14 Spinal Metastases
Introduction
Spinal metastases may affect different components of the spine, and the approach to treatment depends on correct localization of the affected compartment. Metastases to the spine most frequently involve the vertebral elements and the epidural space.1–4 In a small proportion of patients, symptoms and signs may arise from metastases that spread to the intradural spinal compartment, either extramedullary or intramedullary (Table 14-1).5–7 The approach to treatment varies according to the affected site, and ideally requires the shared decision of a multidisciplinary team. The specific treatment may include various combinations of steroids, irradiation, surgery, chemotherapy, hormonotherapy, and bisphosphonates, as appropriate for the underlying neoplasm.
Vertebral metastases
Vertebral metastases with epidural extension Epidural metastases without vertebral lesion Intradural metastases |
Vertebral Metastases
Metastases most commonly affect the lungs, liver, and skeletal system. Bone metastases are a significant cause of morbidity, due to pain, pathologic fractures, hypercalcemia, and spinal cord compression.3,4,8,9 The vertebral column is the most common site for skeletal metastases,10 and in autopsy series, 60% of patients dying of cancer were found to have spinal and epidural metastases.11 Destructive vertebral lesions are a common source of morbidity, with pain the presenting complaint in the majority of cases.
All patients with vertebral metastases are at potential risk of developing spinal instability and neurological impairment. Therefore, it is useful to classify vertebral metastases according to biomechanical stability and the presence or absence of dural sac displacement or neural element compression (Table 14-1). This classification allows for early recognition of potential complications. Treatment can be adjusted to meet the potential risks and preserve or restore normal function.
Assessment of the extent of vertebral metastases is based on imaging findings that range from spine radiography and bone scintigraphy to positron emission tomography (PET) using F-18 2-fluoro-2-deoxy-D-glucose (FDG),12 computerized tomography (CT), and magnetic resonance imaging (MRI). MRI is sensitive to both focal vertebral lesions and bone marrow involvement, in both hematologic malignancies and in solid tumors.13
CLINICAL AND IMAGING CRITERIA FOR SPINAL STABILITY IN NEOPLASTIC DISEASE
Determining spinal stability is of paramount importance in choosing the appropriate form of management for patients with spinal metastases, since a major goal of treatment is restoration or maintenance of spinal stability. In trauma, the accepted biomechanical model for thoracolumbar stability after fractures is the three-column concept of the spine (Figure 14-1).14
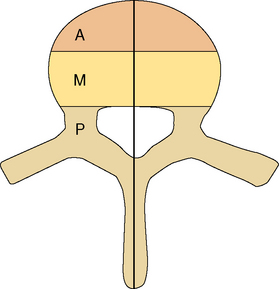
Figure 14-1 Classification system for the evaluation of spinal stability. The three-column system of Denis14 was devised for assessment of spinal column stability in trauma. The system divides the spine into the anterior (A), middle (M), and posterior (P) columns. The spine is considered unstable if two of the three columns are disrupted. The six-column system of Kostuik and Errico15 was devised for evaluating stability in spine tumors. Here the three columns, as defined by Denis, are subdivided into left (L) and right (R) halves. The spine is unstable if three to four of the columns are destroyed.
In neoplastic destruction of the spine these concepts may not always be applicable, because trauma and tumors are quite different conditions in terms of the disruption of bone, disc, and ligament; the quality of surrounding bone stock; and the ability of the spine to heal. A set of criteria, which requires validation, has been developed for spinal tumors.15 The spine is divided into six columns: the three columns defined above14 subdivided into left and right halves (Figure 14-1). The spine is considered to be unstable if three to four of the columns are destroyed, and markedly unstable if five to six of the columns are involved. Angulation of 20 degrees or more adds to the consideration of instability.
Instability does not usually develop when involvement is limited to the vertebral spongy bone core or to the anterior column. When the posterior half (middle column) of the vertebral body is also involved (cortical bone included), pathologic compression fracture can occur, producing kyphosis and extrusion of bone, tumor, or disc into the spinal canal, with resulting neural compromise. Tumor involvement of the middle and posterior column may produce forward shearing deformity. In addition, segmental instability is probably present when the clinical syndrome is characterized by pain that is aggravated by movement (in the absence of significant neural encroachment), and associated with progressive collapse of vertebral bodies or localized kyphosis on imaging studies. The MRI appearance of acute vertebral fractures was studied in a series of 100 patients evaluated for suspected spinal cord compression (SCC).16 Pathological fracture of the vertebral body was present in 51% of compressive levels. Of these, 68% had loss of height greater than 50%; vertebral bodies in the lower thoracic region were more likely to have pathological fractures.
A systematic approach to determining clinical instability of the spine should include anatomic, biomechanical, symptomatic, and therapeutic considerations.17,18 Apart from imaging studies that define anatomic details used in the three-column spinal model (Figure 14-1),16 the concept of clinical instability should be taken into account. Clinical instability is defined as “loss of the ability of the spine under physiologic loads to maintain relationships between vertebrae in such a way that there is either damage or subsequent irritation to the spinal cord or nerve roots, and in addition there is development of incapacitating deformity or pain due to structural changes.”18
TREATMENT OF VERTEBRAL METASTASES
Biomechanically stable vertebral metastases
Radiation therapy
Radiotherapy is effective in reducing metastatic bone pain and, in some instances, causing tumor shrinkage or growth inhibition.8,19–21 There is, as yet, no consensus regarding the most appropriate way of delivering radiotherapy for metastatic bone pain. The practice differs significantly between countries and, indeed, between treatment centers within the same country.
One of the controversies is whether single fraction radiotherapy is as effective as multifraction radiotherapy. Single fraction radiotherapy is more convenient for the patient, and it is also less costly compared with multifraction radiotherapy. However, there are some important concerns relating to single fraction therapy. The equivalent biological dose of single fraction treatment is usually smaller than the total multifraction treatment dose. As a result, the pain response to a single dose may be inferior to the multifraction radiotherapy response. Even if the initial pain response is similar, it may not be durable enough to ensure that the patient remains asymptomatic. In addition, with a potentially reduced tumoricidal effect, single fraction radiotherapy may not be as effective in preventing complications such as pathological fractures and SCC. Systematic reviews of randomized studies examining the effectiveness of single fraction radiotherapy versus multiple fraction radiotherapy pooled the results and used meta-analysis to estimate the effect of treatment on pain response, retreatment rate, and complications.8,19 There was no difference between the two radiotherapy schedules for both overall and complete pain response rates; however, patients treated by single fraction radiotherapy had a higher retreatment rate and a higher rate of pathological fractures compared with patients treated by multifraction schedule. The SCC rates were similar for both arms of the treatment schedules, although there was a trend of increasing SCC rates for patients treated by single fraction radiotherapy. Based on the above analysis of the two treatment schedules, we recommend multifraction radiotherapy for most vertebral metastases except in those patients whose life expectancy is considered to be extremely short, in which case a single fraction schedule may be appropriate for immediate pain control. The treatment schedule should be tailored for each patient based on the type of palliative therapy required and the complications that may yet develop.
Bisphosphonates
These agents work by several different mechanisms to reduce both bone resorption and bone formation.22,23 Bisphosphonates can be divided into two groups: those resembling pyrophosphate (for example, clodronate and etidronate), and the aminobisphosphonates (for example, pamidronate and zoledronic acid). The latter group inhibits enzymes of the mevalonate pathway, disrupting the signaling function of key regulatory proteins. The net effect in both groups is inhibition of osteoclast function, which leads to a decrease in bone resorption. Systematic review showed that bisphosphonates significantly decreased skeletal morbidity, and reduced the probability of vertebral fracture.3,4,9 However, the incidence of SCC was not reduced. This may be related to the fact that studies examining skeletal events were insufficiently powered to show a difference between bisphosphonates and controls for SCC, which is a relatively rare skeletal event. Based on the systematic review, recommendations are that treatment with bisphosphonate medications should start when bone metastases are diagnosed and continue until no longer clinically relevant.3
Vertebral metastases with biomechanical potential or overt instability
Vertebral metastases that lead to biomechanical spine instability, or potential instability, produce a range of symptoms and signs that should direct therapeutic consideration. Patients with localized kyphosis, collapsed vertebra, fracture-dislocation, retropulsion of a bone fragment, or segmental instability may require various surgical procedures tailored to meet decompression, stabilization, or pain relief requirements, depending on symptomatology (Figure 14-2). However, not every case defined by the criteria for unstable spine requires surgical intervention. If the tumor is relatively radiosensitive, radiotherapy may result in satisfactory axial settling and pain relief over a period of weeks or months (Figure 14-2). These patients should be carefully followed because they are still potentially unstable. Surgical procedures are reserved for symptomatic cases, because preventive surgery is probably unjustified in the management of metastatic disease of the spine.
Recommendations for patient selection and optimal methods of decompression and stabilization are constantly evolving. The criteria for spinal instability that should lead to consideration of spinal stabilization are summarized in Table 14-2.
TABLE 14-2 Categories of Spinal Instability in Metastatic Disease that Require Consideration for Spinal Fixation*
* Definition of potential instability relies on imaging studies and is valid only if the vertebral cortical shell is involved.
VERTEBRAL COMPRESSION FRACTURE IN CANCER PATIENTS
Acute vertebral compression fractures are common and may occur because of osteoporosis, neoplastic infiltration of the vertebral body, or trauma (Figure 14-3). Patients may present with osteoporotic fracture secondary to various precipitating factors such as age, steroid therapy, immobility, and prolonged use of low molecular weight heparin. Neoplastic vertebral compression is a common occurrence in both hematological malignancies and solid tumors.13,24–26
The differential diagnosis may be difficult. Imaging findings are suggestive, but often inconclusive. It has been reported, for example, that osteoporotic fractures may accumulate FDG to varying degrees, and false-positive findings may occur when FDG-PET imaging is performed to assess whether metastases are present.12,27 Accurate differentiation of simple osteoporotic fracture from pathological fracture often requires the integration of imaging findings (PET/CT combined technique and MRI) with clinical assessment. MRI findings may be useful (Figure 14-3). A convex posterior border of the vertebral body, abnormal signal intensity of the pedicle or posterior elements, epidural mass, focal paraspinal mass, and other spinal metastases are suggestive of metastatic compression fractures. In contrast, findings suggestive of osteoporotic compression fractures are: a low signal-intensity band on T1-weighted and T2-weighted images, spared normal bone marrow signal intensity of the vertebral body, retropulsion of a posterior bone fragment, multiple compression fractures, and fluid collection (the fluid sign) that results from bone marrow edema.24,25
Treatment options for vertebral compression fracture (Figure 14-2)
Vertebral compression fracture is associated with severe pain and is frequently the cause of immobility. A multidisciplinary approach to patient selection and management is essential (Figure 14-2).Once vertebral body fracture is diagnosed, it is important to evaluate whether dural displacement or significant neural compression is present. In cases with significant compression of neural elements secondary to impingement of bone, disc fragments, or tumor mass, early consideration of surgical decompression combined with stabilization should take place. In neoplastic vertebral fractures where dural displacement is present but compression is not significant, radiotherapy followed by conservative therapy can form the initial approach. Conservative treatment of thoracic or lumbar vertebral fracture includes analgesic medications with or without external bracing and encouragement to maintain weight-bearing activities. Radiotherapy is also added to metastatic vertebral fractures that are not associated with dural displacement. None of these latter modalities is uniformly effective in relieving pain or improving ambulatory status.
For patients who fail to improve with conservative management and have no evidence of cord or thecal sac compression, a minimally-invasive procedure that involves percutaneous injection of polymethylmethacrylate (PMMA), a surgical bone cement, under imaging guidance can be offered.28,29 Precise indications for these techniques are still evolving.
The goal of vertebroplasty is to provide pain relief and bone strengthening in painful vertebral body compression fracture. Selected patients with focal, intense, and intractable midline spinal pain at the level of the fracture, or within two vertebral levels below it, and who failed conservative management, are candidates for this minimally-invasive procedure. Vertebroplasty does not re-expand a collapsed vertebra, but it provides a certain degree of reinforcement and stabilization of the fracture, which alleviates pain. The procedure is relatively contraindicated for patients with evidence of dural displacement or compression,30 and suitable for patients with no radicular signs. Contraindications to the procedure include bleeding disorder and unstable fracture due to posterior element involvement. Procedural complications are relatively rare; most are related to PMMA leakage through cortical defects.29–32 Cement leakage rates range from 9% to 88%, and, in most cases, leakage is asymptomatic. Neural element compression resulting from cement extravasation remains a rare event.
Current experience with vertebroplasty and kyphoplasty for painful vertebral fractures in cancer patients remains limited, but favorable results can be obtained with careful selection of appropriate patients.28–30,33,34 Marked or complete pain relief is reported in 70% to 84% of procedures performed in neoplastic vertebral fractures, and the rate exceeds 90% in osteoporotic fractures. No change in the level of pain is reported in about 9% of neoplastic fractures. Pain relief and increased mobility are expected within 24 hours postprocedure, but occasionally these responses occur after a few days. As a result, analgesic consumption is also significantly reduced in the majority of treated patients.29 Based on current knowledge, these procedures can be applied with a good safety profile in well-selected patients with osteoporotic fractures and in patients with multiple myeloma or metastatic cancer who have refractory spinal pain.
Vertebral Metastases with Epidural Extension
Sixty percent of vertebral metastases associated with epidural extension in adults are related to breast, lung, and prostate cancer.1,35–38 Other common neoplasms include lymphoma, renal cancer, sarcoma, and multiple myeloma. The origin of metastases cannot be identified in 7% to 12% of cases.13,37,39,40 All patients with vertebral metastases are at potential risk of developing compression of the neural roots, cauda equina, and/or the spinal cord. Epidural metastases are the most common cause of spinal cord and cauda equina dysfunction in cancer patients. The term SCC is used in this chapter to include cauda equina compression, unless otherwise noted.
FREQUENCY
The incidence of SCC is unknown. Retrospective clinical studies suggest that 2% to 20% of patients with vertebral metastases develop myelopathy secondary to SCC.10,37,41 A recent population-based study of neoplastic SCC in Ontario found that the cumulative probability of experiencing at least one episode of SCC in the last five years preceding death from cancer was 2.5% and ranged from 0.2% in cancer of the pancreas to 7.9% in myeloma.37 An autopsy study estimated that 5% of cancer patients develop spinal epidural tumor deposits,42 but a proportion may remain clinically silent. SCC may present as the initial manifestation of malignancy in about 8% to 20% of patients with symptomatic epidural deposits.43,44 Carcinoma of the lung, cancer of unknown primary site, multiple myeloma, and non-Hodgkin lymphoma are disproportionately represented (accounting for 78% of episodes) in a series of SCC occurring as initial manifestation of malignancy. These primary tumors account for only 26% of SCC in patients with a previously-established diagnosis of malignancy.
The incidence of SCC secondary to lymphoma, breast, and prostate cancer has decreased over the years.45 This declining incidence probably reflects a shift in oncological treatment policies towards the early use of radiotherapy, or more effective and earlier treatment of the primary tumor reducing the rate of metastatic complications. The routine use of advanced imaging techniques (CT, PET/CT, and MRI) also contributes to early detection and treatment of clinically silent spinal metastases.1,2,12,16,39,40 Imaging of the whole spine is feasible with these techniques. When performed, for example, in patients with clinical symptomatology suggestive of SCC, whole spine images often reveal that two or more levels of compression are present, affecting more than one region of the spine. Multiple levels of neural element compression are detected in 25% to 43% of suspected SCC.2,16,39,40,46
LOCATION OF EPIDURAL TUMOR IN RELATION TO THE SPINAL CORD AND THE YIELD OF DIAGNOSTIC IMAGING
Epidural SCC usually results from metastases to one of three sites: the vertebrae, the paravertebral tissue, or the epidural space itself. Extension of the tumor into the spinal canal may produce variable involvement of the anterior (ventral) compartment, lateral gutters, posterior compartment, or any combination of these sites (Figure 14-4).
Spinal radiography is predictive of epidural disease,47 and an epidural mass is identified at 86% of symptomatic spinal segments. However, radiographic results require 30% to 70% bony destruction before they become positive. An MRI study demonstrated that, although an absent pedicle is often the first radiographic sign of metastatic disease, the pedicle is involved by direct extension from either the vertebral body or the posterior elements, and is therefore a late occurrence in the disease process.48 The region of the vertebral column that is most often involved is the vertebral body, probably because of its extensive vascular supply; thus, most epidural tumors arise in a vertebral body and invade the epidural space anteriorly.16,49 MRI evaluation of neoplastic SCC showed that metastatic disease was present in the bodies of all 160 vertebral levels that were studied.16 Assessment of vertebral quadrants’ (one anterior, two laterals and one posterior column) involvement showed that 97% of the vertebrae had more than 50% involvement. Four columns were affected in 52% and three columns in 30%. Single column involvement was seen only in the anterior column, and accounted for 3% of affected vertebrae. Coexisting anterior and posterior column disease was observed in 75%. The extensive vertebral involvement associated with SCC suggests that any attempt to perform decompressive surgery should always be combined with measures to stabilize the spine.
Normal spinal roentgenograms, seen in 6% of SCC, do not exclude epidural metastases. Normal radiographs are found in 89% of lymphoma patients with epidural compression,45 in whom a paravertebral tumor mass invades the epidural space through the intervertebral foramina, rather than via vertebral extension (Figure 14-4). This mode of epidural invasion is seen in lymphomas, renal cell cancer, superior sulcus tumors (Pancoast syndrome), and neuroblastoma, and accounts for approximately 10% of all SCC.45 Up to 36% of patients with paraspinal tumor have epidural metastases on myelography.50 With the advent of CT scanning and MRI, which adequately demonstrate paravertebral soft tissues, these lesions are being more frequently recognized. A paraspinal mass is detected by MRI at the site of SCC in 28% of patients, and only one third of the masses were detected on plain radiography.40 Pure, exclusively epidural lesions are rare, and their incidence is not known. In a consecutive series of 100 patients with SCC evaluated by MRI, no isolated epidural lesion was noted.16
The location of the extradural metastases within the vertebral canal has important surgical implications. Accurate definition of the epidural mass as posterior, lateral, cuff, or anterior (Figure 14-4) requires CT-myelography or a noninvasive MRI study. MRI is the procedure of choice to evaluate the vertebral column, spinal cord, and soft tissue parts, and usually eliminates the need for other imaging studies.39,51–53 It is the imaging method of choice both for screening and for ultimate diagnosis of SCC whenever it is readily available.2,16,39,40,54,55 Still, spinal CT is an excellent screening method to diagnose patients at risk, and to verify presence of an epidural mass in an emergency setting.1
In a study of 342 episodes of suspected neoplastic SCC evaluated with spinal CT, additional MRI assessment was required due to diagnostic uncertainty in only 5%.1 However, MRI has a broader impact on management. It detects unexpected vertebral and epidural lesions that lead to a change in treatment in up to 50% of patients.2,16,39,54,55 Thus, when SCC is suspected, a complete spine evaluation is indicated, and MRI provides significant economic benefits compared with other imaging modalities used for evaluation.56
SYMPTOMS AND SIGNS
Onset of SCC symptoms may be acute or insidious, and symptom duration varies widely. Pain is the initial symptom in 96% of cases, preceding other symptoms by approximately 2 to 3 months.35,57 Pain can be localized close to the site of the lesion or can be radicular. Pain generally results from nerve root compression or infiltration, compression fractures, segmental instability, displacement of the dura, or dural invasion. Therefore, all patients with symptomatic spinal metastases must be considered at risk for SCC. The site of pain may not correspond to the site of epidural compression on imaging,35 and it may be nonspecific or referred to other sites, frequently leading to a delay in diagnosis.35,57 This is especially true in patients without a previous history of cancer.
At the time of diagnosis, neurological signs are common,35,45,51,57 and include various degrees of muscle weakness in 76%, bladder and bowel dysfunction in over 50%, and sensory deficit in about 50% of patients with SCC. However, weakness and sensory abnormalities are often reported late and identified even later, despite patients having reported pain for a considerable time. Nearly half of severely affected patients develop a complete deficit (no residual spinal cord function) after diagnosis and before undergoing any treatment. Of the paraparetic patients, 28% become paraplegic in less than 24 hours and before initiation of treatment.58 SCC should therefore be treated as soon as possible, and early diagnosis is crucial, as functional outcome largely depends on the neurological function before treatment.45,58–60
Apart from the typical manifestations of SCC, unusual clinical presentations may be seen. These includes atypical facial pain and numbness, Brown-Séquard syndrome, ataxia secondary to posterior column dysfunction, or herpetic rash along the affected dermatomes.45,51,61
PATHOPHYSIOLOGY
The mechanisms that determine the degree of irreversible tissue damage are poorly understood, but appear to be associated with a neurochemical cascade activated by the initial event of compressive injury. Comprehension of the secondary autodestructive processes has increased with the use of well-characterized animal models.62–76 These laboratory studies demonstrate that pharmacologic treatments modify neurochemical changes, attenuate spinal cord edema, ameliorate structural destruction, and significantly delay neurologic deterioration, even if the compressing tumor is not removed. Figure 14-5 summarizes the paradigm of secondary events in neoplastic SCC, and the pharmacological strategies used to ameliorate them in experimental animal models.
The mechanism of injury induced by the expanding extradural tumors is complex and multifactorial. The extradural tumor causes early obstruction of the epidural venous plexus, and also induces arteriolar dilatation via local spinal autoregulatory mechanisms such as activation of endothelial NO synthase.64,65,77 These changes in vascular tone and drainage enhance production of a vasogenic type of edema. With increase of edema and mechanical pressure, a decrease in spinal cord blood flow at the site of compression eventually follows. Ischemia may then play the final deleterious role, leading to cell death if compression is not promptly alleviated. In animal models, development of conduction block and neurologic signs of myelopathy were related to myelin destruction,76 which was probably caused by both mechanical compression and ischemia, and by activation of death receptor pathways associated with oligodendroglial apoptosis.78 Although demyelination can occur at sites of spinal compression,79,80 remyelination may take place after transient compression,81 possibly correlating with recovery of function after prompt decompression.
Local production of cytokines such as prostaglandins, interleukin (IL)-1 and IL-6, may promote an inflammatory response with associated physiological changes of vasodilation, plasma exudation, and edema formation.75,82 In keeping with this concept, a rapid antiedema effect is achieved by steroidal or nonsteroidal antiinflammatory drugs (e.g., indomethacin),67,69,70 or by inhibitors of phagocytic activity.75,82 It was found that IL-1 induces upregulation of adhesion molecules, such as P-selectin and ICAM-1. Blockage of this process resulted in white matter preservation and improved neurological outcome.83,84
Other studies looked at the microglia and phagocytic activity. Immunohistochemical studies showed that in tumor-bearing paraplegic rats, the normal population of resting microglia was replaced by activated amoeboid cells, probably engaged in phagocytosis.75 At onset of paraplegia, marked disruption of normal neurofilament cytoarchitecture was evident. In vivo pharmacological inhibition of phagocytosis (using chloroquine and colchicines) was associated with a reduced ratio of amoeboid microglia, marked preservation of neurofilament structure, diminished synthesis of cytokines (PGE2, IL-1, IL-6), and significant attenuation in spinal cord edema. Initiating this treatment at first sign of neurologic dysfunction significantly delayed the onset of paraplegia, and protracted the course of neurological deterioration toward paraplegia.
These results suggest that inhibition of phagocytosis may delay structural damage, and thus enhance the chance of recovery following antitumor therapy. It provides the scientific background for the clinical use of steroids, and it also explains the favorable neurological outcome in patients with SCC who are treated by radiotherapy and receive high-dose dexamethasone.85 Although dexamethasone is incapable of blocking phagocytosis, it does inhibit inflammatory responses and production of some cytokines that play a role in the phagocytic cascade.
A marked increase in serotonin utilization is present in the compressed cord segments. Inhibition of serotonin receptors results in attenuated vascular permeability and a protracted clinical course toward paraplegia, similar to the favorable effect produced by antiinflammatory agents.73–75,82 Receptor-activated serotonergic mechanisms that are distinct from the mechanism associated with the inflammatory response probably participate in the disruption of the blood-spinal cord barrier in the subacutely developing compression injury. These mechanisms can be separately manipulated pharmacologically to yield measurable effects in experimental models. Finally, in the end stage, when conduction block and ischemia set in, excitotoxins (such as glutamate) mediate the evolution of cytotoxic edema that adds its deleterious role.71,72
SURVIVAL AND SPINAL CORD COMPRESSION
The magnitude of the clinical problem of SCC is usually underestimated. The best evidence suggests that approximately 5% of patients dying of cancer have suffered from epidural metastases.37,42 This calculated incidence exceeds the annual rate of traumatic spinal cord injury. However, because of the high mortality among these patients (50% to 70% at one year), the socioeconomic impact of neoplastic SCC is less than traumatic injury. The overall median survival following the first episode of SCC is short, ranging from 3 to 4 months.36,37,86 As a result, neoplastic SCC accounts for only 10% to 14% of all spinal cord injury admissions to rehabilitation units.87 It was demonstrated that pretreatment ambulatory function is the main determinant for posttreatment gait function, and the survival of nonambulatory patients can only be improved with restoration of gait function by immediate treatment.36,38,88,89
In spite of the poor prognosis of the disease, most patients should receive active treatment36,51,90 to preserve or restore mobility and continence, and to alleviate intractable pain. SCC in itself is usually not the direct cause of death, except when it occurs in the upper portion of the cervical spine. Survival in SCC is related to the natural history of the systemic malignancy. Only about 30% of patients are expected to survive beyond 1 year, and rarely as long as 4 to 9 years.43,91–93
FACTORS INFLUENCING RECOVERY OF FUNCTION
Tumor biology and cell type
The biological activity of the primary neoplasm determines the aggressiveness of both local and systemic disease, the success of therapy, and the posttreatment survival rate.37,58,94 Patients with myeloma, lymphoma, Ewing sarcoma, neuroblastoma, or carcinoma of the breast have a favorable prognosis for recovery of function, and they survive longer after the first episode of SCC.37 The outlook for patients with metastatic bronchogenic carcinoma or metastatic disease of an unknown primary site is generally poor. Overall, radiosensitivity and chemosensitivity is associated with a more favorable prognosis and functional outcome, while radioresistant tumors tend to have worse results.37,58,95
Pretreatment neurological status
SCC outcome depends in good part on the patient’s neurological status at the time of treatment. A positive correlation exists between the pretreatment mobility and functional outcome.51,58,94,96–100 From 80% to 90% of patients who can walk at the time of diagnosis remain ambulant after treatment, 35% to 45% of those who are initially paraparetic become ambulatory, and only 5% to 7% of paraplegic patients regain the ability to walk. The success of therapy in paraplegic patients depends on whether the patient has complete functional cord transection, or whether there is preservation of some neurological function, with motor function the most significant. Only 2% of patients with complete transection recover ambulation, compared to 20% of patients with residual neurological function. Regardless of the mode of therapy, complete paraplegia carries a bad functional prognosis.
The value of early diagnosis is clear, and with the advent of modern imaging techniques the percentage of patients diagnosed while still able to walk is steadily increasing. Series in which modern imaging techniques were used to diagnose SCC contain 42% to 56% ambulatory patients,85,99–101 unlike previous reports in which only 25% of patients were ambulatory at diagnosis.59,95,102,103 Nevertheless, an unacceptable delay in diagnosis, investigation, and referral occurs in many patients with neoplastic SCC, with a median delay of 14 days from the onset of symptoms. This delay results in preventable loss of function before treatment.35,104,105
Progression rate of symptoms
It has been suggested that a rapid onset and progression of neurological symptoms is associated with a worse prognosis as compared to a gradual onset and slow progression of neurological deficit.58,88,106–108 The importance of the rate of symptom progression was emphasized in a study of 15 paraplegic patients treated with radiation therapy.109 Five of the 15 patients regained ambulation after a delay of 3 months or more. The median time from the initial motor symptoms to total paralysis was longer (45 days) in patients who recovered than in those who remained paralyzed (9 days). The progression rate of motor deficit was found to be an independent prognostic factor for posttreatment functional outcome in a study that analyzed variables by multiple logistic regression analysis.88,107,108 A slower development of motor dysfunction predicted a better outcome, and was found to be a stronger predictor than other prognostic factors such as the type of tumor or the pretreatment ambulatory status. However, if the degree of spinal cord damage is taken into account, the neurological grade still has a great influence on prognosis in patients with rapidly developing symptoms (less than 24 hours).58 In patients with rapidly evolving deficit, 20% of paraparetic patients recover, compared with none of the paraplegic patients.
Therefore, residual cord function should also be taken into account as an important prognostic variable in the rapidly evolving deficit. Once paraplegia has set in, the duration of paralysis does have prognostic significance, although anecdotal recovery has been reported even after periods of 4 to 8 weeks.109
Treatment with corticosteroids
The use of steroids in the treatment of SCC is widely accepted.58,60,85,95,103,110 The scientific basis for steroid use stems from animal models of SCC, in which reduction of spinal cord edema and delayed onset of paraplegia were observed after treatment with dexamethasone.62,64,66,67,69,75 The clinical basis for use of high-dose steroids is the rapid symptom relief noted in some patients receiving initial doses of 100 mg of dexamethasone, although the outcome was not superior compared to historical controls who received lower doses of steroids.95 In a prospective study, 37 patients with SCC were randomly assigned to receive initial treatment with either high-dose dexamethasone (100 mg) or a conventional intravenous bolus dose (10 mg) of dexamethasone followed by 16 mg/day orally.110 No dose effect was observed on neurological outcome, but a substantial effect on pain was noted within 24 hours after high-dose treatment. There is only one well-designed, randomized, controlled trial that compared high-dose dexamethasone to no steroids in 57 patients with metastatic SCC treated with radiotherapy alone.85 There was a statistically and clinically significant difference between the steroid-treated and control arms. Of patients receiving steroids, 81% were ambulatory after treatment, compared to only 63% of patients in the control arm. However, steroid treatment was associated with significant side effects in 11%.
Several investigators observed a dramatic resolution of symptoms after treatment with steroids alone.45,111,112 Such steroid-related improvement probably results from a direct oncolytic effect observed in lymphomas and leukemias. This response differs from the pharmacologic effect of steroids that protracts the cascade of deleterious events leading to irreversible spinal cord damage, which are demonstrated in Figure 14-5.
PRINCIPLES OF MANAGEMENT
The management of metastatic SCC is controversial.58–60,94,99,100,113,114 Controversy stems from the lack of prospective, well-designed, controlled clinical studies directly comparing various treatment modalities, and from an absence of standard criteria for evaluation of response. Patients with metastatic SCC have, by definition, tumors that have spread from a primary site and thus are no longer curable by local measures. The therapeutic modalities of surgery and radiotherapy are palliative, and preservation or restoration of ambulation and bladder control are the criteria for successful therapy. Pain relief is also an important, but secondary goal.
Because the treatment is not curative, its effect on the quality of further survival is particularly important. The therapeutic modalities currently available for the treatment of patients with epidural SCC are listed in Table 14-3. An algorithm for treatment selection in the various categories of SCC is shown in Figure 14-6.
TABLE 14-3 Therapeutic Modalities in Spinal Epidural Metastases
Pharmacotherapy
Radiation Therapy Surgery |
Pharmacotherapy
Specific chemotherapy
Epidural metastases arising from chemoresponsive primary tumors are likely to respond to chemotherapy, particularly early in the course of the disease. The response of lymphomas affecting the spine to corticosteroids45 may serve as an example. Several reports suggest that good recovery of neurological function can be obtained by chemotherapy alone in chemosensitive neoplasms such as lymphoma, myeloma, Ewing sarcoma, germ cell tumors, and neuroblastoma.114–119 The published experience of chemotherapy alone is limited and large-scaled studies are not available.
Nonspecific pharmacotherapy
The following recommendations apply for the use of steroids:
Radiation therapy
The results of radiation therapy in 1211 patients with a variety of metastatic tumors36,43,45,85,95,96,99,101,125–129 can be summarized as follows: Between 28% and 50% of patients either regain or maintain ambulation at the end of therapy, and, of those who survive one year, 50% maintain that improvement.45,95 The proportion of patients who are ambulatory at diagnosis is increasing, and they comprise 42% to 64% of patients diagnosed with SCC.36,45,85,99,101 Up to 96% of patients who are ambulatory at diagnosis are likely to maintain mobility. However, 4% to 20% of patients who can walk at the start of treatment will deteriorate to nonambulatory status during or soon after completion of radiotherapy. Radiation therapy also results in pain relief in 50% to 80% of patients.36,45,49,51,94,98,99
The efficacy of radiation therapy depends on the radiosensitivity of the tumor, the neurological status at time of treatment, and maintenance of spinal stability. Patients with highly radiosensitive tumors (such as lymphoma, Ewing sarcoma, seminoma, neuroblastoma, and myeloma) are more likely to regain or maintain ambulation than those with less radiosensitive tumors (overall, 60% to 80% versus 40%).49,58,103,112,114 Improvement after the initiation of radiation therapy may be delayed for a few days before it becomes evident, even in patients with radiosensitive tumors.
Radiotherapy should also be considered as primary therapy for moderately radiosensitive neoplasms, such as breast carcinoma. However, the need for surgical intervention should be critically reviewed for each patient. Radiotherapy is considered as the treatment of first choice for patients who are ambulatory at presentation, and whose spinal stability is maintained. However, in nonambulatory patients, surgical decompression should be considered as the primary avenue of therapy because radiotherapy requires a time interval that may cause an irreversible delay in the reduction of spinal cord damage. A randomized study assigned patients with SCC to primary treatment with either radiotherapy alone or to surgical decompression with stabilization followed by postoperative radiotherapy.130 Patients treated by immediate direct surgical decompressive surgery plus radiotherapy retained the ability to walk longer and regained the ability to walk more often than patients treated by radiotherapy alone. Radiotherapy alone should be employed if surgery is contraindicated because of the patient’s poor general medical status, multiple compression levels, or long-standing paraplegia.
No controlled studies have compared the various dose and fractionation schedules of radiation therapy given to patients with SCC.60 Single-arm studies and retrospective reviews of nonrandomized studies108,127,131–133 suggest that neither the dose nor the fractionation schedule has a major impact on outcome. The chosen treatment schedule should be a short palliative regimen at doses below spinal cord tolerance. The frequently used schedules are 20 Gy in five fractions, 28 Gy in seven fractions, and 30 Gy in ten fractions.
Surgery
Surgical treatment of SCC has been a subject of debate for a long time. A recent randomized trial compared direct decompressive surgical resection plus radiotherapy to treatment with radiotherapy alone.130 This trial proved that primary treatment with surgical decompression and stabilization yields a better outcome than treatment with radiotherapy alone. Prior to this study, surgical series suggested that a high proportion of patients regain or maintain ambulation (approximately 75%).45,97,100,103,134–147 However, the risk associated with operative morbidity (average rate 14%) and mortality (4.5%) should be carefully weighed against the expected gain from surgery. Surgery is usually indicated for patients with SCC who have an expected survival that exceeds 3 to 6 months. This expected survival is longer than the overall median survival of patients with SCC, which ranges between 3 and 4 months.36,37,86
The traditional approach to the treatment of neoplastic SCC has been decompressive laminectomy, with or without postoperative radiation therapy.41,43,45,85,95,96,99,101,102,112,125–127,141 Ambulation following laminectomy is maintained or regained in 35% to 50% of treated patients, a result similar to that achieved by radiation therapy alone. Pain is improved in 50% to 70% of patients, a rate which is also similar to that for radiation therapy. Approximately 14% of patients treated by radiotherapy can deteriorate,36,43,45,85,95,96,125–129 compared to approximately 15% of the patients reported to have worse neurological outcome after laminectomy. Thus, results of laminectomy used as a decompressive surgery are similar to those reported for radiation therapy alone. Therefore, oncology literature favored initial radiotherapy in the majority of the patients, reserving surgery for salvage or diagnosis.
Indications for surgical intervention
Figure 14-6 specifies the circumstances in which surgery should be considered.
Diagnosis in doubt
In 8% of patients with neoplastic SCC, spinal involvement is the initial presentation of cancer.44,102 When the etiology of the spinal lesion is in doubt, surgical decompression should be considered to obtain a tissue diagnosis and to achieve rapid decompression. Current image-assisted (CT-guided) percutaneous needle biopsy of vertebral lesions is diagnostic in 95% of patients44,148 and has a reported complication rate of less than 1%. Therefore, surgery is rarely needed simply to establish the diagnosis of malignancy. If the biopsy shows a chemosensitive tumor (e.g., lymphoma) the patient can immediately proceed to treatment without the delay imposed by surgery. If the biopsy results are not diagnostic, or if the patient’s ability to walk is already impaired and neurological status is unstable, decompression is advisable to achieve an accurate diagnosis and to initiate treatment without delay.
Spinal instability or bone compression:
A major goal of treatment is the restoration or maintenance of spinal stability; therefore, it is essential to diagnose spinal instability. The criteria for spinal stability in neoplastic disease of the spine are specified in section 2.1 of this chapter. Apart from the neuroimaging studies that define anatomic details used in the three-column spinal model (Figure 14-1), the concept of clinical instability should be taken into account.18 Clinical instability should be suspected whenever an incapacitating pain develops under physiologic loads and is associated with structural changes identified on imaging. Thus symptoms and signs should direct therapeutic consideration. Patients with fracture-dislocation, localized kyphosis, collapsed vertebra with retropulsion of a bone fragment, or segmental instability, may require surgical decompression and stabilization if either pain or neurological symptomatology is present. Not every case defined by the criteria for unstable spine requires surgical intervention. If the tumor is relatively radiosensitive, and no neurological deficit is present, radiotherapy may result in satisfactory axial settling and pain relief over a period of weeks or months. These patients should be carefully followed, because they are potentially unstable.
The criteria of spinal instability that should bring about consideration for spinal stabilization are summarized in Table 14-2. The criteria for patient selection, the surgical approach for decompression, and the methods of stabilization vary. Recommendations for patient selection and optimal methods of decompression and stabilization are undergoing constant development.
Previous radiation exposure:
When radiotherapy cannot be used because of previous irradiation, surgical decompression is indicated as the primary therapeutic modality because of the risk of exceeding spinal cord tolerance by further irradiation. Surgery is warranted for relapse occurring months or even years after a successful previous treatment, in the hope of preserving neurological function. In many patients, however, life expectancy at the time of relapse of SCC is short. Retreatment with radiotherapy often preserves ambulation with a low risk of radiation myelopathy during the remaining lifespan.131,149–151
Selection of surgical approach
The goal of surgery is the optimal removal of the compressing epidural mass and restoration of spinal stability. Therefore, the selection of the surgical approach should be determined by variables such as the location of the tumor inside and outside the spinal canal, the main cause of the instability, and feasible options for stabilization. Table 14-4 details the preoperative assessment required for determining the surgical approach. The location of the epidural mass should dictate the approach for decompression. Primary spinal tumors are classified based on tumor location and according to the zones of involvement (McLain and Weinstein classification),152 and this information is used to determine the optimal surgical approach (Figure 14-7). These guidelines are also applicable to metastatic lesions.
TABLE 14-4 Pretreatment or Preoperative Assessment of Tumor Location and Extent of Pathology in Tumors of the Spine
Bone involvement (CT, MRI)
Epidural mass (CT myelography, MRI) Spinal stability (spinal radiography, CT and MRI) Retroperitoneal and/or paravertebral mass (CT, MRI) |
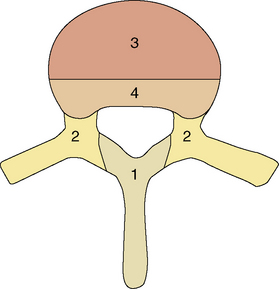
Figure 14-7 Classification system of McLain and Weinstein152 suggested for determining the optimal surgical approach for resection of spinal tumors. The spine is divided into four zones of possible tumor involvement. Zone 1 is best approached posteriorly. Zone 2 tumors may be resected via a posterior or posterolateral approach. Zone 3 tumors are best approached anteriorly. Zone 4 is the most inaccessible region, hence a combined anterior and posterior approach may be recommended.
In patients with metastatic spine disease and limited life expectancy, radical excision of the vertebral tumor is not always the main goal; therefore, selection of surgical approach also requires other considerations. In patients with progressive kyphosis without anterior cord compression, or in patients with thoracic or lumbar pathological fracture or dislocation without significant soft tissue or bony neural compromise, posterior realignment and stabilization may relieve pain and most cord impingement. In patients for whom the anterior approach is not feasible due to technical reasons, poor medical status, or widespread disease, partial decompression and stabilization via a posterior approach may be a useful palliative procedure.153
COMPLICATIONS
Radiotherapy
Most papers dealing with radiotherapy alone do not comment on complications of therapy or mortality. Death in the first month after radiotherapy is most likely the result of active primary or metastatic disease. The main determinants of survival are performance status and tumor burden; 70% of patients considered severely ill at the time of SCC diagnosis were reported to die within 30 days after diagnosis.103 The risk of radiation myelopathy is related to dose/fractionation parameters, which, in palliative treatment, are below the level of spinal cord tolerance. The proportion of patients with neoplastic SCC surviving for more than 2 years is less than 3%, and these patients are at risk of developing radiation myelopathy.131 Of patients who survive for more than 18 months, 5% may develop symptomatic myelopathy.131
Surgical complications
Mortality
The incidence of death within the first month after decompressive laminectomy is approximately 8%.45,154–157 The surgical mortality rate after vertebral body resection of 4% to 8% falls within the same range.45,97,100,103,134–137,142,144,145
Morbidity
The risk of neurological deterioration as a result of surgery is a major concern in patients who present while they are still able to walk. Its average rate following laminectomy is 15% (range: 5% to 30%).45,93,158,159 Neurological deterioration is rare after vertebral body resection, and occurs in only 2% to 6% of patients.97,103,134,160
Nonneurological complications in patients undergoing laminectomy combined with radiotherapy include wound infection, wound dehiscence, spinal epidural hematoma, cerebrospinal fluid leak, and spinal instability. The frequency of these complications ranges from 8% to 54%, with a mean of 12%.45,59 Only a few authors mentioned the problem of instability,45,102 which amounts to about 9% of cases.
As the use of instrumentation increases, nonneurological complications, especially instrumentation-related complications such as dislodgment and infections, are increasing (Table 14-5). Preoperative protein depletion and perioperative administration of corticosteroids are risk factors for wound infection.144,161 The likelihood of postoperative complications is significantly increased in patients with poor neurological outcome, and in patients who develop severe motor deficit prior to surgery.144 Spinal radiation before surgical decompression is associated with a significantly higher rate of major wound complications.162,163 Patients with preoperative irradiation had a 32% major wound complication rate, which was threefold higher than the 12% rate observed in patients who underwent de novo surgery.163 It should be noted that a tailored, patient-specific approach, including vertebral body resection and spinal instrumentation techniques, requires considerable expertise. These procedures should be undertaken only by a surgeon familiar with the specific techniques for posterior and anterior spinal instrumentation, including the different options to suit specific levels along the spine.
TABLE 14-5 Surgery-related Nonneurological Morbidity in Neoplastic Spinal Cord Compression
Surgical Procedure | Morbidity (%) | |
---|---|---|
Range | Average | |
Laminectomy | 8-54 | 15 |
Laminectomy and fixation | 1-21 | 11 |
Vertebral body resection and fixation | 8-41 | 23 |
Patient-specific approach (anterior, posterior or both) and fixation | 25-48 | 37 |
RECURRENT EPIDURAL SCC
About 10% of patients will eventually develop local recurrence of spinal metastasis. Most of these patients have received radiotherapy and some have had previous surgery. Little attention has been paid to this subgroup of patients. For the majority, no reliably effective chemotherapy option remains at this stage. Spinal surgery should be considered in these patients, although many have advanced visceral disease limiting their life expectancy to a few months, or widespread spinal metastases, which limit surgical feasibility. Surgical decompression after previous radiotherapy is associated with an increased rate of morbidity.162,163 A retrospective study of 51 patients undergoing a second course of spinal radiation found that reirradiation was highly successful in preserving neurologic function and rarely resulted in radiation myelopathy.149
Intradural Metastases
Metastatic disease within the confines of the dura constitutes less than 5% of all spinal metastases. Intradural metastases that affect the spinal cord are categorized into those located in the subarachnoid space, causing intradural SCC (Figure 14-8a), and intramedullary metastases that invade the cord substance (Figure 14-8b).
INTRADURAL EXTRAMEDULLARY METASTASES
Metastatic tumors involving the subarachnoid space are by definition leptomeningeal metastases. Unlike the multifocal manifestations produced by diffuse seeding of the subarachnoid space, a metastatic spread may present as a single intradural extramedullary mass lesion compressing the spinal cord.164–166 The clinical features of these metastases are essentially identical to those of patients with epidural SCC. Primary tumors in a series of ten patients included breast, lung, melanoma, and uterine neoplasm in a descending order of frequency.165 Of the ten tumors, nine appeared to have spread from brain metastases via the cerebrospinal fluid pathway; in another series brain metastases anteceded the spinal spread in 80% of cases.164
INTRAMEDULLARY METASTASES
Intramedullary spinal metastases arise either from hematogenous spread to the spinal parenchyma, or by growth of subarachnoid tumor along nerve roots directly into the spinal cord.167 Intramedullary metastases comprise 8.5% of all CNS metastases, and were found in 2% of cancer patients who had autopsy. Lung cancer is a common primary tumor, accounting for approximately 50% of these metastases,5,7,45,167,168 breast cancer contributes about 15%, and lymphoma and melanoma each account for another 10% of cases.5,45,167,168 In two series, intramedullary metastases were the initial manifestation of cancer in 30% of cases.5,169
Disease at other levels of the neuroaxis is common in patients with intramedullary metastases;5,6,168 approximately 50% of patients have brain metastases and 30% have multiple spinal cord metastases. Brain metastases are almost invariably diagnosed prior to the intramedullary lesion, although sometimes the diagnosis is simultaneous. Leptomeningeal seeding coexists in 15% to 44% of patients, and one autopsy study showed that seeding was associated with focal or multifocal direct extension of the leptomeningeal tumor across the pia into the parenchyma of the spinal cord.167
The clinical features of intramedullary metastases include pain in approximately 50% of patients, sensory deficit in more than 80%, weakness in 90%, and urinary incontinence in more than 60%.5,45,168 None of these features reliably differentiates intramedullary metastases from epidural SCC. However, markedly asymmetric neurologic findings (Brown-Séquard or pseudo−Brown-Séquard syndrome), reported in approximately 30% of cases, are suggestive of an intramedullary rather than extramedullary lesion.
Contrast-enhanced MRI is the ultimate diagnostic examination, but when MRI is contraindicated a CT-myelography should be performed.5,6 Myelography is less sensitive than MRI and yields a positive diagnosis in only 50% to 80% of symptomatic cases.5,45 MRI shows that intramedullary metastases are most commonly isolated, small, oval-shaped lesions, without or with slight deformation of the spinal profile. The lesions are enhancing with contrast, and on T2-weighted sequences pencil-shaped hyperintensity frequently extends cranial to the lesion.6 Lumbosacral myelomeres are the most frequently affected segments, but involvement of the thoracic and cervical myelomeres is often encountered. Because multiple spinal lesions are found in approximately one third of patients, the MRI study should encompass the entire spine or the whole neuroaxis. Differential diagnoses include primary intramedullary tumors (e.g., glioma or ependymoma), radiation myelopathy in previously irradiated patients, spinal cord infarction from embolus or vascular occlusion, and paraneoplastic necrotic myelopathy. In patients with no history of malignancy, the differential diagnosis also includes myelitis or acute demyelinating plaque. Imaging evaluation of the whole neuroaxis combined with a careful history and neurological examination may aid in the differential diagnosis.
Intramedullary metastases commonly develop late in the course of malignancy. The median survival from diagnosis of the intramedullary lesion is approximately 4 months.5 Patients with radiosensitive or relatively radiosensitive neoplasms may respond to radiotherapy. Most patients who are ambulatory when diagnosed will experience durable stabilization of function following radiotherapy.
In a small subset of patients surgical resection of an intramedullary lesion should be considered. This is frequently the case when the intramedullary lesion is the first manifestation of malignancy. As with brain metastases, intramedullary deposits are frequently circumscribed, with little invasion of the surrounding spinal medulla, and are thus amenable for gross total resection. In patients with a known diagnosis of cancer, radiation may be considered when the metastasis is isolated, and when the mode of spread is likely to be hematogenous rather than via the subarachnoid space. A few patients who underwent surgical removal of intramedullary metastases are reported to have long-term survival with good neurologic function after treatment.170–173
1. J.A. Talcott, P.C. Stomper, F.W. Drislane, P.Y. Wen, C.C. Block, C.C. Humphrey, et al. Assessing suspected spinal cord compression: a multidisciplinary outcomes analysis of 342 episodes. Support Care Cancer. 1999;7(1):31-38.
2. A.M. Cook, T.N. Lau, M.J. Tomlinson, M. Vaidya, C.J. Wakeley, P. Goddard. Magnetic resonance imaging of the whole spine in suspected malignant spinal cord compression: impact on management. Clin Oncol (R Coll Radiol). 1998;10(1):39-43.
3. J.R. Ross, Y. Saunders, P.M. Edmonds, S. Patel, K.E. Broadley, S.R. Johnston. Systematic review of role of bisphosphonates on skeletal morbidity in metastatic cancer. BMJ. 2003;327(7413):469.
4. N. Pavlakis, M. Stockler. Bisphosphonates for breast cancer. Cochrane Database Syst Rev. (1):2002. CD003474
5. D. Schiff, B.P. O’Neill. Intramedullary spinal cord metastases: clinical features and treatment outcome. Neurology. 1996;47(4):906-912.
6. S. Crasto, S. Duca, O. Davini, L. Rizzo, I.G. Pavanello, T. Avataneo, et al. MRI diagnosis of intramedullary metastases from extra-CNS tumors. Eur Radiol. 1997;7(5):732-736.
7. A. Potti, M. Abdel-Raheem, R. Levitt, D.A. Schell, S.A. Mehdi. Intramedullary spinal cord metastases (ISCM) and non-small cell lung carcinoma (NSCLC): clinical patterns, diagnosis and therapeutic considerations. Lung Cancer. 2001;31(2–3):319-323.
8. M.S. Wai, S. Mike, H. Ines, M. Malcolm. Palliation of Metastatic Bone Pain: Single Fraction versus Multifraction Radiotherapy−A Systematic Review of the Randomised Trials. Cochrane Database Syst Rev. (2):2004. CD004721
9. L.S. Rosen, D. Gordon, S. Tchekmedyian, R. Yanagihara, V. Hirsh, M. Krzakowski, et al. Zoledronic acid versus placebo in the treatment of skeletal metastases in patients with lung cancer and other solid tumors: a phase III, double-blind, randomized trial—the Zoledronic Acid Lung Cancer and Other Solid Tumors Study Group. J Clin Oncol. 2003;21(16):3150-3157.
10. J. Schaberg, B.J. Gainor. A profile of metastatic carcinoma of the spine. Spine. 1985;10(1):19-20.
11. H.L. Abrams, R. Spiro, N. Goldstein. Metastases in carcinoma. Analysis of 1000 autopsied cases. Cancer. 1950;3:74-85.
12. U. Metser, H. Lerman, A. Blank, G. Lievshitz, F. Bokstein, E. Even-Sapir. Malignant involvement of the spine: assessment by 18F-FDG PET/CT. J Nucl Med. 2004;45(2):279-284.
13. B.C. Vande Berg, F.E. Lecouvet, L. Michaux, A. Ferrant, B. Maldague, J. Malghem. Magnetic resonance imaging of the bone marrow in hematological malignancies. Eur Radiol. 1998;8(8):1335-1344.
14. F. Denis. Spinal instability as defined by the three-column spine concept in acute spinal trauma. Clin Orthop. 1984;189:65-76.
15. J.P. Kostuik, J.N. Errico. J.W. Frymoyer, editor. Differential diagnosis and surgical treatment of metastatic spine tumors. New York: Raven Press. 1991:861-888.
16. F.M. Khaw, S.A. Worthy, M.J. Gibson, A. Gholkar. The appearance on MRI of vertebrae in acute compression of the spinal cord due to metastases. J Bone Joint Surg Br. 1999;81(5):830-834.
17. D.S. Bradford. Spinal instability: orthopedic perspective and prevention. Clin Neurosurg. 1980;27:591-610.
18. A.A.I. White, M.M. Panjabi. Clinical biomechanics of the spine. Philadelphia: Lippincott J. B, 1987;191-276.
19. W.M. Sze, M.D. Shelley, I. Held, T.J. Wilt, M.D. Mason. Palliation of metastatic bone pain: single fraction versus multifraction radiotherapy—a systematic review of randomised trials. Clin Oncol (R Coll Radiol). 2003;15(6):345-352.
20. T. Saarto, R. Janes, M. Tenhunen, M. Kouri. Palliative radiotherapy in the treatment of skeletal metastases. Eur J Pain. 2002;6(5):323-330.
21. B. Jeremic. Single fraction external beam radiation therapy in the treatment of localized metastatic bone pain. A review. J Pain Symptom Manage. 2001;22(6):1048-1058.
22. H. Fleisch. Development of bisphosphonates. Breast Cancer Res. 2002;4(1):30-34.
23. H. Fleisch. Bisphosphonates in osteoporosis. Eur Spine J. 2003.
24. A. Baur, A. Stabler, S. Arbogast, H.R. Duerr, R. Bartl, M. Reiser. Acute osteoporotic and neoplastic vertebral compression fractures: fluid sign at MR imaging. Radiology. 2002;225(3):730-735.
25. H.S. Jung, W.H. Jee, T.R. McCauley, K.Y. Ha, K.H. Choi. Discrimination of metastatic from acute osteoporotic compression spinal fractures with MR imaging. Radiographics. 2003;23(1):179-187.
26. J.K. Kim, T.J. Learch, P.M. Colletti, J.W. Lee, S.D. Tran, M.R. Terk. Diagnosis of vertebral metastasis, epidural metastasis, and malignant spinal cord compression: are T(1)-weighted sagittal images sufficient? Magn Reson Imaging. 2000;18(7):819-824.
27. I.H. Shon, I. Fogelman. F-18 FDG positron emission tomography and benign fractures. Clin Nucl Med. 2003;28(3):171-175.
28. W.C. Peh, L.A. Gilula. Percutaneous vertebroplasty: indications, contraindications, and technique. Br J Radiol. 2003;76(901):69-75.
29. D.R. Fourney, D.F. Schomer, R. Nader, J. Chlan-Fourney, D. Suki, K. Ahrar, et al. Percutaneous vertebroplasty and kyphoplasty for painful vertebral body fractures in cancer patients. J Neurosurg. 2003;98(1 Suppl):21-30.
30. N.B. Appel, L.A. Gilula. Percutaneous vertebroplasty in patients with spinal canal compromise. AJR Am J Roentgenol. 2004;182(4):947-951.
31. J.S. Yeom, W.J. Kim, W.S. Choy, C.K. Lee, B.S. Chang, J.W. Kang. Leakage of cement in percutaneous transpedicular vertebroplasty for painful osteoporotic compression fractures. J Bone Joint Surg Br. 2003;85(1):83-89.
32. P. Mousavi, S. Roth, J. Finkelstein, G. Cheung, C. Whyne. Volumetric quantification of cement leakage following percutaneous vertebroplasty in metastatic and osteoporotic vertebrae. J Neurosurg. 2003;99(1 Suppl):56-59.
33. L. Alvarez, A. Perez-Higueras, D. Quinones, E. Calvo, R.E. Rossi. Vertebroplasty in the treatment of vertebral tumors: postprocedural outcome and quality of life. Eur Spine J. 2003;12(4):356-360.
34. J.V. Coumans, M.K. Reinhardt, I.H. Lieberman. Kyphoplasty for vertebral compression fractures: 1-year clinical outcomes from a prospective study. J Neurosurg. 2003;99(1 Suppl):44-50.
35. P. Levack, J. Graham, D. Collie, R. Grant, J. Kidd, I. Kunkler, et al. Don’t wait for a sensory level—listen to the symptoms: a prospective audit of the delays in diagnosis of malignant cord compression. Clin Oncol (R Coll Radiol). 2002;14(6):472-480.
36. O.O. Zaidat, R.L. Ruff. Treatment of spinal epidural metastasis improves patient survival and functional state. Neurology. 2002;58(9):1360-1366.
37. D.A. Loblaw, N.J. Laperriere, W.J. Mackillop. A population-based study of malignant spinal cord compression in Ontario. Clin Oncol (R Coll Radiol). 2003;15(4):211-217.
38. S. Helweg-Larsen, P.S. Sorensen, S. Kreiner. Prognostic factors in metastatic spinal cord compression: a prospective study using multivariate analysis of variables influencing survival and gait function in 153 patients. Int J Radiat Oncol Biol Phys. 2000;46(5):1163-1169.
39. D. Schiff, B.P. O’Neill, C.H. Wang, J.R. O’Fallon. Neuroimaging and treatment implications of patients with multiple epidural spinal metastases. Cancer. 1998;83(8):1593-16601.
40. D.J. Husband, K.A. Grant, C.S. Romaniuk. MRI in the diagnosis and treatment of suspected malignant spinal cord compression. Br J Radiol. 2001;74(877):15-23.
41. C.A. Cobb3rd, M.E. Leavens, N. Eckles. Indications for nonoperative treatment of spinal cord compression due to breast cancer. J Neurosurg. 1977;47(5):653-658.
42. K.D. Barron, A. Hirano, S. Araki, R.D. Terry. Experiences with metastatic neoplasms involving the spinal cord. Neurology. 1959;9(2):91-106.
43. F. Bach, B.H. Larsen, K. Rohde, S.E. Borgesen, F. Gjerris, T. Boge-Rasmussen, et al. Metastatic spinal cord compression. Occurrence, symptoms, clinical presentations and prognosis in 398 patients with spinal cord compression. Acta Neurochir. 1990;107(1–2):37-43.
44. D. Schiff, B.P. O’Neill, V.J. Suman. Spinal epidural metastasis as the initial manifestation of malignancy: clinical features and diagnostic approach. Neurology. 1997;49(2):452-456.
45. J.B. Posner. J.B. Posner, editor. Spinal metastases. Philadelphia: Davis, F. A. Company. 1995:111-142.
46. M.C. Chamberlain, P.A. Kormanik. Epidural spinal cord compression: a single institution’s retrospective experience. Neuro-oncol. 1999;1(2):120-123.
47. R.K. Portenoy, B.S. Galer, O. Salamon, M. Freilich, J.E. Finkel, D. Milstein, et al. Identification of epidural neoplasm. Radiography and bone scintigraphy in the symptomatic and asymptomatic spine. Cancer. 1989;64(11):2207-2213.
48. P.L. Asdourian, M. Weidenbaum, R.L. DeWald, K.W. Hammerberg, R.G. Ramsey. The pattern of vertebral involvement in metastatic vertebral breast cancer. Clin Orthop. 1990;250:164-170.
49. T. Siegal. A. Twijnstra, A. Keyser, B.W. Ongerboer de Visser, editors. Spinal epidural metastases from solid tumors. Clinical diagnosis and management. The Netherlands: Elsevier Science Publications. 1993:283-305.
50. F. Graus, G. Krol, M. FK. Early diagnosis of spinal epidural metastases: correlation with clinical and radiological findings (abstr). 1985. Conference
51. T.N. Byrne. Spinal cord compression from epidural metastases. N Engl J Med. 1992;327(9):614-619.
52. R.F. Carmody, P.J. Yang, G.W. Seeley, J.F. Seeger, E.C. Unger, J.E. Johnson. Spinal cord compression due to metastatic disease: diagnosis with MR imaging versus myelography. Radiology. 1989;173(1):225-229.
53. P.M. Colletti, H.T. Dang, M.W. Deseran, R.M. Kerr, W.D. Boswell, P.W. Ralls. Spinal MR imaging in suspected metastases: correlation with skeletal scintigraphy. Magn Reson Imaging. 1991;9(3):349-355.
54. U. Heldmann, P.S. Myschetzky, H.S. Thomsen. Frequency of unexpected multifocal metastasis in patients with acute spinal cord compression. Evaluation by low-field MR imaging in cancer patients. Acta Radiol. 1997;38(3):372-375.
55. P.M. Colletti, H.J. Siegel, M.Y. Woo, H.Y. Young, M.R. Terk. The impact on treatment planning of MRI of the spine in patients suspected of vertebral metastasis: an efficacy study. Comput Med Imaging Graph. 1996;20(3):159-162.
56. J.E. Jordan, S.S. Donaldson, D.R. Enzmann. Cost effectiveness and outcome assessment of magnetic resonance imaging in diagnosing cord compression. Cancer. 1995;75(10):2579-2586.
57. A. Solberg, R.M. Bremnes. Metastatic spinal cord compression: diagnostic delay, treatment, and outcome. Anticancer Res. 1999;19(1B):677-684.
58. A. Barcena, R.D. Lobato, J.J. Rivas, F. Cordobes, S. de Castro, A. Cabrera, et al. Spinal metastatic disease: analysis of factors determining functional prognosis and the choice of treatment. Neurosurgery. 1984;15(6):820-827.
59. G.F. Findlay. Adverse effects of the management of malignant spinal cord compression. J Neurol Neurosurg Psychiatry. 1984;47(8):761-768.
60. D.A. Loblaw, N.J. Laperriere. Emergency treatment of malignant extradural spinal cord compression: an evidence-based guideline. J Clin Oncol. 1998;16(4):1613-1624.
61. B. Hainline, M.H. Tuszynski, J.B. Posner. Ataxia in epidural spinal cord compression. Neurology. 1992;42(11):2193-2195.
62. Y. Ushio, R. Posner, J.H. Kim, W.R. Shapiro, J.B. Posner. Treatment of experimental spinal cord compression caused by extradural neoplasms. J Neurosurg. 1977;47(3):380-390.
63. Y. Ushio, R. Posner, J.B. Posner, W.R. Shapiro. Experimental spinal cord compression by epidural neoplasm. Neurology. 1977;27(5):422-429.
64. H. Ikeda, Y. Ushio, T. Hayakawa, H. Mogami. Edema and circulatory disturbance in the spinal cord compressed by epidural neoplasms in rabbits. J Neurosurg. 1980;52(2):203-209.
65. A. Kato, Y. Ushio, T. Hayakawa, K. Yamada, H. Ikeda, H. Mogami. Circulatory disturbance of the spinal cord with epidural neoplasm in rats. J Neurosurg. 1985;63(2):260-265.
66. J.Y. Delattre, E. Arbit, H.T. Thaler, M.K. Rosenblum, J.B. Posner. A dose-response study of dexamethasone in a model of spinal cord compression caused by epidural tumor. J Neurosurg. 1989;70(6):920-925.
67. T. Siegal, Y. Shapira, U. Sandbank, R. Catane. Indomethacin and dexamethasone treatment in experimental neoplastic spinal cord compression: Part 1. Effect on water content and specific gravity. Neurosurgery. 1988;22(2):328-333.
68. T. Siegal, E. Shohami, Y. Shapira. Indomethacin and dexamethasone treatment in experimental neoplastic spinal cord compression: Part 2. Effect on edema and prostaglandin synthesis. Neurosurgery. 1988;22(2):334-339.
69. T. Siegal, E. Shohami, Y. Shapira. Comparison of soluble dexamethasone sodium phosphate with free dexamethasone and indomethacin in treatment of experimental neoplastic spinal cord compression. Spine. 1988;13(10):1171-1176.
70. T. Siegal, T. Siegal, Y. Shapira, E. Shohami. The early effect of steroidal and non-steroidal anti-inflammatory agents on neoplastic epidural cord copmpression. Ann NY Acad Sci. 1989;559:488-490.
71. T. Siegal, F. Lossos. Experimental neoplastic spinal cord compression: effect of anti-inflammatory agents and glutamate receptor antagonists on vascular permeability. Neurosurgery. 1990;26(6):967-970.
72. T. Siegal, E. Shohami, F. Lossos. Experimental neoplastic spinal cord compression: effect of ketamine and MK-801 on edema and prostaglandins. Neurosurgery. 1990;26(6):963-966.
73. T. Siegal. Participation of serotonergic mechanisms in the pathophysiology of experimental neoplastic spinal cord compression. Neurology. 1991;41(4):574-580.
74. T. Siegal. Serotonergic manipulations in experimental neoplastic spinal cord compression. J Neurosurg. 1993;78(6):929-937.
75. T. Siegal. Spinal cord compression: from laboratory to clinic. Eur J Cancer. 1995;31A(11):1748-1753.
76. T. Siegal, T.Z. Siegal, U. Sandbank, E. Shohami, J. Shapira, J.M. Gomori, et al. Experimental neoplastic spinal cord compression: evoked potentials, edema, prostaglandins, and light and electron microscopy. Spine. 1987;12(5):440-448.
77. M. Ishikawa, E. Sekizuka, B. Krischek, U. Sure, R. Becker, H. Bertalanffy. Role of nitric oxide in the regulation of spinal arteriolar tone. Neurosurgery. 2002;50(2):371-377. discussion 377–8
78. S. Casha, W.R. Yu, M.G. Fehlings. Oligodendroglial apoptosis occurs along degenerating axons and is associated with FAS and p75 expression following spinal cord injury in the rat. Neuroscience. 2001;103(1):203-218.
79. A.R. Blight, V. Decrescito. Morphometric analysis of experimental spinal cord injury in the cat: the relation of injury intensity to survival of myelinated axons. Neuroscience. 1986;19(1):321-341.
80. S.G. Waxman. Demyelination in spinal cord injury. J Neurol Sci. 1989;91(1–2):1-14.
81. R.F. Gledhill, W.I. McDonald. Morphological characteristics of central demyelination and remyelination: a single-fiber study. Ann Neurol. 1977;1(6):552-560.
82. T. Siegal, E. Shezen, T. Siegal. The effect of in-vivo inhibition of phagocytic activity in experimental neoplastic spinal cord compression: Immunohistochemistry, vascular permeability and neurologic function. J Neuro-Oncol. 1994;21:68. (Abst P269)
83. M. Farooque, J. Isaksson, Y. Olsson. Improved recovery after spinal cord injury in neuronal nitric oxide synthase-deficient mice but not in TNF-alpha-deficient mice. J Neurotrauma. 2001;18(1):105-114.
84. M. Farooque, J. Isaksson, Y. Olsson. White matter preservation after spinal cord injury in ICAM-1/P-selectin-deficient mice. Acta Neuropathol (Berl). 2001;102(2):132-140.
85. S. Sorensen, S. Helweg-Larsen, H. Mouridsen, H.H. Hansen. Effect of high-dose dexamethasone in carcinomatous metastatic spinal cord compression treated with radiotherapy: a randomised trial. Eur J Cancer. 1994;30A(1):22-27.
86. Y. Guo, B. Young, J.L. Palmer, Y. Mun, E. Bruera. Prognostic factors for survival in metastatic spinal cord compression: a retrospective study in a rehabilitation setting. Am J Phys Med Rehabil. 2003;82(9):665-668.
87. W.O. McKinley, M.E. Huang, M.A. Tewksbury. Neoplastic vs. traumatic spinal cord injury: an inpatient rehabilitation comparison. Am J Phys Med Rehabil. 2000;79(2):138-144.
88. D. Rades, F. Heidenreich, M. Bremer, J.H. Karstens. Time of developing motor deficits before radiotherapy as a new and relevant prognostic factor in metastatic spinal cord compression: final results of a retrospective analysis. Eur Neurol. 2001;45(4):266-269.
89. I.E. Eriks, E.L. Angenot, G.J. Lankhorst. Epidural metastatic spinal cord compression: functional outcome and survival after inpatient rehabilitation. Spinal Cord. 2004;42(4):235-239.
90. D. Schiff. Spinal cord compression. Neurol Clin. 2003;21(1):67-86. viii
91. T.J. Podd, D.S. Carpenter, C.A. Baughan, D. Percival, P. Dyson. Spinal cord compression: prognosis and implications for treatment fractionation. Clin Oncol (R Coll Radiol). 1992;4(6):341-344.
92. H. Tatsui, T. Onomura, S. Morishita, M. Oketa, T. Inoue. Survival rates of patients with metastatic spinal cancer after scintigraphic detection of abnormal radioactive accumulation. Spine. 1996;21(18):2143-2148.
93. H.C. Bauer, R. Wedin. Survival after surgery for spinal and extremity metastases. Prognostication in 241 patients. Acta Orthop Scand. 1995;66(2):143-146.
94. E. Maranzano, P. Latini. Effectiveness of radiation therapy without surgery in metastatic spinal cord compression: final results from a prospective trial. Int J Radiat Oncol Biol Phys. 1995;32(4):959-967.
95. H.S. Greenberg, J.H. Kim, J.B. Posner. Epidural spinal cord compression from metastatic tumor: results with a new treatment protocol. Ann Neurol. 1980;8(4):361-366.
96. J.P. Constans, E. de Divitiis, R. Donzelli, R. Spaziante, J.F. Meder, C. Haye. Spinal metastases with neurological manifestations. Review of 600 cases. J Neurosurg. 1983;59(1):111-118.
97. N. Sundaresan, J.H. Galicich, J.M. Lane, M.S. Bains, P. McCormack. Treatment of neoplastic epidural cord compression by vertebral body resection and stabilization. J Neurosurg. 1985;63(5):676-684.
98. S. Turner, B. Marosszeky, I. Timms, J. Boyages. Malignant spinal cord compression: a prospective evaluation. Int J Radiat Oncol Biol Phys. 1993;26(1):141-146.
99. S. Helweg-Larsen. Clinical outcome in metastatic spinal cord compression. A prospective study of 153 patients. Acta Neurol Scand. 1996;94(4):269-275.
100. N. Sundaresan, V.P. Sachdev, J.F. Holland, F. Moore, M. Sung, P.A. Paciucci, et al. Surgical treatment of spinal cord compression from epidural metastasis. J Clin Oncol. 1995;13(9):2330-2335.
101. R.A. Huddart, B. Rajan, M. Law, L. Meyer, D.P. Dearnaley. Spinal cord compression in prostate cancer: treatment outcome and prognostic factors. Radiother Oncol. 1997;44(3):229-236.
102. R.W. Gilbert, J.H. Kim, J.B. Posner. Epidural spinal cord compression from metastatic tumor: diagnosis and treatment. Ann Neurol. 1978;3(1):40-51.
103. T. Siegal. Surgical decompression of anterior and posterior malignant epidural tumors compressing the spinal cord: a prospective study. Neurosurgery. 1985;17(3):424-432.
104. D.J. Husband. Malignant spinal cord compression: prospective study of delays in referral and treatment. Bmj. 1998;317(7150):18-21.
105. S. Helweg-Larsen, P.S. Sorensen. Symptoms and signs in metastatic spinal cord compression: a study of progression from first symptom until diagnosis in 153 patients. Eur J Cancer. 1994;30A(3):396-398.
106. D. Rades, M. Blach, M. Bremer, I. Wildfang, J.H. Karstens, F. Heidenreich. Prognostic significance of the time of developing motor deficits before radiation therapy in metastatic spinal cord compression: one-year results of a prospective trial. Int J Radiat Oncol Biol Phys. 2000;48(5):1403-1408.
107. D. Rades, F. Heidenreich, J.H. Karstens. Final results of a prospective study of the prognostic value of the time to develop motor deficits before irradiation in metastatic spinal cord compression. Int J Radiat Oncol Biol Phys. 2002;53(4):975-979.
108. D. Rades, J.H. Karstens, W. Alberti. Role of radiotherapy in the treatment of motor dysfunction due to metastatic spinal cord compression: comparison of three different fractionation schedules. Int J Radiat Oncol Biol Phys. 2002;54(4):1160-1164.
109. S. Helweg-Larsen, B. Rasmusson, P.S. Sorensen. Recovery of gait after radiotherapy in paralytic patients with metastatic epidural spinal cord compression. Neurology. 1990;40(8):1234-1236.
110. C.J. Vecht, H. Haaxma-Reiche, W.L. van Putten, M. de Visser, E.P. Vries, A. Twijnstra. Initial bolus of conventional versus high-dose dexamethasone in metastatic spinal cord compression. Neurology. 1989;39(9):1255-1257.
111. P.R. Clarke, M. Saunders. Steroid-induced remission in spinal canal reticulum cell sarcoma. Report of two cases. J Neurosurg. 1975;42(3):346-348.
112. L.F. Marshall, T.W. Langfitt. Combined therapy for metastatic extradural tumors of the spine. Cancer. 1977;40(5):2067-2070.
113. T. Siegal. Current considerations in the management of neoplastic spinal cord compression. Spine. 1989;14(2):223-228.
114. T. Siegal. Spinal epidural involvement in haematological tumors: clinical featuresand therapeutic options. Leuk Lymphoma. 1991;5:101-110.
115. E.T. Wong, C.S. Portlock, J.P. O’Brien, L.M. DeAngelis. Chemosensitive epidural spinal cord disease in non-Hodgkins lymphoma. Neurology. 1996;46(6):1543-1547.
116. K. Cooper, D. Bajorin, W. Shapiro, G. Krol, G. Sze, G.J. Bosl. Decompression of epidural metastases from germ cell tumors with chemotherapy. J Neurooncol. 1990;8(3):275-280.
117. G.B. Gale, D.M. O’Connor, J.Y. Chu, S. Tantana, T.R. Weber. Successful chemotherapeutic decompression of epidural malignant germ cell tumor. Med Pediatr Oncol. 1986;14(2):97-99.
118. J. Gale, G.M. Mead, P.D. Simmonds. Management of spinal cord and cauda equina compression secondary to epidural metastatic disease in adults with malignant germ cell tumours. Clin Oncol (R Coll Radiol). 2002;14(6):481-490.
119. F.A. Hayes, E.I. Thompson, E. Hvizdala, D. O’Connor, A.A. Green. Chemotherapy as an alternative to laminectomy and radiation in the management of epidural tumor. J Pediatr. 1984;104(2):221-224.
120. C.E. Fadul, W. Lemann, H.T. Thaler, J.B. Posner. Perforation of the gastrointestinal tract in patients receiving steroids for neurologic disease. Neurology. 1988;38(3):348-352.
121. D.E. Weissman. Glucocorticoid treatment for brain metastases and epidural spinal cord compression: a review. J Clin Oncol. 1988;6(3):543-551.
122. H.L. Weiner, A.R. Rezai, P.R. Cooper. Sigmoid diverticular perforation in neurosurgical patients receiving high-dose corticosteroids. Neurosurgery. 1993;33(1):40-43.
123. J.A. MartensonJr, R.G. Evans, M.R. Lie, D.M. Ilstrup, R.P. Dinapoli, M.J. Ebersold, et al. Treatment outcome and complications in patients treated for malignant epidural spinal cord compression (SCC). J Neurooncol. 1985;3(1):77-84.
124. E. Maranzano, P. Latini, S. Beneventi, E. Perruci, B.M. Panizza, C. Aristei, et al. Radiotherapy without steroids in selected metastatic spinal cord compression patients. A phase II trial. Am J Clin Oncol. 1996;19(2):179-183.
125. E.A. Obbens, J.H. Kim, H. Thaler, M.D. Deck, J.B. Posner. Metronidazole as a radiation enhancer in the treatment of metastatic epidural spinal cord compression. J Neurooncol. 1984;2(2):99-104.
126. K.M. Harrison, H.B. Muss, M.R. Ball, M. McWhorter, D. Case. Spinal cord compression in breast cancer. Cancer. 1985;55(12):2839-2844.
127. E. Maranzano, P. Latini, E. Perrucci, S. Beneventi, M. Lupattelli, E. Corgna. Short-course radiotherapy (8 Gy x 2) in metastatic spinal cord compression: an effective and feasible treatment. Int J Radiat Oncol Biol Phys. 1997;38(5):1037-1044.
128. F. Kovner, S. Spigel, I. Rider, I. Otremsky, I. Ron, E. Shohat, et al. Radiation therapy of metastatic spinal cord compression. Multidisciplinary team diagnosis and treatment. J Neurooncol. 1999;42(1):85-92.
129. P.D. Brown, S.L. Stafford, S.E. Schild, J.A. Martenson, D. Schiff. Metastatic spinal cord compression in patients with colorectal cancer. J Neurooncol. 1999;44(2):175-180.
130. W.F. Regine, P.A. Tibbs, A. Young, R. Payne, S. Saris, R.J. Kryscio, et al. Metastatic spinal cord compression: a randomized trial of direct decompressive surgical resection plus radiotherapy vs. radiotherapy alone. Int J Radiat Oncol Biol Phys. 2003;57(Suppl. 2):S125.
131. E. Maranzano, R. Bellavita, P. Floridi, G. Celani, E. Righetti, M. Lupattelli, et al. Radiation-induced myelopathy in long-term surviving metastatic spinal cord compression patients after hypofractionated radiotherapy: a clinical and magnetic resonance imaging analysis. Radiother Oncol. 2001;60(3):281-288.
132. P.J. Hoskin, A. Grover, R. Bhana. Metastatic spinal cord compression: radiotherapy outcome and dose fractionation. Radiother Oncol. 2003;68(2):175-180.
133. D. Rades, J.H. Karstens. A comparison of two different radiation schedules for metastatic spinal cord compression considering a new prognostic factor. Strahlenther Onkol. 2002;178(10):556-561.
134. K.D. Harrington. Anterior cord decompression and spinal stabilization for patients with metastatic lesions of the spine. J Neurosurg. 1984;61(1):107-117.
135. T. Siegal, P. Tiqva. Vertebral body resection for epidural compression by malignant tumors. Results of forty-seven consecutive operative procedures. J Bone Joint Surg Am. 1985;67(3):375-382.
136. P.R. Cooper, T.J. Errico, R. Martin, B. Crawford, T. DiBartolo. A systematic approach to spinal reconstruction after anterior decompression for neoplastic disease of the thoracic and lumbar spine. Neurosurgery. 1993;32(1):1-8.
137. M. Onimus, S. Schraub, D. Bertin, J.F. Bosset, M. Guidet. Surgical treatment of vertebral metastasis. Spine. 1986;11(9):883-891.
138. M. Onimus, P. Papin, S. Gangloff. Results of surgical treatment of spinal thoracic and lumbar metastases. Eur Spine J. 1996;5(6):407-411.
139. K. Tomita, N. Kawahara, T. Kobayashi, A. Yoshida, H. Murakami, T. Akamaru. Surgical strategy for spinal metastases. Spine. 2001;26(3):298-306.
140. H.R. Durr, B. Wegener, A. Krodel, P.E. Muller, V. Jansson, H.J. Refior. Multiple myeloma: surgery of the spine: retrospective analysis of 27 patients. Spine. 2002;27(3):320-324. discussion 325–6
141. A. Schoeggl, M. Reddy, C. Matula. Neurological outcome following laminectomy in spinal metastases. Spinal Cord. 2002;40(7):363-366.
142. H. Hirabayashi, S. Ebara, T. Kinoshita, Y. Yuzawa, I. Nakamura, J. Takahashi, et al. Clinical outcome and survival after palliative surgery for spinal metastases: palliative surgery in spinal metastases. Cancer. 2003;97(2):476-484.
143. N.C. Hatrick, J.D. Lucas, A.R. Timothy, M.A. Smith. The surgical treatment of metastatic disease of the spine. Radiother Oncol. 2000;56(3):335-339.
144. J.J. Wise, J.S. Fischgrund, H.N. Herkowitz, D. Montgomery, L.T. Kurz. Complication, survival rates, and risk factors of surgery for metastatic disease of the spine. Spine. 1999;24(18):1943-1951.
145. G.L. Walsh, Z.L. Gokaslan, I.E. McCutcheon, M.T. Mineo, A.W. Yasko, S.G. Swisher, et al. Anterior approaches to the thoracic spine in patients with cancer: indications and results. Ann Thorac Surg. 1997;64(6):1611-1618.
146. G. Sapkas, J. Kyratzoulis, N. Papaioannou, G. Babis, D. Rologis, S. Tzanis. Spinal cord decompression and stabilization in malignant lesions of the spine. Acta Orthop Scand Suppl. 1997;275:97-100.
147. Z.L. Gokaslan, J.E. York, G.L. Walsh, I.E. McCutcheon, F.F. Lang, J.B. PutnamJr, et al. Transthoracic vertebrectomy for metastatic spinal tumors. J Neurosurg. 1998;89(4):599-609.
148. I.S. Fyfe, A.P. Henry, R.C. Mulholland. Closed vertebral biopsy. J Bone Joint Surg Br. 1983;65(2):140-143.
149. D. Schiff, E.G. Shaw, T.L. Cascino. Outcome after spinal reirradiation for malignant epidural spinal cord compression. Ann Neurol. 1995;37(5):583-589.
150. A.L. Grosu, N. Andratschke, C. Nieder, M. Molls. Retreatment of the spinal cord with palliative radiotherapy. Int J Radiat Oncol Biol Phys. 2002;52(5):1288-1292.
151. S. Milker-Zabel, A. Zabel, C. Thilmann, W. Schlegel, M. Wannenmacher, J. Debus. Clinical results of retreatment of vertebral bone metastases by stereotactic conformal radiotherapy and intensity-modulated radiotherapy. Int J Radiat Oncol Biol Phys. 2003;55(1):162-167.
152. R.F. McLain, J.N. Weinstein. Tumors of the spine. Semin Spine Surgery. 1990;2:157-180.
153. K. Shimizu, J. Shikata, H. Iida, R. Iwasaki, J. Yoshikawa, T. Yamamuro. Posterior decompression and stabilization for multiple metastatic tumors of the spine. Spine. 1992;17(11):1400-1404.
154. W.J. Levy, J.P. LatchawJr, R.W. Hardy, J.P. Hahn. Encouraging surgical results in walking patients with epidural metastases. Neurosurgery. 1982;11(2):229-233.
155. R.C. DunnJr, W.A. Kelly, R.N. Wohns, J.F. Howe. Spinal epidural neoplasia. A 15-year review of the results of surgical therapy. J Neurosurg. 1980;52(1):47-51.
156. R.L. DeWald, K.H. Bridwell, C. Prodromas, M.F. Rodts. Reconstructive spinal surgery as palliation for metastatic malignancies of the spine. Spine. 1985;10(1):21-26.
157. R.G. Perrin, R.J. McBroom. Anterior versus posterior decompression for symptomatic spinal metastasis. Can J Neurol Sci. 1987;14(1):75-80.
158. M. Heller, R.J. McBroom, T. MacNab, R. Perfin. Treatment of metastatic disease of the spine with posterolateral decompression and Luque instrumentation. Neuroorthopedics. 1986;2:70-74.
159. R.M. Sherman, J.P. Waddell. Laminectomy for metastatic epidural spinal cord tumors. Posterior stabilization, radiotherapy, and preoperative assessment. Clin Orthop. 1986;207:55-63.
160. N.E. Slatkin, J.B. Posner. Management of spinal epidural metastases. Clin Neurosurg. 1983;30:698-716.
161. I.B. McPhee, R.P. Williams, C.E. Swanson. Factors influencing wound healing after surgery for metastatic disease of the spine. Spine. 1998;23(6):726-732. discussion 732–3
162. N. Sundaresan, A. Rothman, K. Manhart, K. Kelliher. Surgery for solitary metastases of the spine: rationale and results of treatment. Spine. 2002;27(16):1802-1806.
163. Z. Ghogawala, F.L. Mansfield, L.F. Borges. Spinal radiation before surgical decompression adversely affects outcomes of surgery for symptomatic metastatic spinal cord compression. Spine. 2001;26(7):818-824.
164. T.S. Chow, I.E. McCutcheon. The surgical treatment of metastatic spinal tumors within the intradural extramedullary compartment. J Neurosurg. 1996;85(2):225-230.
165. R.G. Perrin, K.E. Livingston, B. Aarabi. Intradural extramedullary spinal metastasis. A report of 10 cases. J Neurosurg. 1982;56(6):835-837.
166. R.O. Mirimanoff, N.C. Choi. Intradural spinal metastases in patients with posterior fossa brain metastases from various primary cancers. Oncology. 1987;44(4):232-236.
167. D.A. Costigan, M.D. Winkelman. Intramedullary spinal cord metastasis. A clinicopathological study of 13 cases. J Neurosurg. 1985;62(2):227-233.
168. J.L. Grem, J. Burgess, D.L. Trump. Clinical features and natural history of intramedullary spinal cord metastasis. Cancer. 1985;56(9):2305-2314.
169. J.W. Dunne, C.G. Harper, R. Pamphlett. Intramedullary spinal cord metastases: a clinical and pathological study of nine cases. Q J Med. 1986;61(235):1003-1020.
170. O.E. Schijns, E. Kurt, P. Wessels, G.J. Luijckx, E.A. Beuls. Intramedullary spinal cord metastasis as a first manifestation of a renal cell carcinoma: report of a case and review of the literature. Clin Neurol Neurosurg. 2000;102(4):249-254.
171. T.G. Gasser, J. Pospiech, D. Stolke, K. Schwechheimer. Spinal intramedullary metastases. Report of two cases and review of the literature. Neurosurg Rev. 2001;24(2–3):88-92.
172. M. Ogino, R. Ueda, M. Nakatsukasa, I. Murase. Successful removal of solitary intramedullary spinal cord metastasis from colon cancer. Clin Neurol Neurosurg. 2002;104(2):152-156.
173. J.M. Findlay, M. Bernstein, R.G. Vanderlinden, L. Resch. Microsurgical resection of solitary intramedullary spinal cord metastases. Neurosurgery. 1987;21(6):911-915.