CHAPTER 79 Pallidal Interventions for Parkinson’s Disease
In the context of movement disorders such as Parkinson’s disease (PD), pallidal surgery refers to lesioning or chronic electrical stimulation (deep brain stimulation [DBS]) of the posterior GPi. Lesioning of the GPi, or pallidotomy, was first performed in patients with PD in the 1940s and 1950s, before any theoretical understanding of its function. The first modern study of pallidotomy for PD (performed in the era of computed tomography and magnetic resonance imaging) was that of Laitinen and colleagues1 in 1992. In the 1990s, chronic DBS emerged as a reversible, adjustable alternative to stereotactic lesioning and was used at all targets where lesioning was effective. GPi-DBS was first reported by Siegfried and Lippitz2 in 1994. Several controlled studies have shown that either GPi-DBS or STN-DBS can modify all cardinal motor signs of PD.3 DBS is safer than pallidotomy for bilateral intervention. Currently, for patients undergoing DBS for PD, the STN is the more commonly selected target, based on evidence that STN-DBS allows a greater reduction in antiparkinsonian medications and a lower stimulation voltage than GPi-DBS. However, randomized studies comparing GPi and STN are showing comparable efficacy,4 and there may be subgroups of PD patients for whom GPi-DBS is preferred. At this time, GPi-DBS for PD is an “on-label” indication for the Medtronic Activa DBS system (Minneapolis, MN).
Mechanism of Action
In the parkinsonian state, neuronal discharge in the GPi and STN is abnormal in several ways: spontaneous firing rates are elevated, neurons that normally discharge independently develop abnormal synchrony, bursting discharge is increased, neurons show oscillations in both the theta to alpha (3 to 8 Hz) and low beta (8 to 20 Hz) ranges, and cells that normally respond to movement of a single joint show responses to multiple joints (loss of joint selectivity).5 Pallidotomy, when it covers the majority of the motor territory of the GPi, eliminates these abnormalities. Although it clearly does not restore normal function (presumably, it reduces GPi output to zero), it partially corrects the cortical metabolic abnormalities found in PD.6 Apparently, motor-controlling cortical areas in adults can function more normally without basal ganglia output than under the influence of the highly abnormal basal ganglia output characteristic of PD.
With regard to GPi-DBS, the mechanism of action is less clear. Originally conceived of as a “reversible lesion,” it now appears that DBS at the frequencies used in PD surgery (100 to 200 Hz) drives the efferent axons of the stimulated structure. The effect on neuronal cell bodies is not certain. GPi-DBS decreases the firing rates in one of its major efferent targets, the ventrolateral thalamus, consistent with activation of the GABAergic pallidothalamic projection.7 Thus, unlike pallidotomy, GPi-DBS does not suppress the abnormally increased GPi firing rates, although it probably does reduce bursting and oscillatory activity in the most detrimental (alpha to beta) frequency ranges.
Indications
Indications for pallidal surgery in PD are as follows:
Contraindications to pallidal surgery in PD are as follows:
In addition to the indications listed, good candidates for pallidal lesioning include patients with asymmetric symptoms (because pallidotomy can be safely performed on only one side) and patients who will have difficulty complying with the follow-up required for the management of deep brain stimulators. For patients requiring bilateral surgery and who can comply with follow-up appointments, bilateral DBS is generally preferred. The exact indications for GPi-DBS versus STN-DBS have not been well defined.4,8 There is no evidence that either pallidotomy or GPi-DBS is neuroprotective against ongoing brain degeneration in PD; thus, they should not be performed early in the disease when symptoms are well managed medically.
Results
Outcomes for surgical therapy in PD are described in terms of standard rating scales of parkinsonian motor signs and symptoms, the most prevalent of which is the Unified Parkinson’s Disease Rating Scale (UPDRS). In a randomized trial of pallidotomy with a medical control arm and blinded evaluations, unilateral pallidotomy produced a 32% decrease (improvement) in the total UPDRS score at 1 year, which was significant compared with a 5% increase in the control group.9 A European study produced similar results at 6-month follow-up.10
Unilateral pallidal stimulation produced similar results to unilateral pallidotomy in a randomized study.11 Unilateral GPi-DBS has also been compared with unilateral STN-DBS, and both procedures produced similar improvements in contralateral bradykinesia12 and manual dexterity.13 The only published randomized comparison of bilateral GPi-DBS and bilateral STN-DBS showed a 38% improvement in motor UPDRS in the GPi group not taking medication, compared with 48% in the STN group. However, cognitive and behavioral complications were observed only in the STN group.4 A large study (300 patients) is in progress comparing bilateral pallidal DBS (and bilateral STN-DBS) with a medical control arm.
Complications
The most serious intraoperative or early postoperative complication of DBS or pallidotomy is intracerebral hemorrhage. In the series reported by Binder and colleagues,14 the risk of symptomatic hemorrhage from DBS surgery on a per patient basis was 2.1%, which is consistent with other large series. Early postoperative complications include mental status changes and hardware infection. In the report by Sillay and coworkers,15 the incidence of perioperative (within 6 months) device infection requiring a return to the operating room for partial or complete hardware removal was 4.5% per patient. Long-term hardware-related complications include skin erosion, lead fracture, pulse generator migration, and lead twisting. The incidence of lead fracture and other hardware complications increases with longer postoperative follow-up time and may be as high as 8.4% per electrode-year.16
The risk of hemorrhagic stroke is probably similar for pallidotomy and pallidal DBS. Lesioning surgery does not entail any hardware-related complications and has a much lower rate of infection owing to the absence of an indwelling device. Lesioning, however, carries certain risks that are not present with DBS surgery. It is possible to create inadvertent thermal lesions in critical structures surrounding the intended target, such as the corticobulbar tract (CBT), cortisospinal tract (CST), or optic tract (OT). Bilateral lesioning of the GPi carries a high risk of permanent speech or cognitive dysfunction, even when lesions are correctly placed.17,18 Delayed ischemic subcortical infarction appears to be more common following pallidotomy than after GPi-DBS.19,20 This may relate to thermal damage to neighboring perforating vessels at the time of radiofrequency lesioning.
Surgical Technique
The optimal methods for movement disorder surgery are far from standardized and continue to evolve.
Stereotactic Localization
Figure 79-1 shows the intended active contact location for GPi-DBS within the posterior motor territory of the nucleus. Leads are placed so that the active contact is 3 to 4 mm from the border between the GPi and the internal capsule; this allows the stimulation-induced electrical effect to spread throughout most of the motor territory of the GPi without spreading to the internal capsule. For pallidotomy, the lesion includes the point shown but should extend to the border of the internal capsule posteromedially, the border of the GPe dorsolaterally, and the base of the pallidum inferiorly. Including the GPe in the lesion reduces the efficacy of pallidotomy.21
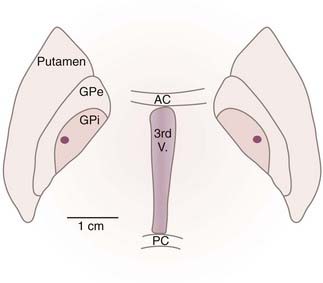
FIGURE 79-1 Typical active contact location for deep brain stimulation of the globus pallidus interna (GPi; dot) with respect to axial plane anatomy drawn from the Schaltenbrand and Wahren26 atlas of the human brain. The axial plane selected passes through the intercommissural line. AC, anterior commissure; GPe, globus pallidus externa; PC, posterior commissure; 3rd V, third ventricle.
(Modified from Starr PA. Placement of deep brain stimulators into the subthalamic nucleus or globus pallidus internus: technical approach. Stereotact Funct Neurosurg. 2002;79:118-145.)