into the coronal venous plexus, combined with the absence of normal pathways for venous drainage, results in dilation, elongation, and tortuosity of the vessels of the coronal venous plexus. Moreover, because the intrathecal venous system is valveless, the radial and sulcal veins transmit the high pressure directly to the spinal cord, thereby producing venous congestion, venous hypertension, reduced arterial perfusion pressure, ischemia, and myelopathy (see later).
Intradural Arteriovenous Malformations
The nidus of intradural AVMs (Figs. 397-10 and 397-11; see also Figs. 397-3 and 397-4) is embedded within the spinal cord or is partially intramedullary and extramedullary. Intradural AVMs are more uniformly distributed along the length of the spinal cord than are dural or perimedullary AVFs (see Fig. 397-7). They are usually supplied by one or more enlarged medullary arteries that supply the spinal cord as well.
Juvenile Type
The anatomic features of juvenile-type intradural AVMs are represented in Figures 397-3 and 397-10. These lesions are fed by multiple enlarged medullary arteries via the anterior and posterolateral spinal arteries and may have a voluminous arteriovenous nidus that completely fills the thecal sac. The nidus typically has neural tissue within its interstices. Juvenile AVMs frequently involve the vertebral column and the paraspinous soft tissues (metameric form; see Fig. 397-10). Unlike dural AVFs, these lesions are high-flow AVMs; a spinal bruit may indicate their presence.
Glomus Type
The nidus in the glomus subtype of intradural AVMs is a compact tangle of blood vessels that is confined to a short segment of cord (see Figs. 397-4 and 397-11). These lesions typically lie in the anterior half of the spinal cord and are supplied by one or two medullary arteries via the anterior spinal artery or the posterolateral spinal arteries.
Perimedullary Arteriovenous Fistulas
The anatomic derangement in intradural AVFs is depicted in Figures 397-5, 397-12, and 397-14. In these lesions, a direct pial fistulous communication lies between an intradural spinal artery and the coronal venous plexus. The absence of intervening small vessels (i.e., a glomus) at the AVF defines the lesion. The fistula may arise from the anterior or posterolateral spinal arteries but is more commonly supplied by the anterior spinal artery.14,16,32,41 Intradural AVFs are often associated with a venous varix at the arterial-to-venous transition.
Cavernous Angiomas
Because cavernous angiomas are usually angiographically occult, it is not surprising that the spinal cord vascular anatomy is not altered by them (see Fig. 397-6). These mulberry-like lesions are typically small (5 to 15 mm), have a low level of blood flow, and are supplied by delicate thin-walled vessels. A rim of hemosiderin and gliosis, the product of previous small hemorrhages, typically surrounds these well-demarcated lesions. Cavernous malformations may occur in association with cerebral cavernous malformations and may occur at more than one level of the spinal cord when they are associated with familial multiple cavernous angiomatosis,42,43 which is now known to be an autosomal dominant trait that maps to chromosome 7q.44–50
Etiology, Pathophysiology, Clinical Findings, and Natural History
Dural Arteriovenous Fistulas
Spinal dural AVFs are distinguished from intradural AVMs by their cause, pathophysiology, incidence, age at onset of symptoms, distribution along the spinal axis (see Fig. 397-7), and neurological findings and progression (Table 397-2).5,15 These distinguishing characteristics are important because effective treatment of such lesions requires early recognition and clinical differentiation from intradural spinal AVMs.
TABLE 397-2 Comparison of Clinical Syndromes of Dural Arteriovenous Fistulas and Intradural Arteriovenous Malformations
DURAL AVF (N = 27) | INTRADURAL AVM* (N = 54) | |
---|---|---|
Gender | Predominantly male | Male or female |
Mean age at diagnosis (yr) | 46 | 24 |
Onset of symptoms | Gradual (85%) | Acute (37%) |
SAH | 0 | 50% |
First symptom | Paresis (44%) | SAH (32%) |
Spinal bruit | 0 | 6% |
Exacerbation of symptoms by activity | 70% | 15% |
Arms affected | 0 | 11% |
AVF, arteriovenous fistula; AVM, arteriovenous malformation; SAH, subarachnoid hemorrhage.
* Includes perimedullary AVFs.
Adapted from Rosenblum B, Oldfield EH, Doppman JL, et al. Spinal arteriovenous malformations: a comparison of dural arteriovenous fistulas and intradural AVMs in 81 patients. J Neurosurg. 1987;67:795-802.
Although the exact mechanism underlying the origin of dural AVFs is unknown, the late onset of symptoms, lack of association with other vascular anomalies, strong predilection for the lower spinal segments, absence of medullary veins in these patients,15,40,51 predominant occurrence in men, and occasional development of similar lesions by acquired means, such as traumatic paraspinal AVFs52 and postoperative dural AVFs,53 suggest that these lesions are acquired.5,15 The pathophysiology of the progressive myelopathy produced by these lesions is clear: dural AVFs are low-flow arteriovenous shunts that induce venous hypertension. By directly measuring venous pressure in the coronal venous plexus at surgery in patients with spinal dural AVFs, Hassler and coworkers demonstrated that venous pressure in the spinal cord averages 74% of the simultaneous mean systemic arterial pressure.54 Therefore, by the time of treatment, these patients have venous hypertension severe enough to reduce spinal cord arterial perfusion pressure to less than 30% of normal (spinal cord perfusion pressure = mean arterial pressure−venous pressure), thereby resulting in venous congestion, spinal cord ischemia, and progressive cord injury with clinical myelopathy. The clinical result of progressive cord injury from venous hypertension is an insidious but progressive decline in motor and sensory function, which is the manifestation in 85% to 95% of patients with dural AVFs.15,55 However, about 5% to 15% of patients with dural AVFs experience episodes of acute myelopathic exacerbation (Foix-Alajouanine syndrome).17 The rapid worsening in such cases probably indicates profound venous congestion, which unless treated expeditiously to eliminate venous hypertension, will result in venous thrombosis and irreversible cord injury.56 An even rarer finding—and one that has been reported in only a few patients with dural AVFs—is acute hemorrhage.
For clinical diagnosis, it is important to remember that dural AVFs are distinguished from intradural AVMs by a number of clinical features (see Fig. 397-7 and Table 397-2).16 Unlike intradural AVMs, dural AVFs have a strong male predilection (>80%) and develop in the latter half of life (in 80% of patients the onset of symptoms begins after the age of 40).15 Dural AVFs have a strong tendency to occur in the lower thoracic and lumbar regions.15 Consequently, patients with spinal dural AVFs are unlikely to exhibit upper extremity involvement and typically have an insidious onset of paraparesis or sphincter dysfunction. Low back or radicular pain often precedes the onset of a gradually progressive myelopathy. Patients with dural AVFs frequently report worsening of symptoms during physical exertion (neurogenic claudication) or with certain changes in posture (see Table 397-2).15
There are no studies of the natural history of patients with untreated dural AVFs. The first report describing them was not published until 1977.13 With that report it became apparent that treatment was simple, safe, and effective. Thus, a prospective study of their natural history without treatment has not been possible and cannot be justified now or in the future. However, the natural history of dural AVFs can be deduced from studies performed before recognition of their existence and from studies of the condition of patients at treatment.57 This opportunity is based on their high prevalence in patients with spinal AVMs; their distinguishing epidemiologic and clinical features,5,15 which are distinct enough to permit their identification with reasonable accuracy in previous studies30,58–60; and the consistency of their clinical course. The information available to examine the natural history comes principally from a retrospective analysis performed by Aminoff and Logue and reported in 1974.58,59 Although their study began before the introduction of selective spinal arteriography, it clearly reveals the natural history of patients with dural AVFs. The study was composed predominantly of adult men with thoracolumbar lesions (49 of the 60 patients were ≥41 years old, 43 of those 49 were men, and 55 of the 60 lesions [92%] were located in the thoracic or lumbar spinal segments)43 that had never hemorrhaged (90%), features that distinguish patients with dural AVFs from those with other types of spinal AVMs.57 The course of the disease, as defined by their study, consisted of progressive neurological decline and functional disability. Twenty percent of the 60 patients required crutches or were nonambulatory by 6 months after the onset of symptoms other than pain (Fig. 397-13). Fifty percent of the patients were severely disabled (confined to a wheelchair or bed) within 3 years of the onset of gait impairment, and 91% had restricted activity within 3 years of the onset of symptoms.58,59 Similarly, in their 1976 report, which included 23 patients with type I AVMs (now known to be dural AVFs), Tobin and Layton noted that “There seems to be a distinct natural history for patients who have the angiographic Type I arteriovenous malformation. These usually have a slowly progressive course, evolving over 2 to 3 years, leading to nearly complete paraplegia and bowel or bladder incontinence. Few of these patients seem to have other modes of presentation.”29
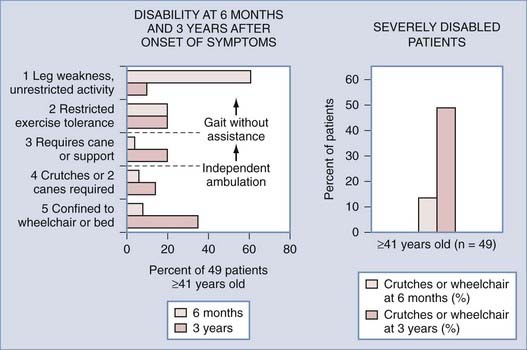
FIGURE 397-13 Degree of functional disability at 6 months and 3 years after the onset of symptoms in 49 patients aged 41 years or older reported by Aminoff and Logue.59 Almost all these patients had spinal dural arteriovenous fistulas. Note that the greatest change in function between 6 months and 3 years is in patients with minimal neurological deficit who progressed to severe functional disability. By 3 years, half the patients were confined to wheelchairs or had to use crutches to ambulate.
(From Oldfield E. Spinal vascular malformations. In: Swash M, ed. Outcomes in Neurological and Neurosurgical Disorders. Cambridge, UK: Cambridge University Press; 1998.)
In contrast to the gradual, slowly progressive decline in motor and sensory function over a period of 2 to 3 years,5,13,15,29,55,59,61,62 about 15% of patients with dural AVFs have more rapid, subacute neurological worsening (Foix-Alajouanine syndrome). This deterioration is unpredictable and indicates severe venous congestion, which unless treated immediately will lead to venous thrombosis, infarction, and irreversible loss of neurological function.17,56
Intradural Arteriovenous Malformations
Spinal cord AVMs, unlike dural AVFs, occur in males and females at a nearly equal incidence (see Fig. 397-7). Furthermore, intradural vascular malformations of the spinal cord have an earlier onset of symptoms, a higher incidence of association with other vascular anomalies, and a more uniform distribution along the spinal axis than do dural AVFs (see Fig. 397-7).15 Collectively, these observations suggest that AVMs of the spinal cord are congenital lesions, most likely the result of inborn errors of vascular embryogenesis (Table 397-3). The embryologic insult probably occurs before day 18 of gestation because the spinal cord vasculature is fully developed by that time.
TABLE 397-3 Comparison of Acquired versus Congenital Vascular Malformations
ACQUIRED DURAL AVF | CONGENITAL AVM OF THE SPINAL CORD | |
---|---|---|
Gender | Predominantly male | Male or female |
Onset of symptoms | Later half of adulthood | Child or young adult |
Associated congenital malformation | Never | Occasionally |
Distribution along the spine | Lower half | Diffuse |
Normal spinal venous pathways | Absent | Present |
AVF, arteriovenous fistula; AVM, arteriovenous malformation.
From Oldfield E, Doppman J. Spinal arteriovenous malformations. Clin Neurosurg. 1988;34:161-183.
Although current understanding of the pathophysiologic basis of the myelopathy in medullary AVMs (juvenile and glomus types) suggests that the mechanism may not be the same in all patients, the high flow rates associated with this type of spinal AVM underlie all putative mechanisms. In contrast to patients with dural AVFs, an acute initial manifestation consisting of the sudden onset of back pain, suboccipital pain, and meningismus or sudden loss of consciousness occurs in many patients with AVMs of the spinal cord; subarachnoid or intramedullary hemorrhage occurs as the initial finding in about 35% of patients; and by the time of diagnosis, about 50% of patients have had one or more hemorrhages (Table 397-4; see also Table 397-2).15,60,63–65 The incidence of hemorrhage is even higher in children. In 38 children younger than 15 years with AVMs of the spinal cord reported by Riche and colleagues, 84% had an acute initial onset of symptoms, 59% of which were associated with sudden impairment of motor function.18 The high incidence of associated arterial aneurysms and the 13% to 37% incidence of associated vascular abormalities elsewhere in the central nervous system15,60,63 may partially explain the high incidence of hemorrhage.
Conversely, about half of all patients with intramedullary AVMs do not experience the acute deterioration that accompanies hemorrhage. The gradual loss of neurological function in these patients suggests a different mechanism of cord injury.15 Several mechanisms for this progressive form of myelopathy have been proposed, including ischemia resulting from vascular steal,66,67 mechanical compression by an aneurysm, and medullary venous congestion.15 Because intradural AVMs are high-flow shunts, because the medullary arteries that supply the arteriovenous nidus of these lesions also routinely supply the spinal cord, and because visible, often enlarged medullary veins provide venous drainage into the extradural venous system in most of these lesions,15 a vascular steal phenomenon may be the most logical explanation in many patients with progressive loss of neurological function.
The natural history of intradural AVMs, similar to the pathophysiologic mechanism of cord injury, is incompletely defined and variable. Data on long-term disability without therapy are unavailable because these lesions are usually treated when they are diagnosed. Many reports suggest that AVMs of the spinal cord cause recurrent hemorrhages or progressive neurological disability over the first few months or years after they are diagnosed (see Table 397-4). In the 90 patients with intradural AVMs of all types reported by Hurth and associates, 69% had acute episodes of stepwise neurological progression, and 39% of the patients with previous hemorrhage had at least one additional hemorrhage (fatal in one patient).60 Similarly, in the 38 patients with thoracic medullary AVMs treated by Biondi and colleagues by repeated, yearly embolization with particles of polyvinyl alcohol (PVA), 31% relapsed with neurological worsening between treatments (average of 6 years of treatment).64 Pregnancy, vigorous exertion, and minor trauma may cause rapid progression of symptoms or be associated with increased risk for hemorrhage (see Table 397-4).
Most authors characterize the prognosis of these patients as grave and consider the natural history sufficiently unpredictable and hazardous to justify the risks related to treatment.5,15,18,41,60,64,65,68 However, it must be acknowledged that the natural history varies greatly in individual patients and that the prognosis in some of them is not always grim. Retrospective evaluation of Aminoff and Logue’s series57 indicates that in half the patients whose manifestation was acute—one more likely to be associated with intradural AVMs—there was no subsequent neurological progression.58,59 Similar observations were made by Tobin and Layton in their report on the natural history of patients with spinal AVMs, in which they reported a progressive course leading to paraplegia over a period of 2 to 3 years in patients with features that now suggest dural AVFs, but “such a relationship was not as clear” for intradural AVMs.29 Hurth and associates noted that 80% of the 17 untreated patients with AVMs in the cervical segments of the spinal cord were independent and capable of carrying out their jobs 5 years after diagnosis and that 5 patients who were untreated or incompletely treated were unchanged 15 years after diagnosis, thus demonstrating the “slow progression of disease … in a limited number of cases.” Furthermore, 60% of their 17 untreated patients with thoracic AVMs at 5 years and 41% of 17 untreated or incompletely treated patients at 15 years were “self-sufficient and relatively well.”60 The dilemma in making decisions for individual patients is that we have no reliable guidelines that permit us to identify these patients prospectively.
An exception to this occurs in children with symptomatic AVMs of the spinal cord. In the retrospective assessment of 38 children younger than 15 years by Riche and colleagues, symptoms had a sudden onset in 84%, and in 60% of these patients there was sudden impairment of motor function, often associated with physical effort.18 The sudden onset was commonly followed by a period of remission, but with successive attacks in 71% and subarachnoid hemorrhage in 55%. Unlike the situation in adults, a gradually progressive evolution of the syndrome occurred in just 17% of the 38 children.
Perimedullary Arteriovenous Fistulas
Perimedullary AVFs, which lie in the pia on the surface of the spinal cord, constitute about 10% to 20% of all spinal AVMs.14,15,30 They are distributed equally between males and females, and most are associated with an onset of symptoms in the first half of adult life; the average age at diagnosis is older than that of patients with AVMs of the spinal cord but younger than that of patients with dural AVFs (see Fig. 397-7). However, they may also be diagnosed in childhood as early as 3 weeks of age.69,70 Their anatomic distribution along the spinal axis is bimodal, predominantly in the thoracolumbar region, particularly at the conus medullaris, and to a lesser extent in the upper cervical region (see Fig. 397-7).14,15,30,33,69
The existence of these lesions in early childhood suggests a congenital mechanism of origin. However, they may initially be diagnosed in late adulthood, and there are cases in which a perimedullary AVF was acquired. In one instance, an AVF arose in the conus medullaris in a 28-year-old 5 years after resection of a teratoma of the filum terminale.41 In another case, a perimedullary AVF in the conus medullaris developed in a 35-year-old 1 year after resection of an ependymoma at the same site.69 In two patients the origin of an AVF in late adulthood is argued to have occurred in association with deficient medullary venous drainage of the spinal cord.34
The initial clinical finding is a slowly progressive myelopathy or radiculopathy, which occurs in about 80% of patients, or subarachnoid hemorrhage, which affects about 20% of patients.15 For therapeutic purposes, Merland and colleagues categorized perimedullary AVFs into three distinct types (Table 397-5). Type I is a small, simple fistula supplied by a single feeder, usually the terminal portion of a thin anterior spinal artery, but in some instances a posterior spinal artery. Flow through the fistula is usually slow and ascending in the vessels of the coronal venous plexus, which are only slightly tortuous and dilated (Fig. 397-14). Type II AVFs are supplied by one or two main arterial feeders via several distinct arterial pedicles that converge to form multiple discrete shunts and drain into a dilated and tortuous venous system at a relatively high flow rate (see Fig. 397-12). Type III AVFs, which account for the majority of pial AVFs, are single giant AVFs with very high blood flow located in the cervical or lower thoracolumbar level. They are fed by several branches of the posterior or anterior spinal arteries, which are hugely dilated and converge into a single shunt draining into a giant venous ectasia (Fig. 397-15).30,41
Initially, it appeared as though there were no distinctive clinical features among the various types of perimedullary AVFs, but with more experience it has become apparent that only type II and III AVFs hemorrhage or produce compression of the spinal cord by venous ectasia.30 Nonetheless, most patients have a gradually progressive neurological loss15,30 that is similar to the progression associated with the venous hypertension underlying the myelopathy of spinal dural AVFs. Barrow and colleagues directly quantified venous hypertension in the venous drainage of two patients with intradural AVFs and progressive myelopathy and related the venous pressures with the clinical and MRI findings.69 This mechanism probably accounts for the myelopathy in most patients with type I AVFs because they do not hemorrhage, they are not associated with venous ectasia and cord compression, and the rate of transit from the arterial to the venous system is much too slow to produce vascular steal. However, because type II and III AVFs are always supplied by an artery that also supplies the spinal cord, it is likely that vascular steal accounts for the myelopathy in at least some of these patients with rapid shunting of blood and generous venous drainage pathways on arteriography.
Retrospective studies of the clinical history before treatment provide the only information on the natural history of this type of AVM. They suggest a relentless progression from myelopathy to paraplegia within 5 to 7 years of the onset of symptoms in patients with myelopathy but without hemorrhage, and there is a high incidence of repeated hemorrhage in patients with hemorrhage.14,15,41,30,69
Cavernous Angiomas
Cavernous angiomas, also known as cavernous malformations, cavernous hemangiomas, and cavernomas, are distributed along the entire length of the neuraxis and represent 5% to 12% of all spinal vascular abnormalities.44,71 They are distributed proportional to the volume of nervous tissue, so many more arise in the brain than in the spinal cord.72 Cavernous angiomas in the spinal cord are histologically identical to those in the brain. They typically occur as an intramedullary mass but may be seen as an epidural lesion.
Studies have identified a familial variant of the disease (familial multiple cavernous angiomatosis) characterized by multiple cavernomas and autosomal dominant transmission.42,43,73–78 Genetic studies on patients from affected families have localized the genetic abnormality to chromosome 7q.45–50,78–84 Although cavernomas are associated with familial transmission, they do not necessarily arise early in life. They have been documented to develop de novo (with and without a family history) in the brain and spinal cord as new lesions in patients with previous negative CT and MRI scans and after irradiation of the cranial and spinal axes in adults and children.85–88
Although cavernous angiomas are low-flow lesions, the pathogenesis of the myelopathy is usually via hematomyelia. Symptomatic lesions have a hemosiderin ring on MRI and are seen to have a hemosiderin-stained gliotic capsule at surgery, thus suggesting repeated small hemorrhages (Fig. 397-16). Small lesions may remain clinically silent throughout life, only to be discovered at postmortem examination. Symptomatic cavernous angiomas tend to arise in early adulthood through middle age (third to sixth decades).72
The clinical manifestations vary greatly among patients, but there are four common patterns: discrete acute episodic progression over a period of months or years, slowly progressive decline of neurological function over a period of months or years, acute onset and rapid progression, and slowly progressive loss of function after an acute onset of mild neurological symptoms.89 Cavernomas in the spinal cord, like those in the brainstem, are more commonly symptomatic than those in the cerebral hemispheres. The information available on the natural history of spinal cord cavernous angiomas is limited. The French Study Group of Spinal Cord Cavernomas recently reported the outcomes of 53 patients harboring spinal cord cavernomas, including 13 patients who did not undergo surgery.90 The average follow-up was 7.3 years. Of the 13 patients who did not undergo surgery, 4 experienced relapse of symptoms during the follow-up period. However, in one retrospective series of 67 consecutive patients, 27 of whom underwent surgery for symptom-producing lesions, 42 patients with less severe neurological findings (most of whom had minor nonprogressive neurological deficts, including pain, mild weakness, or myelopathy) did not undergo surgery and were monitored expectantly. In the 33 patients who were monitored for an average of 9.7 years (319 patient-years), there were five symptomatic hemorrhages (1.6% per patient per year; mean time to a new event, 2.7 ± 1.2 years). None of the 33 patients experienced clinically significant neurological deficits as a result of recurrent hemorrhage.91 In another study, none of the 10 patients with symptomatic cavernous angiomas of the spinal cord who were managed without surgery with a mean follow-up of 6.7 years had an acute intramedullary hemorrhage.92 In 9 of these 10 patients, neurological function at last evaluation was the same or better than their function at initial evaluation. The results of these studies coincide with the experience of one of us (E.H.O.), but surgical selection bias (100% of patients in the surgical group had motor symptoms as opposed to 45% in the conservatively managed group at initial evaluation) also has to be considered. Thus, recommendations for treatment must consider the patient’s symptoms, evidence of progression, and the projected risks associated with surgery.
Diagnostic Imaging
Although dural AVFs are distinguished clinically from intradural AVMs by the age at onset of symptoms, distribution along the spinal axis, and neurological findings,15 the specific diagnosis must be confirmed with imaging studies. Clinical features are useful only insofar as they guide the clinician through the proper diagnostic tests toward an accurate diagnosis and appropriate treatment. The imaging evaluation ultimately determines the proper intervention because effective management is predicated on establishing the precise type of vascular abnormality and the specific anatomy of the lesion.
Screening Studies
Initial screening examinations with plain films of the spine are usually helpful only to rule out other causes of radiculomyelopathy, such as spondylosis, vertebral tumor, or fracture. Occasionally, high-flow AVMs cause an increased interpedicular distance at the level of the AVM (see Fig. 397-15), but there are no specific findings of spinal AVMs on plain films.
Magnetic Resonance Imaging
Because of its lower risk and capacity for multiplanar imaging, MRI has replaced myelography as the initial diagnostic procedure in patients with myelopathy. With spinal AVMs, MRI abnormalities may be produced by abnormal vessels in the subarachnoid space, by the nidus of an intramedullary AVM in the spinal cord, or by changes in the spinal cord produced by venous congestion, myelomalacia, infarction, or hemorrhage.19,93–97 MRI is noninvasive and often provides the initial diagnosis of an AVM; it may also differentiate intramedullary AVMs from perimedullary AVFs and dural AVFs. Ideally, MRI should be performed with at least a 1.5-T machine using a spinal coil.
Patients with spinal vascular malformations, except those with cavernous angiomas, often demonstrate a serpentine pattern of low signal in the subarachnoid space on T1- and T2-weighted MRI. This pattern of signal void is due to flow in the dilated, tortuous vessels of the arterialized coronal venous plexus. These arterialized pial veins may focally indent the cord surface and produce a scalloped appearance on sagittal T1-weighted images (Fig. 397-17B and C). The abnormal signal produced by the enlarged coronal venous plexus is frequently most prominent in the posterior pia and subarachnoid space.
Magnetic resonance angiography (MRA) has become increasingly useful in the evaluation of patients suspected of harboring a spinal vascular malformation.98,99 MRA techniques, including time-resolved spinal MRA and contrast-enhanced MRA, have evolved to the extent that it can be used to detect spinal vascular malformations and to define them in some patients (or at a minimum provide the information to focus subsequent spinal digital subtraction arteriography [DSA] when it is performed). However, it should be emphasized that MRA should not supplant dedicated spinal catheter DSA because catheter arteriography remains the “gold standard” for definitively categorizing a spinal vascular malformation and precisely defining its anatomy.
Dural Arteriovenous Fistulas
Patients with dural AVFs have a wide range of findings on MRI.19,94 T1- and T2-weighted images, when abnormal, often reveal an area of focal cord expansion; there is a central area of low or high signal on T1-weighted images and increased signal intensity on T2-weighted images in the thoracolumbar segments of a cord swollen from venous congestion (see Fig. 397-17A and B). In the large study of Gilbertson and coworkers, increased T2 signal in the spinal cord was the most common finding, and it occurred in all patients.100 Studies performed immediately after the intravenous injection of gadolinium–diethylenetriaminepentaacetic acid (DTPA) reveal enhancement of the vessels of the coronal venous plexus (see Fig. 397-17C) and may produce enhancement of the most severely involved segments of the spinal cord; delayed imaging after gadolinium (40 to 45 minutes) may reveal an increase in the extent of enhancement to involve the entire enlarged lower portion of the spinal cord (Fig. 397-18).97 Whether gadolinium enhancement of the spinal cord indicates edema, venous congestion, or loss of integrity of the blood-brain barrier associated with ischemic injury remains to be determined. Although the sensitivity of T2 signal changes is high, T2 signal abnormality and enhancement of the spinal cord on T1-weighted images are nonspecific features, and some patients do not have visible contrast enhancement of the congested vessels of the coronal venous plexus (see Fig. 397-18). It has recently been suggested that peripheral hypointensity on T2-weighted images of the spinal cord is a consistent and specific diagnostic feature of venous congestion associated with dural AVFs.101
Contemporary spinal MRA can not only demonstrate the presence of abnormal arterial feeders or draining veins (or both) but can also often depict the site of the fistula and the location of the shunt within one vertebral level of where it is found with spinal DSA.98,99
Surgical elimination of the spinal dural AVF results in diminishment of the swollen appearance of the spinal cord, induces resolution of the abnormal T2 hyperintensity in the spinal cord, and eliminates enhancement of the abnormal vessels of the coronal venous plexus over the weeks or months after surgery (see Fig. 397-17).98,99,102,103
Intradural Arteriovenous Malformations and Arteriovenous Fistulas
At the site of intramedullary AVMs, T1-weighted images usually demonstrate a focal signal flow void comprising multiple channels with a serpentine pattern in an area of local expansion of the spinal cord (Fig. 397-19).94,95 The position of the nidus in the axial plane of the cord is established with sagittal and axial images, which sometimes permit the exact intramedullary position of an AVM to be recognized and occasionally distinguish perimedullary AVFs from intramedullary AVMs that are partially intramedullary and extramedullary.94,95 Subacute hemorrhage is seen as increased signal on T1-weighted studies. An aneurysm or a venous varix associated with an AVM or an AVF, respectively, is recognized as a globular-shaped region of flow void (see Fig. 397-15C and D).93,94,104 Spinal MRA can identify the presence of an intradural AVF or AVM, but classification into the correct subtype can be difficult. Occlusion of the nidus with emboli or thrombosis of a varix after interruption of an AVF can be confirmed by loss of the signal flow void on T1-weighted images,94 as well as by spinal MRA.
Although MRI may suggest a spinal vascular malformation and the need for further spinal arteriographic evaluation, if MRI is performed only without contrast enhancement, the images may either be normal or show nonspecific changes in patients with dural AVFs (see Fig. 397-18). Because dural AVFs are the most common type of spinal vascular malformation and the type most amenable to treatment, patients with unexplained progressive myelopathy or radiculopathy and a normal unenhanced MRI study require additional diagnostic evaluation with contrast-enhanced MRI, spinal MRA, or both. In cases in which MRI is negative or equivocal and clinical suspicion remains, spinal DSA should still be performed.
Cavernous Angiomas
The results of arteriography and myelography are usually normal, so MRI is the most important diagnostic test for detection of cavernous angiomas.44,71 MRI typically demonstrates a well-delineated, predominantly low-signal spherical or oblong intramedullary lesion with scattered heterogeneous areas of increased signal (see Fig. 397-16). The decreased signal is due to deposition of hemosiderin along the periphery of and within the malformation. However, the absence of a true “flow void” signal is consistent with these low-flow lesions. On T2-weighted images, this mix of signal intensity produces the familiar “target” configuration that is typical of cavernous malformations (see Fig. 397-16A).
Computed Tomographic Angiography
Computed tomographic angiography (CTA) may also be used as a screening test in patients suspected of having a spinal vascular malformation. With 4- and 16-detector and, more recently, 64-detector spiral CT imaging, CTA has been used to successfully detect spinal vascular malformations.20,105 CTA generally clearly demonstrates the draining veins and usually depicts the feeding vessels and the fistula or fistulas.20,105 CT scanning, however, is poor for examining the spinal cord parenchyma itself. As with spinal MRA, CTA can demonstrate the presence of the fistula and often delineates the anatomy of the fistula but cannot supplant spinal DSA. CTA is useful in helping focus DSA, thus reducing the time needed for the study and the amount of contrast material required.
Myelography
It is important to remember that myelography is a sensitive diagnostic screening test for spinal AVMs. A technically acceptable myelogram reliably demonstrates abnormal vascularity as serpentine filling voids in the subarachnoid space in all types of spinal vascular abnormalities (Fig. 397-20) except cavernous angiomas. Therefore, in a patient with equivocal findings on MRI, myelography that fails to demonstrate abnormal vessels in the subarachnoid space obviates the need for spinal arteriography. However, once the diagnosis of a spinal AVM has been confirmed with MRI or myelography, spinal arteriography is necessary to establish the type of spinal AVM, define the precise anatomic location of the nidus of the AVM, and identify the arterial supply to the AVM and to the spinal cord.
Selective Spinal Arteriography
Because successful treatment of spinal AVMs requires detailed understanding of the regional vascular anatomy, spinal arteriography is indispensable. It provides precise anatomic information about four items that are crucial to the surgeon or interventional neuroradiologist: the type of vascular malformation, the exact location and anatomic configuration of the fistula or nidus of the vascular malformation, identification of the feeding vessels to the malformation, and delineation of the regional vascular anatomy of the spinal cord. Table 397-6 summarizes the major features distinguishing dural AVFs from intradural AVMs of the spinal cord and perimedullary AVFs.
TABLE 397-6 Radiographic Features Distinguishing Dural and Intradural Malformations
DURAL AVM (N = 27) | INTRADURAL AVM* (N = 54) | |
---|---|---|
Site of nidus | 100% in the lateral canal | 80% within the cord |
11% on the ventral cord surface | ||
9% on the dorsal cord surface | ||
Level of spine | Lower half | Diffuse |
Rapid flow | 0 | 80% |
Associated spinal aneurysm | 0 | 44% |
Common supply of the AVM and medullary artery | 15% | 100% |
Route of venous drainage | 100% rostral | 81% rostral |
4% caudal | 72% caudal |
AVF, arteriovenous fistula; AVM, arteriovenous malformation.
* Includes perimedullary AVFs.
Adapted from Rosenblum B, Oldfield EH, Doppman JL, et al. Spinal arteriovenous malformations: a comparison of dural arteriovenous fistulas and intradural AVMs in 81 patients. J Neurosurg. 1987;67:795-802.
Dural Arteriovenous Fistulas
Selective DSA of dural AVFs demonstrates intrathecal drainage of the fistula, via an arterialized medullary vein, into the dilated, tortuous veins of the coronal venous plexus (see Fig. 397-8); in most patients this occurs predominantly along the posterior surface of the spinal cord. In some patients it also discloses the nidus of the AVF in the intervertebral foramen and lateral aspect of the spinal canal (see Fig. 397-8A). A prolonged view of the venous phase after selective injection of the artery feeding the AVF fully defines the abnormal venous anatomy because these low-flow lesions often require 15 seconds or more for the contrast material to dissipate.
Intracranial dural AVFs that drain inferiorly into the spinal venous system and cause myelopathy can mimic spinal dural AVFs clinically and on MRI and myelographic studies (Fig. 397-21).21,22,106 The AVF in these patients is located in the dura of the posterior fossa convexity or the tentorium and is supplied by branches of the internal or external carotid or vertebral/basilar arteries. Myelopathy occurs when the arterialized venous drainage has access to the valveless spinal venous system. Hence, if a patient with myelopathy has MRI or myelographic findings of a dilated, tortuous coronal venous plexus but normal spinal arteriography, the patient should undergo cerebral arteriography to search for a dural AVF of the rostral portion of the cervical segments of the spinal cord, the tentorium, or the dura of the posterior fossa. In this circumstance, bilateral selective vertebral arteriography is important because unilateral injection with retrograde flow down the opposite vertebral artery will not detect some cervical spinal dural AVFs.107 Similarly, because sacral dural AVFs with intrathecal medullary venous drainage and consequent myelopathy may be supplied by branches of the iliac arteries,23,108 it is important for the arteriogram to include selective injection of all arteries potentially supplying the sacral dura if selective spinal arteriography is negative. Finally, occasionally in patients with atherosclerosis the origin of the feeding vessel is occluded and selective spinal arteriography is negative; in such patients, CTA or MRA may help localize the site of the dural AVF, or surgical exploration focused on the results of spinal MRI and knowledge that the medullary vein draining the dural AVF enters the subdural space contiguous with the dural penetration of a nerve root, usually in the lower thoracic region, can be used to locate and treat the AVF.109
Intradural Arteriovenous Malformations
Selective spinal arteriography of glomus-type AVMs typically demonstrates a dense mass of blood vessels confined to a short segment of the spinal cord. These lesions may be supplied solely by the anterior spinal artery (see Fig. 397-11) or by the anterior and posterior spinal arteries.
Juvenile-type spinal AVMs have multiple feeding vessels and occupy a larger volume of the spinal cord. The nidus is usually large and contains spinal cord tissue within its interstices. In the metameric form, paraspinous soft tissues may also be involved (see Fig. 397-10).
Direct AVFs in the pia may be perfused by the anterior or posterior spinal arteries. These lesions are located on the cord surface or, rarely, on the filum terminale. The most common form, type III, is often associated with extensive venous enlargement and variceal formation. For the smaller, type I AVFs, the exact site of the fistula can often be determined as the point at which the feeding artery abruptly dilates as it joins the venous drainage (see Fig. 397-12). Angiomyelotomography is useful to detect small perimedullary AVFs (type I), to distinguish them from AVMs of the spinal cord, and to enhance precision of the definition of the vascular anatomy of the AVF before treatment.14,30,41
Treatment and Outcome
Dural Arteriovenous Fistulas
Surgery
The goal of treatment of spinal dural AVFs is permanent elimination of venous congestion of the spinal cord. This goal, which may be accomplished by excision, obliteration, or occlusion of the nidus or by interruption of venous drainage from the fistula between the dura and the dilated coronal venous plexus, has not always been the objective of treatment. When spinal arteriography was first introduced in the 1960s, the AVF was not identified in the spinal dura but was thought to reside in the arterialized pial veins of the coronal venous plexus. Consequently, these dilated, tortuous, arterialized coronal veins were mistaken for the nidus of the AVM and became the target of microneurosurgical operations designed to “strip” the cord of the purported pial nidus.12,68,110,111 Unfortunately, this treatment often exacerbated rather than eliminated the venous congestion. Furthermore, these stripping procedures involved an extensive multilevel laminectomy, tedious and unnecessary pial dissection, and a higher risk for morbidity in the patient. Moreover, the veins being excised were the normal veins of the coronal plexus that had been altered in appearance by the reception of blood under high pressure and flow.2 Recognition of the actual location of the dural AVF implied that effective treatment of most spinal vascular malformations should be directed at the dural nidus of the fistula. Simple interruption of the AVF produces permanent resolution of the venous congestion and improvement of myelopathy in many patients.5,13,15,21,55,62,112–114
Operative treatment of dural AVFs begins with a laminectomy one level above and one level below the dural nidus and the intramedullary vein that drains the fistula. One useful adjunct in localization of the point of fistualization is to place a coil in the feeding artery as close to the fistula as possible at the time of the spinal arteriogram. Fluoroscopy may then be used in the operating room to easily localize the fistula. After a midline dural opening, the arterialized medullary vein is identified. This vessel almost always penetrates the dura at the site of the dural penetration of the posterior nerve rootlets (Video 397-1; see also Fig. 397-2).
To confirm that the vessel to be interrupted is the medullary vein draining the dural AVF and not a medullary artery, its configuration is compared with the anatomy disclosed in the venous phase of the preoperative arteriogram, and it is followed as it crosses the subarachnoid space and joins the abnormal-appearing coronal venous plexus (Figs. 397-22 and 397-23). After identification and confirmation, the arterialized medullary vein is coagulated with bipolar forceps over a length of 4 to 6 mm and sharply divided as it penetrates the inner layer of dura between the dura and the engorged coronal venous plexus. Interruption of the medullary vein usually produces a visible change in venous turgor, and after a few minutes, the color of the arterialized coronal venous plexus may change from red to blue. However, it should be noted that interruption of the medullary vein generally does not produce an immediate or dramatic change in venous color (perhaps because of shunting of blood through a spinal cord capillary bed that is maximally dilated to supply the cord in the face of severely diminished perfusion pressure).
Special Circumstances
The only patients with persistence of a spinal dural AVF after surgical interruption of the vein draining it intradurally are those with more than one medullary vein draining the dural AVF intrathecally and those with extradural and intradural venous drainage of the fistula, a setting in which flow through the dural AVF remains patent.112 In this rare circumstance, the dural fistula should be excised or obliterated with bipolar cautery, but only if the segmental intercostal artery feeding the dural fistula is not also the origin of a medullary artery supplying the spinal cord.112 Because the artery of Adamkiewicz also arises from the same region as the spinal dural AVF in most patients, it is particularly important to note that 5% to 15% of dural AVFs are supplied by intercostal arteries that also supply the spinal cord via a medullary artery (see Fig. 397-23).15,115 When the same segmental vessel (intercostal artery or one of its equivalents) supplies the AVF and the spinal cord,115 simple intradural interruption of the arterialized medullary vein alone is the treatment of choice because it provides lasting obliteration of the fistula and is the only treatment that does not risk arterial occlusion and cord infarction (see Fig. 397-23).5,21,112
Outcome
Operative treatment of dural AVFs is safe and consistently interrupts the progression of neurological deficits.2,5,13,15,55,62,116,117 When the series from the National Hospital for Nervous Disease55 and the National Institutes of Health15 are combined (total of 76 patients), 37 of the 49 severely disabled (Aminoff and Logue grades 3 to 5) patients (76%) had improved gait after surgery (Fig. 397-24). Furthermore, 26 of 27 patients (96%) with disability but unaided locomotion retained independent ambulation, and 17 of the 27 (63%) improved. Of the 76 patients, 70 (92%) either stabilized or improved. Improvement in control of micturition and independent ambulation is achieved in most patients after surgery.15,55 Patients with more advanced preoperative disability are likely to be stabilized but are less likely to improve after surgery.2,13,15,55,57,62 These observations have been confirmed in more recent reports.113,114,116 One retrospective study of 25 patients found a tendency toward moderate, but definite functional decline several years after treatment.118 This occurred more commonly in patients with a more prolonged clinical course before treatment and in patients with more severe neurological deficits at treatment. Thus, this functional decline may represent the additive effects of age on the original neurological damage.
In summary, neurological outcome is closely related to the preoperative neurological function of the patient (see Fig. 397-24). With early diagnosis and treatment, before progression of the myelopathy and neurological impairment, patients retain their pretreatment neurological function or improve. Finally, because simple interruption of the venous drainage of a spinal dural AVF provides lasting occlusion of the fistula,112 as it does for cranial dural AVFs,108 this has been used to support the concept that the venous approach to the treatment of certain dural AVFs can be used successfully alone.119,120
Embolization
Although dural AVFs can be treated by transarterial embolization, the durability of embolic occlusion and neurological improvement has been problematic despite the promise offered by the introduction of new embolic agents and increased experience.117,121 Additionally, embolization cannot usually be safely performed in patients with a common arterial supply to the dural AVF and the spinal cord (see Figs. 397-14 and 397-24).5,15,115
With particulate embolization, the improvement in symptoms is generally only transient because the embolized dural AVF recanalizes117 and recurrent myelopathy follows.111,122 For spinal dural AVFs, particulate embolization is rarely indicated inasmuch as they are usually low-flow lesions and curative treatment is generally safe and straightforward.
Embolization with liquid polymerizing agents (N-butyl-2-cyanoacrylate [NBCA]) is used at some centers as the primary treatment of patients with spinal dural AVFs. It has been argued that embolization with liquid agents will pass distally into the feeding artery, reach the nidus of the dural AVF and the vein draining it, and provide permanent obliteration of the fistula. However, it is generally accepted that embolic occlusion with these agents does not consistently provide complete or permanent occlusion of other types of central nervous system AVFs and AVMs.123 Moreover, although minimal clinical or arteriographic follow-up has been performed in patients with spinal dural AVFs occluded with liquid agents, the incidence of recanalization is high, as is the frequency with which patients require additional therapy later.113,114,124 Merland and colleagues noted persistent flow through dural AVFs requiring immediate surgery in 14 of 45 patients who underwent embolization with liquid embolic agents.125 Niimi and associates examined the outcomes of 47 patients in whom embolic therapy was used for spinal dural AVFs.126 In 8 of the 47 (17%), the inadequacy of therapy was recognized immediately (partial embolization) because the acrylic did not reach the fistula or an anterior or posterior spinal artery arose at the same level. Two of these 8 patients were monitored for less than 1 month, but 5 of the 6 patients monitored for more than 1 month required additional treatment. Of the remaining 39 patients, 4 were observed for less than 1 month (in 1 of whom recanalization had already occurred), and 35 were monitored for at least 1 month, 18 of whom (51%) experienced recurrence. Overall, 26 of the 43 patients (60%) who were monitored for at least 1 month either had inadequate therapy at the outset (8 patients) or had a known recurrence of the AVF. Many of the patients who had not yet recanalized had been observed for less than 1 year,126 an interval known to be inadequate for establishing the permanency of occlusion.123
One critical issue alluded to earlier that affects durability may be attributed to inadequate penetration of the embolic agent to the site of the fistula and most proximal portion of the draining vein. Guillevin and colleagues reported a series of 26 patients with spinal dural AVFs treated with NBCA.127 With a mean follow-up of 38 months, when glue was found in the dura mater on CT scans (in 19 patients, 73%), none of these patients had a recanalized fistula on arteriography, and the clinical status of all patients improved. However, in 7 patients (27%), glue was observed in or proximal to the foramen on CT scans, and recurrence was high in this group (5 of the 7 patients had recurrence and required surgery).
A newer liquid embolic agent, Onyx (ethylene vinyl alcohol copolymer), offers additional promise in the endovascular treatment of spinal dural AVFs (Fig. 397-25). Onyx possesses some advantages over NBCA as an embolic agent: it can be delivered slowly in more controlled fashion over a period of several minutes, it has a lower risk for premature venous occlusion or occlusion of a large intradural portion of the draining vein, and it carries a low risk for a retained catheter. Large series of spinal dural AVFs do not exist. Although the experience with Onyx in treating intradural spinal vascular malformations128 and cranial dural AVFs129–131 suggests that Onyx may be effective for treatment of spinal dural AVFs, insufficient experience exists for conclusions to be reached on its utility.
Finally, hemorrhage132 and delayed paraplegia40,65 after embolization of spinal dural AVFs with NBCA have been reported. This suggests either propagation of venous thrombosis into the coronal venous plexus or passage of embolic material through the AVF and into the coronal venous plexus, thereby exacerbating the venous hypertension and leading to hemorrhage or venous infarction.
One clear indication for embolization of spinal dural AVFs is to treat patients with Foix-Alajouanine syndrome and incipient venous thrombosis and spinal cord infarction. A patient with an untreated dural AVF and rapid progression of symptoms has perilously high venous hypertension and is at risk for venous thrombosis and irreversible cord injury. Transarterial embolization of the fistula in these patients provides an immediate reduction in venous congestion until definitive surgical treatment can be performed.56
Intradural Vascular Malformations
Surgery
A number of general principles apply to the surgical management of all intradural AVMs.12,65,68,133–135 Surgery should be attempted only after careful consideration of the vascular anatomy of the lesion and the adjacent spinal cord as depicted by selective spinal arteriography. After acute subarachnoid or intraparenchymal hemorrhage, surgery is best delayed to permit lysis and absorption of the clot. Preoperative embolization with particulate or liquid embolic material or occlusion of giant feeders by balloon occlusion reduces the blood flow and tension in the AVM or AVF. Because it commonly leaves small particles in feeding vessels to a greater extent than in venous drainage vessels (E.H.O., personal observations), preoperative particulate embolization can also assist the surgeon in defining the feeding arteries during surgery. An operating microscope and appropriate microsurgical instrumentation are mandatory. Operative exposure must extend at least one level above and one level below the nidus of the AVM. The dura should be opened in the midline, and the arachnoid is preserved to avoid laceration of any delicate, distended, or adherent vessels underlying the dural opening. The use of small (2 to 3 mm × 10 to 15 mm) rectangular cotton pledgets or stay sutures secured to the pia-arachnoid facilitates the retraction and exposure provided by myelotomy for intramedullary vascular malformations. Painstaking hemostasis is crucial to maintain optimal visualization of the nidus of the AVM. The vascular anatomy defined on the preoperative angiogram must be carefully correlated to that seen intraoperatively to identify and confirm the major feeding and draining vessels and to plan the sequence of steps for excision.
Intraoperative ultrasonography with a directional flow probe or intraoperative arteriography136,137 may be helpful to localize the intramedullary nidus and to distinguish afferent from efferent vessels. Bipolar cautery, used judiciously, can reduce the size and turgor of the AVM. Continuous bipolar irrigation maintains optimal visualization and prevents adherence to friable vessels. Large feeding vessels may require ligatures or clips, but metallic clips are generally avoided so that they do not interfere with the results of postoperative imaging. Generally, one major draining vein is preserved until the last arterial feeders have been taken. Intraoperative arteriography can be used to detect any residual AVM that is still patent,136,137 but it may not detect small portions of thrombosed nidus that recanalize later. Hemostasis is reassessed completely before closure. The dura is closed in watertight fashion.
For certain ventral AVMs or AVFs, Martin and coworkers138 and Markert and colleagues139 described exposure of the ventrolateral quadrant of the spinal cord through an extended posterolateral approach that can be used in the cervical and thoracic regions. This technique includes a midline skin incision with a transverse extension at the level of the lesion, unilateral division and retraction of the paraspinous muscles, laminectomy and unilateral removal of the facets and pedicles, dural incision over the dorsal root entry zone, multilevel division of the ipsilateral dentate ligaments, and elevation and rotation of the spinal cord with dentate traction stitches. This technique provides exposure of the ventral root entry zone, the ipsilateral half of the ventral surface of the spinal cord, and the anterior spinal artery, although the surface of the spinal cord beyond the anterior spinal artery is not seen well. On rare occasion, anterior approaches (including cervical corpectomy and transthoracic variants) have been reported to successfully treat challenging intradural spinal vascular malformations, but closure and avoidance of cerebrospinal fluid leakage complications remain challenging. Additionally, these approaches often require fusion with its associated problems.140–142
Intramedullary Arteriovenous Malformations
Circumferential dissection is carried out along the gliotic plane between the intramedullary AVM and the adjacent neural tissue (Fig. 397-26). To facilitate dissection of deeper portions of the AVM, the dorsal portion of the spinal cord is gently retracted laterally with small cottonoids or fine pial sutures attached to the dura laterally. Although the outcome of operative treatment of spinal intramedullary AVMs is, in general, less satisfactory than the results of treatment of dural AVFs, there are several characteristics of glomus-type intramedullary AVMs that often render them technically less problematic than juvenile-type lesions. Unlike juvenile-type AVMs, glomus lesions are generally compact, lack intertwined neural elements, and usually have a single arterial feeding vessel.15 In addition, the cervical region has a more abundant collateral system than do the thoracolumbar cord segments, thus permitting total surgical excision of certain cervical intramedullary lesions.12,15,24,65,68,143 However, if a relatively large lesion is situated entirely intraparenchymally in the anterior half of the spinal cord, complete excision may yield unacceptable neurological deficits. Operative intervention may be inadvisable in this circumstance.
Because of their relative infrequency, no adequate long-term study comparing surgical and embolic treatment of intramedullary AVMs in the cervical region has been conducted. However, intramedullary AVMs in the thoracic and lumbar regions pose substantially greater operative risk because of their more tenuous collateral blood supply. Ventral intramedullary lesions in these segments are usually treated with repeated arteriographic embolization (see later).64
Because they are voluminous, high-flow lesions that usually contain neural tissue within the interstices of the component vessels of the AVM and derive their arterial supply from multiple medullary arteries that also supply the spinal cord, most juvenile-type intramedullary AVMs are considered inoperable. For patients with progressive myelopathy or hemorrhage, embolization is usually recommended (see later).64 An exception is a dorsally located lesion that is partly intramedullary and partly extramedullary.12,25,65,68,133,143 Presurgical or intraoperative embolization permits easier and safer excision and may improve the chance for total excision of these lesions.26–28143
Outcome
Furthermore, the natural history of intramedullary AVMs is variable (see earlier), and the arteriographic outcome of surgery is known for only 3 years after surgery (Table 397-7). Early postoperative arteriography revealed persistent AVMs in as many as 40% of patients treated surgically during the 1960s and 1970s.15 At 3 years after surgery, about 30% to 50% of patients are improved, 30% to 50% remain unchanged, and 10% to 20% are worse. However, there are limited data on the incidence of persistent AVMs, even as early as 3 years, and no long-term data exist on the incidence of clinical relapse or progression because of incomplete surgical excision. Moreover, there are no studies of delayed arteriographic follow-up of greater than 1 year after surgical treatment of intradural AVMs. The short-term clinical results (3-year follow-up) of surgery clearly indicate superior outcomes in patients who receive treatment before serious neurological deficits arise (Fig. 397-27). However, this must be balanced against the knowledge that some patients have an innocuous clinical course for many years without any treatment (see earlier and personal observations of E.H.O.).
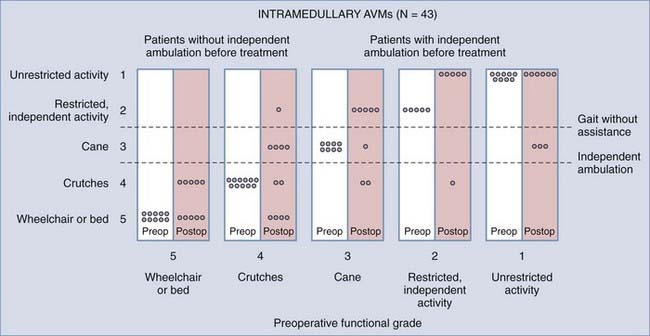
FIGURE 397-27 Relationship of preoperative functional state and postoperative outcome in patients with intramedullary arteriovenous malformations (AVMs). As in patients with dural arteriovenous fistulas (AVFs), the outcome after surgery is directly related to the patient’s neurological status preoperatively. Generally, patients who walked independently before treatment do so after treatment. Note, however, that many patients were not treated until they had already acquired severe functional disability. As in patients with dural AVFs (see Fig. 397-24), early diagnosis and therapy offer the best outcome for patients in both groups.
(From Muraszko KM, Oldfield EH. Vascular malformations of the spinal cord and dura. Neurosurg Clin N Am. 1990;1:631-652. Data from Rosenblum B, Oldfield EH, Doppman JL. Pathogenesis of spinal arteriovenous malformations. J Neurosurg. 1987;67:795-802.)
Embolization
Embolic occlusion of spinal AVMs developed as a natural extension of the introduction and refinement of selective spinal arteriography.10,27,144 With certain intradural AVMs, embolization is used alone. Because the incidence of recanalization after embolic occlusion is quite high,122,145 embolization may be used before surgery for lesions that can be excised with acceptable risk. The nidus of an intramedullary AVM, as occurs in hemangioblastomas,93 is often composed of several independent vascular compartments with varying degrees of overlapping common supply. Embolization, including the use of liquid embolizing materials such as the polymerizing acrylics, rarely permanently occludes the entire nidus of the AVM. For this reason, some clinicians who once advocated the routine use of glue for embolizing intradural spinal AVMs no longer do so because of the low incidence of lasting occlusion and the frequency of complications associated with it.63,64 However, more recent reports have created new enthusiasm for embolization of spinal AVMs with liquid embolic agents, including NBCA glue and Onyx.121,128,146,147
Although several particulate materials have been used to occlude spinal AVMs, including Gelfoam, PVA sponge microparticles, silk threads, and clot, the most commonly used particulate is PVA in the 150- to 250-µm-diameter range. Selection of the size of the particles is based on the desire to have them pass freely through the conducting vessels, the medullary arteries, the anterior or posterior spinal artery, and the enlarged sulcal vessels to the AVM without occluding the normal sulcal vessels. Because the diameter of the normal anterior spinal artery is 340 to 1100 µm and the diameter of the normal sulcal ateries is 60 to 72 µm, PVA particles 150 to 250 µm in diameter should pass through the anterior spinal artery, not enter the normal sulcal arteries, and pass into the enlarged sulcal arteries supplying the AVM.148,149 When the vessel providing the most direct route to the AVM is particularly difficult to engage with a catheter, such as feeding arteries from the cervical segment of the vertebral artery, the particles can be embolized in a flow-directed manner toward the nidus via temporary or permanent balloon occlusion of the artery just distal to the origin of the feeding vessel. With particulate embolization, progressive occlusion of the malformation is carefully monitored with repeated arteriographic examination, and the embolization is terminated when the lesion is almost completely occluded (in situ PVA continues to cause clot to form and propagate after completion of the procedure) or when there is retrograde reflux of contrast medium proximal to the catheter tip, a finding indicating that flow-directed targeting of the nidus will no longer occur. Liquid materials (e.g., NBCA, Onyx), which are less embolic, require placement of the catheter tip at the edge of the nidus of the AVM and injection of a small amount of the polymer. Once again, prolonged injections may be achieved with Onyx.
Even though embolization of spinal AVMs with PVA does not provide lasting elimination of flow through the AVM, it plays an important role in the current management of patients with intradural AVMs. Presurgical particulate embolization makes surgery easier, and when used as the only therapy, it reduces the risk for hemorrhage (which could cause quadriplegia or death), mollifies the severity of spasticity in some patients, and most important, delays neurological progression in most patients. The results obtained by Biondi and colleagues—who advocate routine yearly arteriography and embolization with PVA (Ivalon) particles, regardless of symptoms—suggest that serial endovascular embolization with PVA provides safe and successful management of most patients with AVMs in the thoracic region of the spinal cord.64 Their long-term follow-up (mean, 6 years) of 35 patients showed that although no patients had complete, permanent obliteration of the AVM (most patients had <50% reduction in AVM size), nearly two thirds were clinically improved at the end of the study. One of us (E.H.O.) has several patients with thoracic or lumbar ventral intramedullary AVMs that receive their blood supply from the anterior spinal artery whose neurological status has been stabilized for several years by repetitive particulate embolic occlusion when symptoms recur.
Experience with the use of Onyx for the embolization of spinal intramedullary AVMs has been reported recently.128 Seventeen patients with symptomatic spinal intramedullary AVMs were treated exclusively with Onyx. Thirteen patients underwent a single treatment session and 4 underwent two treatment sessions. The mean follow-up was 24 months. Total AVM obliteration was achieved in 6 patients (35%), subtotal obliteration was achieved in 5 patients (29%), and partial obliteration was achieved in 5 patients (29%). Improvement in neurological or functional status (or both) was noted in 14 patients (82%). Intraprocedural complications occurred in 2 patients without neurological sequelae. Although further follow-up is necessary to critically evaluate durability, the encouraging results of this study demonstrate the potential of Onyx for the treatment for spinal intramedullary AVMs.
As with surgery for intradural AVMs, the best results with embolization are achieved in patients without major neurological deficits before treatment.9,15,27,63,64,132,145,148,149 Selective catheterization and embolization are associated with risks of occlusion of the anterior spinal artery and paraplegia or quadriplegia, perforation of an intradural vessel with the catheter and subarachnoid or intramedullary hemorrhage,145 and passage of emboli through the AVM into the venous system and subsequent hemorrhage or venous infarction132 or into the distal vessels of the parent artery, such as the basilar artery when using the vertebral artery to reach the AVM.
Radiosurgery
Radiosurgery has been established as a potentially effective treatment option for select AVMs of the brain. However, a possible role for radiosurgery in the management of intramedullary spinal AVMs has not been extensively investigated. Sinclair and coauthors reported on the Stanford experience using multisession CyberKnife radiosurgery for intramedullary spinal AVMs.150 Their report included seven patients with a follow-up of greater than 3 years after treatment. Six of the seven patients had significant reductions in AVM volume on MRI. Five patients underwent follow-up arteriography, four of whom had residual AVMs (although reduced in size), and one patient with a conus AVM had obliteration of it. No evidence of further hemorrhage or neurological deterioration attributed to the radiosurgical treatment was noted. Taken together, this study demonstrates the feasibility of stereotactic radiosurgery for the treatment of intramedullary spinal AVMs. However, 3 years of follow-up is too early for radiation-induced myelopathy to appear, and thus its incidence, as well as the incidence of delayed recanalization, remains to be determined. This treatment needs further investigation before its role in the treatment of spinal AVMs can be assessed.
Summary of Treatment Selection for Intramedullary Malformations
As a result of rapidly and simultaneously evolving classification schemes, diagnostic techniques, surgical instrumentation, catheter sophistication, advances in embolic agents, and other factors, the results of contemporary surgery, alone or after embolization, for intradural AVMs are incompletely known. The information on surgery is limited to series that began 2 to 3 decades ago. The three surgical and single embolization series included in Table 397-7 are the only published series with more than 10 patients that permit tabulation of the data. Furthermore, for individual patients, there appear to be prominent selection biases for the use of surgery, embolization, or combined treatment in all series, surgical or embolization, large or small. For instance, although most intradural AVMs are located in the thoracic or thoracolumbar segments of the spinal cord, with few exceptions68 the published reports emphasizing surgical success focus on patients with cervical lesions,9,65,143 which arise in segments of the spinal cord with the greatest collateral blood supply. Moreover, there is a conspicuous bias for surgery on lesions in the dorsal half of the spinal cord, as exemplified by the tendency to provide surgical therapy for “long dorsal AVMs” before they were recognized to be the products of dural AVFs. Dorsal intramedullary AVMs are less likely to receive their blood supply from the anterior spinal artery and are associated with less surgical risk than ventral lesions are.133 The tendency to avoid subjecting patients in good condition to a therapeutic procedure that risks leaving them paraplegic results in a longer delay from diagnosis to surgical treatment with more difficult AVMs. In one series the average duration from the onset of symptoms to treatment was 2.7 years for dural AVFs versus 4.2 years for intradural AVMs, and patients with intradural AVMs were more likely to have worse functional grades at treatment than were patients with dural AVFs.15
Perimedullary Arteriovenous Fistulas
With intradural AVFs, the goal of treatment is precise interruption of the abnormal communication between the artery and vein. Elimination of the extramedullary fistulous connection of perimedullary AVFs may be achieved by surgery16,32,41,63,132 or embolization.14,41,146,147,151 For treatment purposes, these lesions have been divided by Merland and associates into three groups based on the caliber, length, and number of vessels supplying and draining the fistula (see Table 397-5): small fistulas in the conus or filum terminale supplied by a long, thin anterior spinal artery (type I) (see Fig. 397-14); larger fistulas in the pia posterolaterally or anterolaterally composed of several discrete shunts and fed by enlarged anterior or posterior spinal arteries (type II; see Fig. 397-12); and the most common type, single giant fistulas supplied by several enormously enlarged anterior and posterior spinal arteries (type III—Fig. 397-28; also see Fig. 397-15).41,63 In AVFs in which a long, small-caliber anterior spinal artery is the sole feeding vessel (type I perimedullary AVFs), embolization is not usually safe, and surgical intervention is generally safer and effective (see Fig. 397-14).16,32,41,104 With type II lesions, embolization may be useful to reduce blood flow through the AVF in preparation for surgery, but it is not generally curative when used alone, perhaps because of the multiplicity of the constituents of the AVF.30 Thus, surgical intervention is generally accepted as the treatment of choice for posterior or posterolateral perimedullary AVFs (see Figs. 397-12 and 397-14) or when embolization is inherently dangerous.30,33,41,69 Surgical interruption of AVFs supplied by the anterior spinal artery on the ventral surface of the cord in the midline has been performed successfully via a transvertebral approach32 and by posterolateral approaches with rotation of the spinal cord.16,138,139 Patients with the giant simple fistulas (type III AVF) can be treated with selective occlusion by detachable balloons (which permits a trial occlusion before disengagement), occlusion with coils, interruption with surgery (see Fig. 397-15), or a combination of embolic and surgical approaches (see Fig. 397-28).41,63 With perimedullary AVFs, as with the other forms of AVMs, better results are achieved in patients with early diagnosis and interruption before serious neurological deficits develop.15,30,33,41
Cavernous Angiomas
The usual considerations for surgery in treating intraparenchymal cord lesions apply in the management of cavernous angiomas. Lesions lying in the dorsal half of the cord, which are immediately accessible with a limited myelotomy over the most superficial aspect of the lesion (frequently indicated by bluish gray discoloration of the pia; see Figs. 397-6 and 397-16), require less manipulation of the spinal cord for exposure, are associated with less risk for additional neurological injury during surgery, and have a more favorable prognosis for improvement after removal than do ventral lesions. Techniques for removing cavernous angiomas (see Figs. 397-6 and 397-16) are similar to those for excising benign intramedullary spinal cord tumors, with the exception that at some sites the margin of the cavernoma may be more adherent to the contiguous spinal cord than is common in benign tumors. Because residual portions of cavernous angiomas, left in situ during surgery, tend to rehemorrhage and cause recurrent myelopathy,152,153 complete excision is mandatory and requires careful inspection of the bed of the angioma in the spinal cord before closure.44,89,153–155
With cavernous angiomas, as with dural and intradural spinal AVMs, the outcome after surgery depends greatly on the patient’s neurological function before surgery.44,89,90,152–154,156–160 Fifteen percent to 25% of patients with spinal cord cavernous angiomas have an increased deficit immediately after surgery. However, these deficits generally resolve with time, and most patients exhibit improvement or stabilization of their neurological deficits. Thus, the early and long-term outcome after surgical excision of cavernomas of the spinal cord is one of improvement or stabilization.152,153,157
Afshar JK, Doppman JL, Oldfield EH. Surgical interruption of intradural draining vein as curative treatment of spinal dural arteriovenous fistulas. J Neurosurg. 1995;82:196-200.
Aminoff M, Barnard R, Logue V. The pathophysiology of spinal vascular malformations. J Neurol Sci. 1974;23:255-263.
Aminoff M, Logue V. The prognosis of patients with spinal vascular malformations. Brain. 1974;97:211-218.
Aminoff M, Logue V. Clinical features of spinal vascular malformations. Brain. 1974;97:197-210.
Behrens S, Thron A. Long-term follow-up and outcome in patients treated for spinal dural arteriovenous fistula. J Neurol. 1999;246:181-185.
Biondi A, Merland JJ, Reizine D, et al. Embolization with particles in thoracic intramedullary arteriovenous malformations: long-term angiographic and clinical results. Radiology. 1990;177:651-658.
Cohen-Gadol AA, Jacob JT, Edwards DA, et al. Coexistence of intracranial and spinal cavernous malformations: a study of prevalence and natural history. J Neurosurg. 2006;104:376-381.
Corkill RA, Mitsos AP, Molyneux AJ. Embolization of spinal intramedullary arteriovenous malformations using the liquid embolic agent, Onyx: a single-center experience in a series of 17 patients. J Neurosurg Spine. 2007;7:478-485.
Hurth M, Houdart R, Djindjian R, et al. Arteriovenous malformations of the spinal cord: clinical, anatomical and therapeutic considerations—a series of 150 cases. Progr Neurol Surg. 1978;9:238-266.
Kendall B, Logue V. Spinal epidural angiomatous malformations draining into intrathecal veins. Neuroradiology. 1977;3:181-189.
Kharkar S, Shuck J, Conway J, et al. The natural history of conservatively managed symptomatic intramedullary spinal cord cavernomas. Neurosurgery. 2007;60:865-872.
Logue V. Angiomas of the spinal cord: review of the pathogenesis, clinical features, and results of surgery. J Neurol Neurosurg Psychiatry. 1979;42:1-11.
Narvid J, Hetts SW, Larsen D, et al. Spinal dural arteriovenous fistulae: clinical features and long-term results. Neurosurgery. 2008;62:159-166.
Niimi Y, Berenstein A, Setton A, et al. Embolization of spinal dural arteriovenous fistulae: results and follow- up. Neurosurgery. 1997;40:675-682.
Ogilvy C, Louis D, Ojemann R. Intramedullary cavernous angiomas of the spinal cord: clinical presentation, pathological features, and surgical management. Neurosurgery. 1992;31:219-230.
Oldfield EH, DiChiro G, Quindlen EA, et al. Successful treatment of a group of spinal cord arteriovenous malformations by interruption of dural fistula. J Neurosurg. 1983;59:1019-1030.
Rodesch G, Hurth M, Alvarez H, et al. Spinal cord intradural arteriovenous fistulae: anatomic, clinical, and therapeutic considerations in a series of 32 consecutive patients seen between 1981 and 2000 with emphasis on endovascular therapy. Neurosurgery. 2005;57:973-983.
Rodesch G, Hurth M, Alvarez H, et al. Embolization of spinal cord arteriovenous shunts: morphological and clinical follow-up and results—review of 69 consecutive cases. Neurosurgery. 2003;53:40-49.
Rosenblum B, Oldfield E, Doppman J, et al. Spinal arteriovenous malformations: a comparison of dural arteriovenous fistulas and intradural AVMs in 81 patients. J Neurosurg. 1987;67:795-802.
Sinclair J, Chang SD, Gibbs IC, et al. Multisession CyberKnife radiosurgery for intramedullary spinal cord arteriovenous malformations. Neurosurgery. 2006;58:1081-1089.
Symon L, Kuyama H, Kendall B. Dural arteriovenous malformations of the spine: clinical features and surgical results in 55 cases. J Neurosurg. 1984;60:238-247.
Veznedaroglu E, Nelson PK, Jabbour PM, et al. Endovascular treatment of spinal cord arteriovenous malformations. Neurosurgery. 2006;59(5 suppl 3):S202-S209.
Vishteh A, Sankhla S, Anson J, et al. Surgical resection of intramedullary spinal cord cavernous malformations: delayed complications, long-term outcomes, and associated cryptic venous malformations. Neurosurgery. 1997;41:1094-1101.
Wrobel CJ, Oldfield EH, DiChiro G, et al. Myelopathy due to intracranial dural arteriovenous fistulas draining intrathecally into spinal medullary veins. Report of three cases. J Neurosurg. 1988;69:934-939.
1 Elsberg C. Diagnosis and Treatment of Surgical Diseases of Spinal Cord and Its Membranes. Philadelphia: Saunders; 1916.
2 Oldfield EH, DiChiro G, Quindlen EA. Successful treatment of a group of spinal cord arteriovenous malformations by interruption of dural fistula. J Neurosurg. 1983;59:1019-1030.
3 Sargent P. Hemangioma of the pia mater causing compression paraplegia. Brain. 1925;48:259-267.
4 Wyburn-Mason R. The Vascular Abnormalities and Tumors of the Spinal Cord and Its Membranes. London: H. Klimpton; 1943.
5 Oldfield E, Doppman J. Spinal arteriovenous malformations. Clin Neurosurg. 1988;34:161-183.
6 Baker HL, Love JG, Layton DD. Angiographic and surgical aspects of spinal vascular anomalies. Radiology. 1967;88:1078-1085.
7 Di Chiro G, Doppman J, Ommaya A. Selective arteriography of arteriovenous aneurysms of spinal cord. Radiology. 1967;88:1067-1077.
8 Doppman J. The nidus concept of spinal cord arteriovenous fistulas. A surgical recommendation based upon angiographic observations. Br J Radiol. 1971;44:758-763.
9 Newton T, Adams J. Angiographic demonstration and nonsurgical embolization of spinal cord angioma. Radiology. 1968;91:873-876.
10 Doppman J, DiChiro G, Ommaya A. Selective Arteriography of the Spinal Cord. St. Louis: Warren H. Green; 1969.
11 Krayenbuhl H, Yasargil M, McClintock H. Treatment of spinal cord vascular malformations by surgical excision. J Neurosurg. 1969;30:427-435.
12 Malis L. Microsurgery for spinal cord arteriovenous malformations. Clin Neurosurg. 1979;26:543-555.
13 Kendall B, Logue V. Spinal epidural angiomatous malformations draining into intrathecal veins. Neuroradiology. 1977;3:181-189.
14 Djindjian M, Djindjian R, Rey A, et al. Intradural extramedullary spinal arteriovenous malformations fed by the anterior spinal artery. Surg Neurol. 1977;8:85-93.
15 Rosenblum B, Oldfield E, Doppman J, et al. Spinal arteriovenous malformations: a comparison of dural arteriovenous fistulas and intradural AVMs in 81 patients. J Neurosurg. 1987;67:795-802.
16 Aminoff M, Gutin P, Norman D. Unusual type of spinal arteriovenous malformation. Neurosurgery. 1988;22:589-591.
17 Foix C, Alajouanine T. La melite necrotique subaique. Rev Neurol. 1926;2:1-42.
18 Riche M, Modensesi-Freitas J, Djindjian M, et al. Arteriovenous malformations (AVM) of the spinal cord in children: a review of 38 cases. Neuroradiology. 1982;22:171-180.
19 Masaryk T, Ross J, Modic M, et al. Radiculomeningeal vascular malformations of the spine: MR imaging. Radiology. 1987;164:845-849.
20 Yamaguchi S, Eguchi K, Kiura Y, et al. Multi-detector-row CT angiography as a preoperative evaluation for spinal arteriovenous fistulae. Neurosurg Rev. 2007;30:321-326.
21 Thompson B, Oldfield E. Spinal vascular malformations. In: Carter L, Spetzler R, Hamilton M, editors. Neurovascular Surgery. New York: McGraw-Hill; 1994:1167-1195.
22 Woimant F, Merland J, Riche M, et al. Syndrome bulbo-medullaire en rapport avec une fistule arterio-venueuse meningee du sinus lateral a drainage veineux medullaire. Rev Neurol. 1982;138:559-566.
23 Larsen DW, Halbach VV, Teitelbaum GP, et al. Spinal dural arteriovenous fistulas supplied by branches of the internal iliac arteries. Surg Neurol. 1995;43:35-40.
24 Touho H, Karasawa J, Shishido H, et al. Successful excision of a juvenile-type spinal arteriovenous malformation following intraoperative embolization. Case report. J Neurosurg. 1991;75:647-651.
25 Houdart R, Djindjian R, Hurth M, et al. Treatment of angiomas of the spinal cord. Surg Neurol. 1974;2:186-194.
26 Ausman J, Gold L, Tadavarthy S, et al. Intraparenchymal embolization for obliteration of an intramedullary AVM of the spinal cord. J Neurosurg. 1977;47:119-125.
27 Doppman J, DiChiro G, Ommaya A. Percutaneous embolisation of spinal cord arteriovenous malformations. J Neurosurg. 1971;34:48-55.
28 Latchaw R, Harris R, Chou S, et al. Combined embolization and operation in the treatment of cervical arteriovenous malformations. Neurosurgery. 1980;6:131-137.
29 Tobin W, Layton D. The diagnosis and natural history of spinal cord arteriovenous malformations. Mayo Clin Proc. 1976;51:637-646.
30 Mourier KL, Gobin YP, George B, et al. Intradural perimedullary arteriovenous fistulae: results of surgical and endovascular treatment in a series of 35 cases. Neurosurgery. 1993;32:885-891.
31 Gillilan L. The arterial blood supply to the human spinal cord. J Comp Neurol. 1958;110:75-103.
32 Heros R, Debrun G, Ojemann R, et al. Direct spinal arteriovenous fistula: a new type of spinal AVM: case report. J Neurosurg. 1986;64:134-139.
33 Hida K, Iwasaki Y, Goto K, et al. Results of the surgical treatment of perimedullary arteriovenous fistulas with special reference to embolization. J Neurosurg. 1999;90:198-205.
34 Tender GC, Vortmeyer AO, Oldfield EH. Spinal intradural arteriovenous fistulas acquired in late adulthood: absent spinal venous drainage in pathogenesis and pathophysiology. Report of two cases. J Neurosurg Spine. 2005;3:488-494.
35 Suh T, Alexander L. Vascular system of the human spinal cord. Arch Neurol Psychiatry. 1939;41:659-677.
36 Turnbull I. Microvasculature of the human spinal cord. J Neurosurg. 1971;35:141-147.
37 Taveras J, Wood E. Diagnostic Neuroradiolgy, 2nd ed. Baltimore: Williams & Wilkins; 1976.
38 Gillilan L. Veins of the spinal cord. Anatomic details; suggested clinical applications. Neurology. 1970;20:860-868.
39 McCutcheon IE, Doppman JL, Oldfield EH. Microvascular anatomy of dural arteriovenous abnormalities of the spine: a microangiographic study. J Neurosurg. 1996;84:215-220.
40 Merland J, Riche M, Chiras J. Intraspinal extramedullary arteriovenous fistulae draining into the medullary veins. J Neuroradiol. 1980;7:271-320.
41 Gueguen B, Merland J, Riche M, et al. Vascular malformations of the spinal cord: intrathecal perimedullary arteriovenous fistulas fed by medullary arteries. Neurology. 1987;37:969-979.
42 Bicknell J, Carlow T, Kornfeld M. Familial cavernous angiomas. Arch Neurol. 1978;35:746-749.
43 Rigamonti D, Hadley M, Drayer B, et al. Cerebral cavernous malformations: incidence and familial occurrence. N Engl J Med. 1988;319:343-347.
44 Cosgrove G, Bertrand G, Fontaine S, et al. Cavernous angiomas of the spinal cord. J Neurosurg. 1988;68:31-34.
45 Craig HD, Gunel M, Cepeda O, et al. Multilocus linkage identifies two new loci for a mendelian form of stroke, cerebral cavernous malformation, at 7p15-13 and 3q25.2-27. Hum Mol Genet. 1998;7:1851-1858.
46 Dubovsky J, Zabramski JM, Kurth J, et al. A gene responsible for cavernous malformations of the brain maps to chromosome 7q. Hum Mol Genet. 1995;4:453-458.
47 Gunel M, Awad IA, Anson J, et al. Mapping a gene causing cerebral cavernous malformation to 7q11.2-q21. Proc Natl Acad Sci U S A. 1995;92:6620-6624.
48 Gunel M, Awad IA, Finberg K, et al. A founder mutation as a cause of cerebral cavernous malformation in Hispanic Americans. N Engl J Med. 1996;334:946-951.
49 Gunel M, Awad IA, Finberg K, et al. Genetic heterogeneity of inherited cerebral cavernous malformation. Neurosurgery. 1996;38:1265-1271.
50 Polymeropoulos MH, Hurko O, Hsu F, et al. Linkage of the locus for cerebral cavernous hemangiomas to human chromosome 7q in four families of Mexican-American descent. Neurology. 1997;48:752-757.
51 Brunereau L, Gobin YP, Meder JF, et al. Intracranial dural arteriovenous fistulas with spinal venous drainage: relation between clinical presentation and angiographic findings. AJNR Am J Neuroradiol. 1996;17:1549-1554.
52 Weingrad DN, Doppman JL, Chreitien PB, et al. Paraplegia due to postraumatic pelvic arteriovenous fistula treated by surgery and embolization. J Neurosurg. 1979;50:805-810.
53 Yoshino O, Matsui H, Hirano N, et al. Acquired dural arteriovenous malformation of the lumbar spine: case report. Neurosurgery. 1998;42:1387-1389.
54 Hassler W, Thron A, Grote E. Hemodynamics of spinal dural arteriovenous fistulas. An intraoperative study. J Neurosurg. 1989;70:360-370.
55 Symon L, Kuyama H, Kendall B. Dural arteriovenous malformations of the spine: clinical features and surgical results in 55 cases. J Neurosurg. 1984;60:238-247.
56 Criscuolo G, Oldfield E, Doppman J. Reversible acute and subacute myelopathy in patient with dural arteriovenous fistulas: Foix-Alajouanine syndrome reconsidered. J Neurosurg. 1989;70:354-359.
57 Oldfield E. Spinal vascular malformations. In: Swash M, editor. Outcomes in Neurological and Neurosurgical Disorders. Cambridge, UK: Cambridge University Press, 1998.
58 Aminoff M, Logue V. Clinical features of spinal vascular malformations. Brain. 1974;97:197-210.
59 Aminoff M, Logue V. The prognosis of patients with spinal vascular malformations. Brain. 1974;97:211-218.
60 Hurth M, Houdart R, Djindjian R, et al. Arteriovenous malformations of the spinal cord: clinical, anatomical and therapeutic considerations—a series of 150 cases. Prog Neurol Surg. 1978;9:238-266.
61 Aminoff M, Barnard R, Logue V. The pathophysiology of spinal vascular malformations. J Neurol Sci. 1974;23:255-263.
62 Logue V. Angiomas of the spinal cord: review of the pathogenesis, clinical features, and results of surgery. J Neurol Neurosurg Psychiatry. 1979;42:1-11.
63 Riche M, Melki J, Merland J. Embolization of spinal cord vascular malformations via the anterior spinal artery. AJNR Am J Neuroradiol. 1983;4:378-381.
64 Biondi A, Merland JJ, Reizine D, et al. Embolization with particles in thoracic intramedullary arteriovenous malformations: long-term angiographic and clinical results. Radiology. 1990;177:651-658.
65 Yasargil M, Symon L, Teddy P. Arteriovenous malformations of the spinal cord. In: Symon L, editor. Advances and Technical Standards in Neurosurgery. Wien: Springer-Verlag; 1984:61-102.
66 Bandyopadhyay S, Sheth RD. Acute spinal cord infarction: vascular steal in arteriovenous malformation. J Child Neurol. 1999;14:685-687.
67 Djindjian M, Djindjian R, Hurth M, et al. Steal phenomenon in spinal arteriovenous malformations. J Neuroradiol. 1978;5:187.
68 Cogen P, Stein B. Spinal cord arteriovenous malformations with significant intramedullary components. J Neurosurg. 1983;59:471-478.
69 Barrow DL, Colohan AR, Dawson R. Intradural perimedullary arteriovenous fistulas (type IV spinal cord arteriovenous malformations). J Neurosurg. 1994;81:221-229.
70 Sure U, Wakat J, Gatscher S, et al. Spinal type IV arteriovenous malformations (perimedullary fistulas) in children. Childs Nerv Syst. 2000;16:508-515.
71 Simard J, Garcia-Bengochea F, Ballinger W, et al. Cavernous angioma: a review of 126 collected and 12 new clinical cases. Neurosurgery. 1986;18:162-172.
72 Voigt K, Yasargil M. Cerebral cavernous hemangiomas or cavernomas: incidence, pathology, localization, diagnosis, clinical features and treatment. Review of the literature and report of an unusual case. Neurochir (Stuttg). 1976;19:59-68.
73 Brunereau L, Labauge P, Tournier-Lasserve E, et al. Familial form of intracranial cavernous angioma: MR imaging findings in 51 families. French Society of Neurosurgery. Radiology. 2000;214:209-216.
74 Hayman LA, Evans RA, Ferrell RE, et al. Familial cavernous angiomas: natural history and genetic study over a 5- year period. Am J Med Genet. 1982;11:147-160.
75 Labauge P, Laberge S, Brunereau L, et al. Hereditary cerebral cavernous angiomas: clinical and genetic features in 57 French families. Societe Francaise de Neurochirurgie. Lancet. 1998;352:1892-1897.
76 Passarin MG, Salviati A, Gambina G, et al. Familial cavernous hemangioma with atypical neuroimaging. Ital J Neurol Sci. 1996;17:295-300.
77 Siegel AM. Familial cavernous angioma: an unknown, known disease [editorial; comment]. Acta Neurol Scand. 1998;98:369-371.
78 Siegel AM, Andermann E, Badhwar A, et al. Anticipation in familial cavernous angioma: a study of 52 families from International Familial Cavernous Angioma Study. IFCAS Group [letter]. Lancet. 1998;352:1676-1677.
79 Gil-Nagel A, Dubovsky J, Wilcox KJ, et al. Familial cerebral cavernous angioma: a gene localized to a 15-cM interval on chromosome 7q [published erratum appears in Ann Neurol 1996;40:480]. Ann Neurol. 1996;39:807-810.
80 Johnson EW, Iyer LM, Rich SS, et al. Refined localization of the cerebral cavernous malformation gene (CCM1) to a 4-cM interval of chromosome 7q contained in a well-defined YAC contig. Genome Res. 1995;5:368-380.
81 Laberge S, Labauge P, Marechal E, et al. Genetic heterogeneity and absence of founder effect in a series of 36 French cerebral cavernous angiomas families. Eur J Hum Genet. 1999;7:499-504.
82 Notelet L, Chapon F, Khoury S, et al. Familial cavernous malformations in a large French kindred: mapping of the gene to the CCM1 locus on chromosome 7q. J Neurol Neurosurg Psychiatry. 1997;63:40-45.
83 Siegel AM, Andermann F, Badhwar A, et al. Anticipation in familial cavernous angioma: ascertainment bias or genetic cause. Acta Neurol Scand. 1998;98:372-376.
84 Zhang J, Clatterbuck RE, Rigamonti D, et al. Mutations in KRIT1 in familial cerebral cavernous malformations. Neurosurgery. 2000;46:1272-1277.
85 Larson H, Ball W, Bove K, et al. Formation of intracerebral cavernous malformations after radiation treatment for central nervous system neoplasia in children. J Neurosurg. 1998;88:51-56.
86 Maraire JN, Abdulrauf SI, Berger S, et al. De novo development of a cavernous malformation of the spinal cord following spinal axis radiation. Case report. J Neurosurg. 1999;90:234-238.
87 Massa-Micon B, Luparello V, Bergui M, et al. De novo cavernoma case report and review of literature. Surg Neurol. 2000;53:484-487.
88 Novelli P, Reigel D, Gleatson P, et al. Multiple cavernous angiomas after high-dose whole-brain radiation therapy. Pediatr Neurosurg. 1997;26:322-325.
89 Ogilvy C, Louis D, Ojemann R. Intramedullary cavernous angiomas of the spinal cord: clinical presentation, pathological features, and surgical management. Neurosurgery. 1992;31:219-230.
90 Labauge P, Bouly S, Parker F, et al. Outcome in 53 patients with spinal cord cavernomas. Surg Neurol. 2008;70:176-181.
91 Cohen-Gadol AA, Jacob JT, Edwards DA, et al. Coexistence of intracranial and spinal cavernous malformations: a study of prevalence and natural history. J Neurosurg. 2006;104:376-381.
92 Kharkar S, Shuck J, Conway J, et al. The natural history of conservatively managed symptomatic intramedullary spinal cord cavernomas. Neurosurgery. 2007;60:865-872.
93 Di Chiro G, Doppman J, Dwyer A, et al. Tumors and arteriovenous malformations of the spinal cord: assessment using MR. Radiology. 1985;156:689-697.
94 Doppman J, DiChiro G, Dwyer A, et al. Magnetic resonance imaging of spinal cord arteriovenous malformations. J Neurosurg. 1987;66:830-834.
95 Dormont D, Gelbert F, Assouline E, et al. MR Imaging of spinal arteriovenous malformations at 0.5 T: study of 34 cases. AJNR Am J Neuroradiol. 1988;9:833-838.
96 Minami S, Sagoh T, Nishimura K, et al. Spinal arteriovenous malformations: MR imaging. Radiology. 1988;169:109-115.
97 Terwey B, Becker H, Thron A, et al. Gadolinium-DTPA enhanced MR imaging of spinal dural arteriovenous fistulas. J Comput Assist Tomogr. 1989;13:30-37.
98 Ali S, Cashen TA, Carroll TJ, et al. Time-resolved spinal MR angiography: initial clinical experience in the evaluation of spinal arteriovenous shunts. AJNR Am J Neuroradiol. 2007;28:1806-1810.
99 Mull M, Nijenhuis RJ, Backes WH, et al. Value and limitations of contrast-enhanced MR angiography in spinal arteriovenous malformations and dural arteriovenous fistulas. AJNR Am J Neuroradiol. 2007;28:1249-1258.
100 Gilbertson JR, Miller GM, Goldman MS, et al. Spinal dural arteriovenous fistulas: MR and myelographic findings. AJNR Am J Neuroradiol. 1995;16:2049-2057.
101 Hurst RW, Grossman RI. Peripheral spinal cord hypointensity on T2-weighted MR images: a reliable imaging sign of venous hypertensive myelopathy. AJNR Am J Neuroradiol. 2000;21:781-786.
102 Horikoshi T, Hida K, Iwasaki Y, et al. Chronological changes in MRI findings of spinal dural arteriovenous fistula. Surg Neurol. 2000;53:243-249.
103 Willinsky RA, terBrugge K, Montanera W, et al. Posttreatment MR findings in spinal dural arteriovenous malformations. AJNR Am J Neuroradiol. 1995;16:2063-2071.
104 Mourier KL, Gelbert F, Rey A, et al. Spinal dural arteriovenous malformations with perimedullary drainage. Indications and results of surgery in 30 cases. Acta Neurochir (Wien). 1989;100:136-141.
105 Si-jia G, Meng-wei Z, Xi-ping L, et al. The clinical application studies of CT spinal angiography with 64-detector row spiral CT in diagnosing spinal vascular malformations. Eur J Radiol. 2009;71:22-28.
106 Wrobel CJ, Oldfield EH, DiChiro G, et al. Myelopathy due to intracranial dural arteriovenous fistulas draining intrathecally into spinal medullary veins. Report of three cases. J Neurosurg. 1988;69:934-939.
107 Fassett DR, Rammos SK, Patel P, et al. Intracranial subarachnoid hemorrhage resulting from cervical spine dural arteriovenous fistulas: literature review and case presentation. Neurosurg Focus. 2009;26(1):E4.
108 Stein SC, Ommaya A, Doppman JL, et al. Arteriovenous malformation of the cauda equina with arterial supply from branches of the internal iliac arteries. J Neurosurg. 1972;36:649-651.
109 Oldfield EH, Bennett A, Chen MY, et al. Successful management of patients with spinal dural arteriovenous fistulas and negative arteriography. J Neurosurg. 2002;96(2 suppl):220-229.
110 Luessenhop A, De La Cruz T. The surgical excision of spinal intradural vascular malformations. J Neurosurg. 1969;330:552-559.
111 Morgan MK, Marsh WR. Management of spinal dural arteriovenous malformations. J Neurosurg. 1989;70:832-836.
112 Afshar JK, Doppman JL, Oldfield EH. Surgical interruption of intradural draining vein as curative treatment of spinal dural arteriovenous fistulas. J Neurosurg. 1995;82:196-200.
113 Behrens S, Thron A. Long-term follow-up and outcome in patients treated for spinal dural arteriovenous fistula. J Neurol. 1999;246:181-185.
114 Westphal M, Koch C. Management of spinal dural arteriovenous fistulae using an interdisciplinary neuroradiological/neurosurgical approach: experience with 47 cases. Neurosurgery. 1999;45:451-457.
115 Doppman J, DiChiro G, Oldfield E. Origin of spinal arteriovenous malformation and normal cord vasculature from a common segmental artery: angiographic and therapeutic considerations. Radiology. 1985;154:687-689.
116 Cecchi PC, Musumeci A, Faccioli F, et al. Surgical treatment of spinal dural arterio-venous fistulae: long-term results and analysis of prognostic factors. Acta Neurochir (Wien). 2008;150:563-570.
117 Narvid J, Hetts SW, Larsen D, et al. Spinal dural arteriovenous fistulae: clinical features and long-term results. Neurosurgery. 2008;62:159-166.
118 Tacconi L, Lopez Izquierdo BC, Symon L. Outcome and prognostic factors in the surgical treatment of spinal dural arteriovenous fistulas. A long-term study. Br J Neurosurg. 1997;11:298-305.
119 Mullan S. Reflections upon the nature and management of intracranial and intraspinal vascular malformations and fistulae. J Neurosurg. 1994;80:606-616.
120 Thompson BG, Doppman JL, Oldfield EH. Treatment of cranial dural arteriovenous fistulae by interruption of leptomeningeal venous drainage. J Neurosurg. 1994;80:617-623.
121 Veznedaroglu E, Nelson PK, Jabbour PM, et al. Endovascular treatment of spinal cord arteriovenous malformations. Neurosurgery. 2006;59(5 suppl 3):S202-S209.
122 Hall W, Oldfield E, Doppman J. Recanalization of spinal cord arteriovenous malformations following embolization. J Neurosurg. 1989;70:714-720.
123 Gruber A, Mazal P, Bavinski G, et al. Repermeation of partially embolized cerebral arteriovenous malformations: a clinical, radiologic, and histologic study. AJNR Am J Neuroradiol. 1996;17:1323-1331.
124 Birchall D, Hughes DG, West CG. Recanalisation of spinal dural arteriovenous fistula after successful embolisation. J Neurol Neurosurg Psychiatry. 2000;68:792-793.
125 Merland J, Assouline E, Rufenacht D, et al. Dural spinal arteriovenous fistulae draining into medullary veins. Clinical and radiological results of treatment (embolization and surgery) in 56 cases. In: XIIIth Congress of the European Society of Neuroradiology. Amsterdam: Elsevier; 1985.
126 Niimi Y, Berenstein A, Setton A, et al. Embolization of spinal dural arteriovenous fistulae: results and follow-up. Neurosurgery. 1997;40:675-682.
127 Guillevin R, Vallee JN, Cormier E, et al. N-butyl 2-cyanoacrylate embolization of spinal dural arteriovenous fistulae: CT evaluation, technical features, and outcome prognosis in 26 cases. AJNR Am J Neuroradiol. 2005;26:929-935.
128 Corkill RA, Mitsos AP, Molyneux AJ. Embolization of spinal intramedullary arteriovenous malformations using the liquid embolic agent, Onyx: a single-center experience in a series of 17 patients. J Neurosurg Spine. 2007;7:478-485.
129 Carlson AP, Taylor CL, Yonas H. Treatment of dural arteriovenous fistula using ethylene vinyl alcohol (Onyx) arterial embolization as the primary modality: short-term results. J Neurosurg. 2007;107:1120-1125.
130 Cognard C, Januel AC, Silva NAJr, et al. Endovascular treatment of intracranial dural arteriovenous fistulas with cortical venous drainage: new management using Onyx. AJNR Am J Neuroradiol. 2008;29:235-241.
131 Nogueira RG, Dabus G, Rabinov JD, et al. Preliminary experience with Onyx embolization for the treatment of intracranial dural arteriovenous fistulas. AJNR Am J Neuroradiol. 2008;29:91-97.
132 Djindjian R. Embolization of angiomas of the spinal cord. Surg Neurol. 1975;4:411-420.
133 Connolly ESJr, Zubay GP, McCormick PC, et al. The posterior approach to a series of glomus (type II) intramedullary spinal cord arteriovenous malformations. Neurosurgery. 1998;42:774-785.
134 Yasargil M, DeLong W, Guarnaschelli J. Complete microsurgical excision of cervical extramedullary and intramedullary vascular malformations. Surg Neurol. 1975;4:211-224.
135 Oldfield E. Spinal vascular malformations. In: Macdonald R, editor. Neurosurgical Operative Atlas: Vascular Neurosurgery. New York: Thieme; 2008:190-199.
136 Barrow DL, Boyer KL, Joseph GJ. Intraoperative angiography in the management of neurovascular disorders. Neurosurgery. 1992;30:153-159.
137 Schievink WI, Vishteh AG, McDougall CG, et al. Intraoperative spinal angiography. J Neurosurg. 1999;90:48-51.
138 Martin NA, Khanna RK, Batzdorf U. Posterolateral cervical or thoracic approach with spinal cord rotation for vascular malformations or tumors of the ventrolateral spinal cord. J Neurosurg. 1995;83:254-261.
139 Markert JM, Chandler WF, Deveikis JP, et al. Use of the extreme lateral approach in the surgical treatment of an intradural ventral cervical spinal cord vascular malformation: technical case report. Neurosurgery. 1996;38:412-415.
140 Hida K, Iwasaki Y, Ushikoshi S, et al. Corpectomy: a direct approach to perimedullary arteriovenous fistulas of the anterior cervical spinal cord. J Neurosurg. 2002;96:157-161.
141 MacFarlane MR, Burn PJ, Evison J. Excision of high and mid cervical spinal cord arteriovenous malformations by anterior operation. J Clin Neurosci. 2005;12:71-79.
142 Williams FC, Zabramski JM, Spetzler RF, et al. Anterolateral transthoracic transvertebral resection of an intramedullary spinal arteriovenous malformation. Case report. J Neurosurg. 1991;74:1004-1008.
143 Spetzler RF, Zabramski JM, Flom RA. Management of juvenile spinal AVM’s by embolization and operative excision. Case report. J Neurosurg. 1989;70:628-632.
144 Doppman J, DiChiro G, Ommaya A. Obliteration of spinal-cord arteriovenous malformations by percutaneous embolisation. Lancet. 1968;1:477.
145 Touho H, Karasawa J, Ohnishi H, et al. Superselective embolization of spinal arteriovenous malformations using the Tracker catheter. Surg Neurol. 1992;38:85-94.
146 Rodesch G, Hurth M, Alvarez H, et al. Embolization of spinal cord arteriovenous shunts: morphological and clinical follow-up and results—review of 69 consecutive cases. Neurosurgery. 2003;53:40-49.
147 Rodesch G, Hurth M, Alvarez H, et al. Spinal cord intradural arteriovenous fistulae: anatomic, clinical, and therapeutic considerations in a series of 32 consecutive patients seen between 1981 and 2000 with emphasis on endovascular therapy. Neurosurgery. 2005;57:973-983.
148 Horton J, Latchaw R, Gold L, et al. Embolisation of intramedullary arteriovenous malformations of the spinal cord. AJNR Am J Neuroradiol. 1986;7:113-118.
149 Theron J, Cosgrove R, Melanson D, et al. Spinal arteriovenous malformationss: advances in therapeutic embolization. Radiology. 1986;158:163-169.
150 Sinclair J, Chang SD, Gibbs IC, et al. Multisession CyberKnife radiosurgery for intramedullary spinal cord arteriovenous malformations. Neurosurgery. 2006;58:1081-1089.
151 Riche M, Scialfa G, Gueguen B, et al. Giant extramedullary arteriovenous fistulas supplied by the anterior spinal artery: treatment by detachable balloons. AJNR Am J Neuroradiol. 1983;4:391-394.
152 Amin-Hanjani S, Ogilvy CS, Ojemann R, et al. Risks of surgical management for cavernous malformations of the nervous system. Neurosurgery. 1998;42:1220-1228.
153 Vishteh A, Sankhla S, Anson J, et al. Surgical resection of intramedullary spinal cord cavernous malformations: delayed complications, long-term outcomes, and associated cryptic venous malformations. Neurosurgery. 1997;41:1094-1101.
154 Anson JA, Spetzler RF. Surgical resection of intramedullary spinal cord cavernous malformations. J Neurosurg. 1993;78:446-451.
155 Tyndel F, Bilboa J, Hudson A, et al. Hemangioma calcificans of the spinal cord. Can J Neurol Sci. 1985;12:321-322.
156 Cantore G, Delfini R, Cervoni L, et al. Intramedullary cavernous angiomas of the spinal cord: report of six cases. Surg Neurol. 1995;43:448-452.
157 Cristante L, Herrmann H. Radical excision of intramedullary cavernous angiomas. Neurosurgery. 1998;43:424-431.
158 Harrison MJ, Eisenberg MB, Ullman JS, et al. Symptomatic cavernous malformations affecting the spine and spinal cord. Neurosurgery. 1995;37:195-204.
159 McCormick P, Michelsen W, Post K, et al. Cavernous malformations of the spinal cord. Neurosurgery. 1988;23:459-463.
160 Padovani R, Acciarri N, Giulioni M, et al. Cavernous angiomas of the spinal district: surgical treatment of 11 patients. Eur Spine J. 1997;6:298-303.