Chapter 28 Spinal Cord Tumors
Epidemiology and Etiology
Primary spinal cord tumors comprise 3% to 4% of all primary central nervous system (CNS) tumors in adults.1 Age-adjusted incidence rates are slightly higher for men than women, 0.68 versus 0.61 per 100,000 person-years, respectively.1 Most of these tumors present as extramedullary tumors (70%), whereas intramedullary tumors are less common (30%). Approximately 15% to 25% of spinal tumors involve the cervical spine, including the foramen magnum; 50% to 55% involve the thoracic spinal canal; and 25% to 30% involve the lumbosacral spine.
In the pediatric population, spinal cord tumors are slightly more common, 5% to 6% of all primary CNS tumors.1 Although ependymomas and nerve sheath tumors are the most common histologic types, gliomas make up a larger percentage of tumors in children as compared with adults1 (Table 28-1).
TABLE 28-1 Distribution of Primary Spinal Cord Tumors by Histologic Type (CBTRUS 2010)
Histologic Type | Adult Distribution | Pediatric Distribution |
---|---|---|
Nerve sheath tumor | 40.5% | 15.7% |
Glial origin | ||
Ependymoma | 33.6% | 24.1% |
Pilocytic astrocytoma | 1.4% | 12.6% |
Other glioma | 4.1% | 13.3% |
Meningeal tumor | 6.1% | 10.1% |
Hemangioma | 2.6% | NA |
NA, not available.
Biologic Characteristics and Molecular Biology
The diversity of primary spinal axis tumors partly results from the large spectrum of phenotypically distinct cells in the axis that are capable of neoplastic transformation.2 The genetic and molecular bases of spinal cord tumors are not yet well understood and vary depending on histologic findings.3 Most tumors are histologically benign but can cause significant morbidity by direct compression of important neural structures.
Anatomy, Pathology, and Pathways of Spread
Anatomy
The spinal cord is responsible for transmitting motor and sensory neural signals between the peripheral nervous system and the brain and contains its own independent pathways for coordinating certain reflexes (Fig. 28-1). Functionally, the cord is divided into 31 segments. At each level, a pair of spinal nerves exits: 8 cervical, 12 thoracic, 5 lumbar, and 5 sacral pairs and 1 coccygeal pair. In the cervical region, the spinal nerves exit above the corresponding vertebrae, whereas from the thoracic region and downward, the spinal nerves exit below the equivalent named vertebrae. Although the vertebral column continues to lengthen until adulthood, the canal and the cord are actually much shorter than the column because both stop lengthening at approximately 4 years of age. This growth differential is responsible for the formation of the cauda equina, a collection of the lower lumbar, sacral, and coccygeal nerves that fills the lower spinal column beyond the conus medullaris.
Tumors are classified based on their location (Fig. 28-2). Intradural tumors include both intramedullary and extramedullary tumors. Intramedullary tumors develop from the cells that make up the spinal cord and cauda equina, and extramedullary tumors arise from the supportive tissues that lie outside the spinal cord and cauda equina, such as the meninges, vascular supply, and connective tissue. Extradural tumors arise from the vertebral canal itself or supportive tissues surrounding the dura.
Pathology and Pathways of Spread
The overall and anatomic distributions of histologic types are listed in Tables 28-1 and 28-2. Intradural extramedullary schwannoma (neurilemmoma) and meningioma are the most common intradural tumors, although occasionally they may present as extradural tumors. Other intradural extramedullary tumors include epidermoid and drop metastases of intracranial primary tumors such as medulloblastoma. The most common intramedullary tumors are those derived from glial precursors, most frequently, ependymomas and astrocytomas of low to intermediate grade. Nonneoplastic conditions may mimic spinal cord tumors (Fig. 28-3), including acute transverse myelitis, Angiostrongylus cantonensis infection, infectious meningitis, intradural disc hernia, pseudotumor, sarcoidosis, and tuberculosis.4–8
TABLE 28-2 Anatomic Distribution of Spinal Tumors by Histologic Type
Extradural Tumors |
Intradural Extramedullary Tumors | Intradural Intramedullary Tumors |
---|---|---|
The predominant mode of spread of primary tumors is by direct extension. Spread along the subarachnoid space with spinal or cranial involvement of the meninges may also occur. One should not always assume that the spinal cord tumor found on imaging is the primary lesion. Although CSF seeding is uncommon, supratentorial tumors that show a tendency toward leptomeningeal metastases include 1.2% of glioblastomas and 1.5% of anaplastic gliomas.9 There are no lymphatics in the spinal cord, and hematogenous spread is rare.
Clinical Manifestations, Patient Evaluation, and Staging
The clinical presentation of tumors of the spinal axis is a function of the local anatomy (see Fig. 28-1). Within the spinal canal, there exists a well-defined extradural space containing epidural fat and blood vessels. The extradural space communicates with adjacent extraspinal compartments via the intervertebral foramina.
Clinical Workup
The diagnostic approach to primary spinal cord tumors is shown in Figure 28-4. In addition to focusing on neurologic symptoms, the history should include an evaluation of the patient’s functional status and symptoms, which may suggest metastatic disease or nonmalignant causes of spinal cord tumors.
Sensory impairment often helps to localize the level of the tumor based on loss of dermatome function, although the upper level of impaired long-tract function may be several segments below the actual tumor (Fig. 28-5). A lesion involving the lateral spinothalamic tract causes numbness, paresthesias, and decreased temperature sensation over the contralateral limb or trunk below the lesion. This produces the classic finding of a sensory level, which is best demonstrated with a pin or a large metal or cold object to test temperature sensation and with observation for absence of perspiration. A lesion in the posterior column causes an ataxic or tabetic gait. Standing posture is affected with the eyes closed (Romberg’s sign). Paresthesias can occur below the level of the lesion.
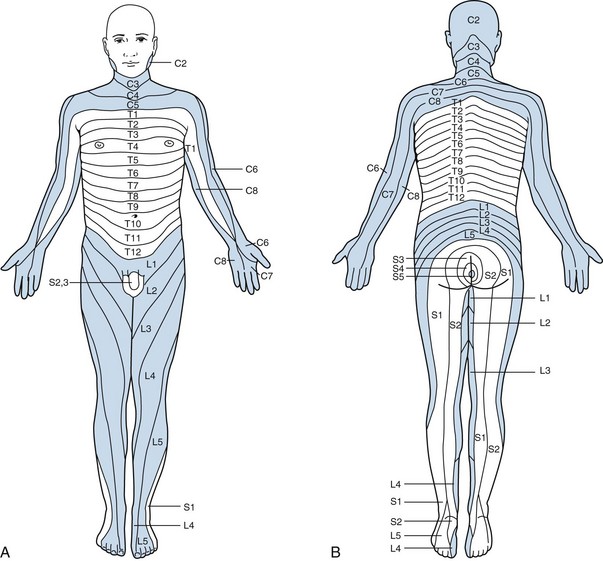
Figure 28-5 Anterior (A) and posterior (B) views of the dermatomes. C, cervical; T, thoracic; L, lumbar; S, sacral.
CREDIT LINE: Adapted from Netter FH, Freidberg SR, Baker RA: Disorders of spinal cord, nerve root, and plexus. In Netter FH, Jones HR Jr, Dingle RV (eds): The Ciba Collection of Medical Illustrations, Vol 1. Nervous System, Part II. West Caldwell, New Jersey, Ciba-Geigy, 1986, pp 181-189; Keegan JJ, Garrett FD: The segmental distribution of cutaneous nerves in the limbs of man. Anat Rec 102:409, 1948.
Primary Therapy
General Principles of Surgery
Surgery has traditionally been the mainstay of therapy for spinal cord tumors.10 As modern diagnostic and therapeutic techniques such as MRI, the operating microscope, microsurgical tools, and intraoperative neurophysiologic assessment have become available, more aggressive surgical intervention has become a more prevalent treatment strategy.11 This section will discuss general principles of surgery for CNS lesions, depending on anatomic location within the spinal cord. The outcome of surgery and its role in multidisciplinary management are described in the sections for each individual type of tumor. Table 28-3 shows an overview of the outcomes of the most common spinal cord tumors by treatment modality.
Surgical Techniques, Postoperative Management, and Complications
Surgery of Intramedullary Tumors
The most common intramedullary tumors are ependymomas and astrocytomas. Modern series advocate maximum safe resection of intramedullary spinal tumors. The ability to resect these tumors completely varies widely, from less than 10% to as much as 86%, depending on the histologic type.12,13–19 Most completely resectable lesions are WHO grade I tumors because higher-grade tumors are defined by their infiltrative potential.16,20–24 The gross total resection (GTR) rates for ependymomas range from 19% to 100%, whereas 0% to 43 % of astrocytomas are resectable.25–28 For infiltrative astrocytomas, the role of surgery is most frequently limited to biopsy for diagnosis. Laminectomy offers limited functional benefit by decompressing the spinal cord in the case of bulky infiltrative tumors. Functional postoperative outcomes may be excellent in selected cases, with the majority of patients (72% to 79%) experiencing either improvement or stabilization of their preoperative presenting symptoms, often after transient postsurgical worsening.12,19,29,30 The incidence of total, permanent postoperative paralysis is reported at 1%.11 Paralysis is often the result of unpredictable, sporadic vascular events.
Intramedullary tumors are almost always approached through a laminectomy exposure with the patient in the prone position.11 After the dura is opened, a longitudinal myelotomy is made over the widened region of the spinal cord, where imaging demonstrates the closest approximation of the tumor to the surface and the incision is deepened several millimeters until the tumor surface is reached. Infiltrative tumors with poorly defined dissection planes cannot be removed completely and, therefore, biopsy for diagnosis is often the safest course of action. When there is an exophytic component to the tumor, maximum safe debulking is typically performed.
Complications of Surgery
In patients who are neurologically intact before surgery, the incidence of significant acute morbidity is generally below 5% for extramedullary tumors and as low as 5% for carefully selected patients with intramedullary tumors.11 Spinal deformities, including scoliosis and kyphosis, as well as infection and CSF leakage, can occur.31,32 The development of postlaminectomy spinal deformities is a serious postoperative complication, occurring in up to 40% of pediatric and adolescent patients, and may be observed as soon as 8 months postoperatively.33
General Principles of Radiation Therapy
Because of the potential for growth and developmental side effects, the use of irradiation in children and infants with spinal cord tumors is controversial. Some authors do not recommend postoperative radiation therapy, and suggest that observation with close imaging follow-up and re-resection at the time of second occurrence is a better approach.12 With this strategy, radiation therapy may be avoided or used only for children with multiply recurrent tumors.
General Principles of Chemotherapy
The efficacy of chemotherapy may be limited by the intact blood–nervous system barrier. Regional drug delivery may be in the form of intracerebrospinal fluid therapy, intra-arterial infusion, and intratumoral therapy. Extramedullary spinal tumors gain their blood supply from meningeal blood vessels that are significantly more permeable than those of the brain, and chemotherapy may be of more use for these tumors. Drugs that have some efficacy in treating primary CNS malignant tumors and that are thought to cross the blood-brain barrier have been used in the treatment of malignant glial tumors of the spinal cord; however, no clear benefit from the use of chemotherapy has been demonstrated thus far.*
Irradiation Techniques and Tolerance
Definitions of Treatment Volumes
Three-dimensional treatment planning based on International Commission on Radiation Units (ICRU) volume definitions is the norm.39,40 The gross target volume (GTV) represents the grossly visible disease burden. Typically, this is the T1-enhancing abnormality on MRI or the nonenhancing tumor seen on T2 or FLAIR images. If there is no residual abnormality after a surgical resection, the tumor resection cavity is defined to be the GTV. Surrounding edema is not considered part of the GTV. The clinical target volume (CTV) is the T2 or FLAIR abnormality (which does include edema) on MRI as well as any areas potentially containing microscopic disease. Suggested definitions and doses to be delivered to the GTV and CTV are listed by histologic diagnosis in Table 28-4. The planning target volume (PTV) adds a dosimetric margin that takes into account uncertainties in daily treatment setup and physiologic variations that are difficult or impossible to control, such as (potential) fluctuations in the mass effect from cord edema that may occur over the course of treatment. Organs at risk typically include the thyroid and salivary glands, esophagus, lungs, heart, stomach, small bowel, liver, kidneys, bladders, ovaries, testicles, and uninvolved portions of the spinal cord itself.
Treatment Techniques
The most common approaches include a single posterior field (PA), opposed lateral fields, a PA field with opposed laterals, and oblique wedge-pair fields.41 They are typically designed to treat the CTV and GTV. For tumors in the cervicothoracic region, a split-beam approach is sometimes used. The central axis is placed just above the shoulders. Opposed lateral fields are used to treat the upper spine, whereas a PA field is used for the area of the spine below. Tumors in the thoracic region are often treated with a three-field approach using a PA field and opposed lateral beams. In the lumbar region, care must be taken to minimize the dose to the kidneys; a four-field approach using AP/PA and opposed lateral beams with the AP/PA beams preferentially weighted may be useful.
Certain neoplasms require treatment of the entire craniospinal axis. Several modifications of this approach are used in clinical practice (Fig. 28-6). Patients may be treated either in the supine or prone position, often in an immobilization cast to ensure daily positional reproducibility.42,43 The intracranial contents, including the upper one or two segments of the cervical cord, are treated through opposed lateral fields. Customized blocks protect the normal head and neck tissues from the primary radiation beam. The spine is treated through one or two posterior fields, depending on the size of the patient. In one method, the collimator for the lateral cranial fields is angled to match the divergence of the upper border of the adjacent spinal field, and the treatment couch is angled so that the inferior border of the cranial field is perpendicular to the superior edge of the spinal field. Alternatively, one may dispense with collimator and couch angles by calculating appropriate gaps. The gap is calculated so that the 50% isodose lines meet at the level of the anterior spinal cord. All junction lines are moved 0.5 to 1 cm every 8 to 10 Gy to avoid overdosing or underdosing segments of the cord. This is accomplished by shortening the inferior margin of the lateral cranial fields, symmetrically lengthening the superior and inferior margins of the posterior spinal field, and shortening the cranial margin of the caudal spinal field; a fixed block is placed at the inferior margin of the caudal spinal field to keep the lower margin of the irradiated volume at the same location.
IMRT is a treatment delivery method that may be used to further optimize the dose distribution. Because multiple critical sensitive organs are located near the spinal cord, improved dose distribution should allow the dose to these structures to be minimized. Because most spinal cord tumors typically recur locally, IMRT should allow the exploration of anatomic/biologic treatment planning and delivery in order to optimize different doses to different cell populations within heterogeneous volumes. The use of image guidance may also allow for reduced margins for sparing of critical structures. The use of IMRT is currently under investigation at multiple institutions.44,45
Stereotactic radiosurgery (SRS) and body radiotherapy (SBRT) deliver a highly conformal high dose of radiotherapy with stereotactic guidance. Doses may be delivered as a single dose (SRS) or as a short fractionated course (SBRT). Potential advantages include patient convenience with a shorter treatment course and the steep dose gradient at the edge of the field, which may allow for greater tissue sparing. SRS for primary spinal cord tumors remains experimental at this time but is an active area of research.46–48
Tolerance of the Spinal Cord and Lumbosacral Nerve Roots
A dose of 45 to 50.4 Gy in 25 to 28 fractions over 5 or weeks is usually considered to be safe. The risk of myelopathy is less than 1%, well below the steep portion of the dose-response curve.46,49,50 It is estimated that with conventionally fractionated irradiation (1.8 to 2 Gy per fraction in five fractions per week), at 5 years the incidence of myelopathy is 5% for doses in the range of 57 to 61 Gy (tolerance dose TD5/5) and 50% for doses of 68 to 73 Gy (TD50/5).49 There is no convincing evidence that the cervical and thoracic cord differ in their radiosensitivity and there appears to be little change in tolerance with variations in the length of cord irradiated.49 Table 28-5 shows a range of isomorbid fractionation schemes, all of which carry a 5% risk of radiation myelopathy.
TABLE 28-5 Fractionation Schemes with a 5% Risk of Radiation-Induced Spinal Cord Myelopathy
Dose per Fraction (Gy) | No. Fractions | Total Dose (Gy) |
---|---|---|
2 | 29 | 58 |
3 | 13 | 39 |
3.3 | 11 | 33 |
4 | 7 | 28 |
5 | 5 | 25 |
10 | 1 | 10 |
Data from references 50, 98, 116, 206–209, 210, 211–214.
The tolerance of the lumbosacral nerve roots appears to be somewhat higher than that of the spinal cord. Most series report a 0% complication rate if patients are treated to doses of 70 Gy (or equivalent) as long as fraction sizes are kept at or below 2 Gy.51–53
The Quantitative Analysis of Normal Tissue Effects in the Clinic (QUANTEC) reviewed data from preclinical, conventional, reirradiation, and SBRT studies in order to refine normal tissue dose/volume tolerance guidelines.54 Based on this, a total dose of 54, 60, or 69 Gy with conventional 2-Gy once-daily fractionation was associated with a myelopathy rate of less than 1%, 6%, and 50%, with a calculated strong dependence on dose/fraction (α/β = 0.87 Gy). With reirradiation of the full cord cross section at conventional fractionation, cord tolerance appears to increase at least 2% at 6 months after an initial course of radiation therapy. For partial cord irradiation as part of spinal SRS, a maximum cord dose of 13 Gy in a single fraction or 20 Gy in three fractions appeared to have a minimal (<1%) risk of injury.55
Primary Therapy By Tumor Type
Astrocytoma
Astrocytomas represent 7% to 11% of all primary spinal tumors. They are the most common intramedullary spinal cord tumors and comprise 35% to 45% of all reported cases of intramedullary tumors. The median age at presentation is approximately 35 years.16,56–58 There does not appear to be a difference in the age of presentation between low- and high-grade tumors.16 In children, 75% to 90% of intramedullary spinal cord tumors are astrocytomas and 85% to 95% of these are low-grade, fibrillary, or juvenile pilocytic astrocytomas (Fig. 28-7). Fewer than 10% of pediatric and 25% of adult spinal cord astrocytomas are malignant.*
Motor weakness is the most common presenting symptom in astrocytomas, occurring in up to 87% of patients. The median time from onset of symptoms to diagnosis ranges from 6 months to 2 years.16,41,57,60 In general, high-grade astrocytomas tend to have a shorter prodrome (median duration, 1.6 to 7 months) than low-grade tumors (median duration, 2 years).16,29,56