Shock, Sepsis, and Multiple Organ Dysfunction Syndrome
Beverly Carlson, Lorraine Fitzsimmons and Christopher Walker
Objectives
• Describe the generalized shock response and systemic inflammatory response.
• Explain the pathophysiology of the five forms of shock and MODS.
• Identify the clinical manifestations of the five forms of shock and MODS.
• Summarize the nursing priorities for managing a patient with each type of shock or MODS.
Be sure to check out the bonus material, including free self-assessment exercises, on the Evolve web site at
http://evolve.elsevier.com/Urden/priorities/.
Shock is an acute, widespread process of impaired tissue perfusion that results in cellular, metabolic, and hemodynamic alterations. Ineffective tissue perfusion occurs when an imbalance develops between cellular oxygen supply and cellular oxygen demand. This imbalance can occur for a variety of reasons and eventually results in cellular dysfunction and death. This chapter presents an overview of the general shock response, or shock syndrome, followed by a discussion of the various shock states.
Shock Syndrome
Shock is a complex pathophysiological process that often results in multiple organ dysfunction syndrome (MODS) and death. All types of shock eventually result in ineffective tissue perfusion and acute circulatory failure. The shock syndrome is a pathway involving a variety of pathological processes that may be categorized as four stages: initial, compensatory, progressive, and refractory. Progression through each stage varies with the patient’s prior condition, duration of initiating event, response to therapy, and correction of underlying cause.
Etiology
Shock can be classified as hypovolemic, cardiogenic, or distributive, depending on the pathophysiological cause and hemodynamic profile. Hypovolemic shock results from a loss of circulating or intravascular volume. Cardiogenic shock results from the impaired ability of the heart to pump. Distributive shock results from maldistribution of circulating blood volume and can be further classified as septic, anaphylactic, or neurogenic. Septic shock is the result of microorganisms entering the body. Anaphylactic shock is the result of a severe antibody-antigen reaction. Neurogenic shock is the result of the loss of sympathetic tone.
Pathophysiology
During the initial stage, cardiac output (CO) is decreased, and tissue perfusion is threatened. Almost immediately, the compensatory stage begins as the body’s homeostatic mechanisms attempt to maintain CO, blood pressure, and tissue perfusion. The compensatory mechanisms are mediated by the sympathetic nervous system (SNS) and consist of neural, hormonal, and chemical responses. The neural response includes an increase in heart rate and contractility, arterial and venous vasoconstriction, and shunting of blood to the vital organs. Hormonal compensation includes activation of the renin response and stimulation of the anterior pituitary and adrenal medulla. Activation of the renin response results in the production of angiotensin II, which causes vasoconstriction and the release of aldosterone and antidiuretic hormone (ADH), leading to sodium and water retention. Stimulation of the anterior pituitary results in the secretion of adrenocorticotropic hormone (ACTH), which stimulates the adrenal cortex to produce glucocorticoids, causing a rise in blood glucose levels. Stimulation of the adrenal medulla causes the release of epinephrine and norepinephrine, which further enhance the compensatory mechanisms.
During the progressive stage, the compensatory mechanisms begin failing to meet tissue metabolic needs, and the shock cycle is perpetuated (Concept Map: Shock). As tissue perfusion becomes ineffective, the cells switch from aerobic to anaerobic metabolism to produce energy. Anaerobic metabolism produces small amounts of energy but large amounts of lactic acid, producing lactic acidemia. Increased vascular permeability from endothelial and epithelial hypoxia and inflammatory mediators results in intravascular hypovolemia, tissue edema, and further decline in tissue perfusion.1,2 A systemic release of inflammatory mediators in response to tissue hypoxia, especially in gut tissue, produces microcirculatory impairment and derangement of cellular metabolism, facilitating progression of the shock cycle.1–3 The patient is experiencing the systemic inflammatory response syndrome (SIRS), and irreversible damage begins to occur. Some cells die as a result of apoptosis, an injury-activated, preprogrammed cellular suicide. Others die as the sodium-potassium pump in the cell membrane fails, causing the cell and its organelles to swell. Cellular energy production comes to a complete halt as the mitochondria swell and rupture. At this point, the problem becomes one of oxygen use instead of oxygen delivery. Even if the cell were to receive more oxygen, it would be unable to use it because of damage to the mitochondria. The cell’s digestive organelles swell and leak destructive enzymes into the cell, accelerating cell death.3
Every system in the body is affected by this process (Box 26-1). Cardiac dysfunction develops as a result of the release of myocardial depressant cytokines.1,2 Ventricular failure eventually occurs, further perpetuating the entire process. Central nervous system (CNS) dysfunction develops as a result of cerebral hypoperfusion, leading to failure of the SNS, cardiac and respiratory depression, and thermoregulatory failure. Endothelial injury from hypoxia and inflammatory cytokines and impaired blood flow result in microvascular thrombosis. Hematological dysfunction occurs as a result of consumption of clotting factors, release of inflammatory cytokines, and dilutional thrombocytopenia. Disseminated intravascular coagulation (DIC) eventually may develop. Pulmonary dysfunction occurs as a result of increased pulmonary capillary membrane permeability, pulmonary microemboli, and pulmonary vasoconstriction. Ventilatory failure and acute lung injury (ALI) develop. Renal dysfunction develops as a result of renal vasoconstriction and renal hypoperfusion, leading to acute tubular necrosis (ATN). Gastrointestinal dysfunction occurs as a result of splanchnic vasoconstriction and hypoperfusion and leads to failure of the gut organs. Disruption of the intestinal epithelium releases gram-negative bacteria into the system, which further perpetuates the entire shock syndrome.4
During the refractory stage, shock becomes unresponsive to therapy and is considered irreversible. As the individual organ systems die, MODS—defined as failure of two or more body systems—occurs. Death is the final outcome. Regardless of the etiological factors, death occurs from ineffective tissue perfusion because of the failure of the circulation to meet the oxygen needs of the cell.3
Assessment and Diagnosis
The patient with a mean arterial blood pressure (MAP) less than 60 mm Hg or with evidence of multisystem organ hypoperfusion is considered to be in a shock state.1,5 Because shock is a dynamic physiological phenomenon, hypotension may occur late in the process.6 Clinical manifestations vary according to the underlying cause of shock, the stage of the shock, and the patient’s response to shock.
Compensatory mechanisms may produce normal hemodynamic values even when tissue perfusion is compromised.4–7 Global indicators of systemic perfusion and oxygenation include serum lactate, arterial base deficit, serum bicarbonate, and central or mixed venous oxygen saturation levels. Inadequate cellular oxygenation with anaerobic metabolism and increased metabolic lactate production increase the serum lactate level.8 The level and duration of this hyperlactatemia are predictive of morbidity and mortality.7–11 The base deficit derived from arterial blood gas (ABG) values also reflects global tissue acidosis and is frequently used to assess the severity of shock.6,7,9 Studies have demonstrated serum bicarbonate to be an equivalent alternative to arterial base deficit in predicting mortality in surgical and trauma patients.12,13 The use of mixed venous oxygen saturation (SvO2) measured by means of a pulmonary artery catheter or central venous oxygen saturation (SCvO2) measured with a central venous catheter allows assessment of the balance of oxygen delivery and oxygen consumption and the ratio of oxygen extraction.14–16 After years of recommended use to guide the care of patients with severe sepsis, this measure of global oxygen balance is being evaluated for use in other critically ill populations.15–21 The sections on different types of shock discuss clinical assessment and diagnosis of the patient in shock.
Medical Management
The major focus of the treatment of shock is the improvement and preservation of tissue perfusion. Adequate tissue perfusion depends on an adequate supply of oxygen being transported to the tissues and the cell’s ability to use it. Oxygen transport is influenced by pulmonary gas exchange, CO, and hemoglobin level. Oxygen use is influenced by the internal metabolic environment. Management of the patient in shock focuses on supporting oxygen delivery.1,22
Adequate pulmonary gas exchange is critical to oxygen transport. Establishing and maintaining an adequate airway are the first steps in ensuring adequate oxygenation. After the airway is patent, emphasis is placed on improving ventilation and oxygenation. Therapies include administration of supplemental oxygen and mechanical ventilatory support.
An adequate CO and hemoglobin level are crucial to oxygen transport. CO depends on heart rate, preload, afterload, and contractility. A variety of fluids and drugs are used to manipulate these parameters. The types of fluids used include crystalloids and colloids. The categories of drugs used include vasoconstrictors, vasodilators, positive inotropes, and antidysrhythmics.
Fluid administration is indicated for decreased preload related to intravascular volume depletion, and it can be accomplished by use of a crystalloid or colloid solution, or both. Crystalloids are balanced electrolyte solutions that may be hypotonic, isotonic, or hypertonic. Examples of crystalloid solutions used in shock situations are normal saline and lactated Ringer’s solution. Colloids are protein- or starch-containing solutions. Examples of colloid solutions are blood and blood components, such as albumin, and pharmaceutical plasma expanders, such as hetastarch, dextran, and mannitol.
The choice of fluid is a subject of debate and depends on the situation.15,23–25 Fluid resuscitation with normal saline or with albumin produces similar outcomes regardless of baseline serum albumin level, and both are considered safe.26,27 Crystalloid solutions are inexpensive and effective. Advantages of colloids include faster restoration of intravascular volume and use of smaller amounts. Colloids are believed to stay in the intravascular space, unlike crystalloids, which readily leak into the extravascular space. Disadvantages include expense, allergic reactions, and difficulties in typing and cross-matching blood. Colloids also can leak out of damaged capillaries and cause a variety of additional problems, particularly in the lungs.
Blood should be considered to augment oxygen transport if the patient’s hemoglobin level is critically low, although controversy exists about what threshold value should be used.4,15 Transfusion of stored red blood cells does not substantially increase oxygen consumption and has been associated with immunosuppression, infection, impairment of microcirculatory flow, coagulopathy, and increased mortality. Restrictive transfusion practice has demonstrated lower mortality.4,16,28 Transfusion-related acute lung injury (TRALI) resulting from immune and nonimmune neutrophil activation has become the leading cause of transfusion-related death and may occur with transfusion of any plasma-containing blood or blood product.28–30
Vasoconstrictor agents are used to increase afterload by increasing the systemic vascular resistance (SVR) and improving the patient’s blood pressure level. Vasodilator agents are used to decrease preload or afterload, or both, by decreasing venous return and SVR. Positive inotropic agents are used to increase contractility. Antidysrhythmic agents are used to influence heart rate.31 Box 26-2 provides examples of each of these agents.
Sodium bicarbonate is not recommended in the treatment of shock-related lactic acidosis.16,32,33 No overall benefit has been found, and the risks associated with its use are significant. They include shifting of the oxyhemoglobin dissociation curve to the left, rebound increase in lactic acid production, development of hyperosmolar state, fluid overload resulting from excessive sodium, and rapid cellular electrolyte shifts.11,32,33
The patient should be started on nutritional support therapy as early as possible. The type of nutritional supplementation initiated varies according to the cause of shock, and it should be tailored to the individual patient’s needs, as indicated by the underlying condition and laboratory data. The enteral route is preferred over the parenteral, although parental nutrition should be considered when enteral feeding is contraindicated.25,34–37 Supplementation of enteral feeding with parenteral nutrition to increase caloric intake should be considered but has not been shown to improve patient outcomes.35–37
Glucose control is recommended for all critically ill patients.38,39 Benefits of glucose control in the critically ill include lower incidences of infection, renal failure, sepsis, polyneuropathy, need for blood transfusion, prolonged mechanical ventilation, and death.38–42
Nursing Management
The nursing management of a patient in shock is a complex and challenging responsibility. It requires an in-depth understanding of the pathophysiology of the disease and the anticipated effects of each intervention, as well as a solid understanding of the nursing process. Later sections discuss specific interventions for the patient in shock.
The psychosocial needs of the patient and family dealing with shock are extremely important. These needs are based on situational, familial, and patient-centered variables. Nursing priorities in managing the patient in shock are directed toward (1) providing information on patient status, (2) explaining procedures and routines, (3) supporting the family, (4) encouraging the expression of feelings, (5) facilitating problem solving and shared decision making, (6) individualizing visitation schedules, (7) involving the family in the patient’s care, and (8) establishing contacts with necessary resources.43 Patients and families should be given the option of family presence during invasive procedures and resuscitation.43–45 Collaborative management of the patient with shock is outlined in the Collaborative Management Box on Shock.
Hypovolemic Shock
Hypovolemic shock occurs from inadequate fluid volume in the intravascular space. The lack of adequate circulating volume leads to decreased tissue perfusion and initiation of the general shock response. Hypovolemic shock is the most commonly occurring form of shock.
Etiology
Hypovolemic shock can result from absolute or relative hypovolemia. Absolute hypovolemia occurs when there is a loss of fluid from the intravascular space. This can result from an external loss of fluid from the body or from internal shifting of fluid from the intravascular space to the extravascular space. Fluid shifts can result from a loss of intravascular integrity, increased capillary membrane permeability, or decreased colloidal osmotic pressure. Relative hypovolemia occurs when vasodilation produces an increase in vascular capacitance relative to circulating volume (Box 26-3).
Pathophysiology
Hypovolemia results in a loss of circulating fluid volume. A decrease in circulating volume leads to a decrease in venous return, which results in a decrease in end-diastolic volume or preload. Preload is a major determinant of stroke volume (SV) and CO. A decrease in preload results in a decrease in SV and CO. The decrease in CO leads to inadequate cellular oxygen supply and ineffective tissue perfusion (Figure 26-1).
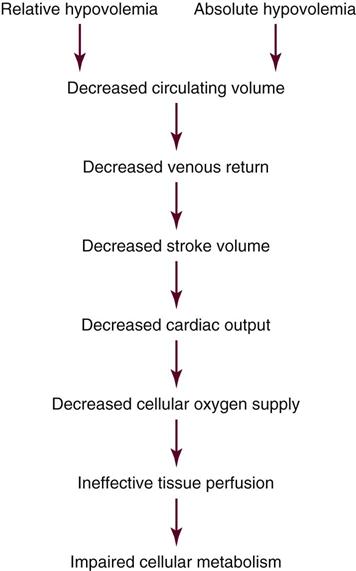
Assessment and Diagnosis
The clinical manifestations of hypovolemic shock depend on the severity of fluid loss and the patient’s ability to compensate for it. Clinical classes have been developed by the American College of Surgeons to describe the levels of severity of hypovolemic shock. Class I indicates a fluid volume loss up to 15% or an actual volume loss up to 750 mL. Compensatory mechanisms maintain CO, and the patient appears free of symptoms other than slight anxiety.4,46
Class II hypovolemia occurs with a fluid volume loss of 15% to 30% or an actual volume loss of 750 to 1500 mL. Falling CO activates more intense compensatory responses. The heart rate increases to more than 100 beats/minute in response to increased SNS stimulation unless blocked by preexisting beta-blocker therapy. The pulse pressure narrows as the diastolic blood pressure increases because of vasoconstriction. The respiratory rate increases to 20 to 30 breaths/minute, and respiratory depth increases in an attempt to improve oxygenation. ABG specimens drawn during this phase reveal respiratory alkalosis and hypoxemia, as evidenced by a low partial pressure of carbon dioxide (PaCO2) and a low partial pressure of oxygen (PaO2), respectively. Urine output starts to decline to 20 to 30 mL/hour as renal perfusion decreases. The urine sodium level decreases, whereas urinary osmolality and specific gravity increase as the kidneys start to conserve sodium and water. The patient’s skin becomes pale and cool with delayed capillary refill because of peripheral vasoconstriction. Jugular veins appear flat as a result of decreased venous return.4,46
Hypovolemic shock that is class III occurs with a fluid volume loss of 30% to 40% or an actual volume loss of 1500 to 2000 mL. This level of severity produces the progressive stage of shock as compensatory mechanisms become overwhelmed and ineffective tissue perfusion develops. Blood pressure decreases. The heart rate increases to more than 120 beats/minute, and dysrhythmias develop as myocardial ischemia ensues. Respiratory distress occurs as the pulmonary system deteriorates. ABG values during this phase reveal respiratory and metabolic acidosis and hypoxemia, as evidenced by a high PaCO2, low bicarbonate (HCO3–), and low PaO2, respectively. Decreased renal perfusion results in the development of oliguria. Blood urea nitrogen (BUN) and serum creatinine levels start to rise as the kidneys begin to fail. The patient’s skin becomes ashen, cold, and clammy, with marked delayed capillary refill. The patient appears confused as cerebral perfusion decreases and the level of consciousness deteriorates.4,46
Class IV hypovolemic shock is usually refractory in nature. It occurs with a fluid volume loss of greater than 40% or an actual volume loss of more than 2000 mL. The compensatory mechanisms of the body completely deteriorate, and organ failure occurs.5 Severe tachycardia and hypotension ensue. Peripheral pulses are absent, and because of marked peripheral vasoconstriction, capillary refill does not occur. The skin appears cyanotic, mottled, and extremely diaphoretic. Urine output ceases. The patient becomes lethargic and unresponsive, and various clinical manifestations associated with failure of the different body systems develop.4,46
Assessment of the hemodynamic parameters of a patient in hypovolemic shock varies by stage but commonly reveals a decreased CO and cardiac index (CI). Loss of circulating volume leads to a decrease in venous return to the heart, which results in a decrease in the preload of the right and left ventricles. This is evidenced by a decline in the central venous pressure (CVP) or right atrial pressure (RAP) and pulmonary artery occlusion pressure (PAOP). Vasoconstriction of the arterial system results in an increase in the afterload of the heart, as evidenced by an increase in the SVR. This vasoconstriction may produce a falsely elevated systolic blood pressure when measured by arterial catheter. MAP is more accurate in this low-flow state.5
Medical Management
The major goals of therapy for the patient in hypovolemic shock are to correct the cause of the hypovolemia, restore tissue perfusion, and prevent complications. This approach includes identifying and stopping the source of fluid loss and administering fluid to replace circulating volume. Fluid administration can be accomplished with use of a crystalloid solution, a colloid solution, blood products, or a combination of fluids. The type of solution used depends on the type of fluid lost, the degree of hypovolemia, the severity of hypoperfusion, and the cause of hypovolemia.
Aggressive fluid resuscitation in trauma and surgical patients is the subject of great debate. The benefit of limited or hypotensive (systolic blood pressure >80 mm Hg) volume resuscitation in patients with uncontrolled hemorrhage is postulated to lessen bleeding and improve survival.22,47–49 The type and amount of solutions used for fluid resuscitation and the rate of administration influence immune function, inflammatory mediator release, coagulation, and the incidence of cardiac, pulmonary, and gastrointestinal complications.23,24,50–52 Consensus on the optimal resuscitative strategy for hypovolemic shock is lacking.23,24,48
Nursing Management
Prevention of hypovolemic shock is one of the primary responsibilities of the nurse in the critical care area. Preventive measures include the identification of patients at risk and frequent assessment of the patient’s fluid balance. Accurate monitoring of intake and output and daily weights are essential components of preventive nursing care. Early identification and treatment result in decreased mortality.
Management of the patient in hypovolemic shock requires continuous evaluation of intravascular volume, tissue perfusion, and response to therapy. The patient in hypovolemic shock may have any number of nursing diagnoses, depending on the progression of the process (Nursing Diagnosis Priorities Box on Hypovolemic Shock). Nursing priorities are directed toward (1) minimizing fluid loss, (2) administering volume replacement, (3) providing comfort and emotional support, and (4) maintaining surveillance for complications.
Measures to minimize fluid loss include limiting blood sampling, observing lines for accidental disconnection, and applying direct pressure to bleeding sites. Measures to facilitate the administration of volume replacement include insertion of large-bore peripheral intravenous catheters, rapid administration of prescribed fluids, and positioning the patient with the legs elevated, trunk flat, and head and shoulders above the chest. Monitoring the patient for clinical manifestations of fluid overload or complications related to fluid and blood product administration is essential for preventing further problems.
Cardiogenic Shock
Cardiogenic shock is the result of failure of the heart to effectively pump blood forward. It can occur with dysfunction of the right or the left ventricle, or both. The lack of adequate pumping function leads to decreased tissue perfusion and circulatory failure. It occurs in approximately 5% to 8% of the patients with an ST-segment myocardial infarction (MI), and it is the leading cause of death of patients hospitalized with MI.53,54 The mortality rate for cardiogenic shock has decreased with the advent of early revascularization therapy and is currently about 47% to 60%.53–56
Etiology
Cardiogenic shock can result from primary ventricular ischemia, structural problems, and dysrhythmias.53,54 The most common cause is acute MI resulting in the loss of 40% or more of the functional myocardium. It can occur with ST-elevation or non-ST-elevation MI.54,57 The damage to the myocardium may occur after one massive MI (usually of the anterior wall), or it may be cumulative as a result of several smaller MIs or a small MI in a patient with preexisting ventricular dysfunction.53,57 End-stage cardiomyopathy may also cause cardiogenic shock as may structural problems of the cardiopulmonary system and dysrhythmias if they disrupt the forward motion of the blood through the heart (Box 26-4).53,54,57
Pathophysiology
Cardiogenic shock results from the impaired ability of the ventricle to pump blood forward, which leads to a decrease in SV and an increase in the blood left in the ventricle at the end of systole. The decrease in SV results in a decrease in CO, which leads to decreased cellular oxygen supply and ineffective tissue perfusion. Typically, myocardial performance spirals downward as compensatory vasoconstriction increases myocardial afterload and low blood pressure worsens myocardial ischemia. Evidence of SIRS has been observed in a substantial number of patients with cardiogenic shock.55,57–59 Activation of inflammatory cytokines induce systemic vasodilation, defective cellular oxygen use, and occasionally, normalization of the CO. Whether this process contributes to the genesis or the outcome of cardiogenic shock is uncertain, but it is thought to be activated by acute MI and to facilitate development of sepsis.55,57,58 As left ventricular contractility declines and ventricular compliance decreases, an increase in end-systolic volume results in blood backing up into the pulmonary system and the subsequent development of pulmonary edema. Pulmonary edema causes impaired gas exchange and decreased oxygenation of the arterial blood, which further impair tissue perfusion (Figure 26-2). Death due to cardiogenic shock may result from multiple organ failure or cardiopulmonary collapse.55,58
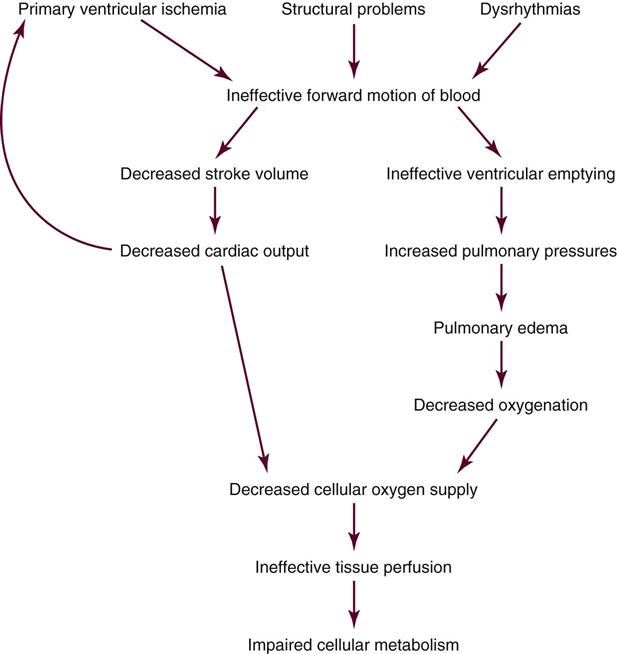
Assessment and Diagnosis
A variety of clinical manifestations occur in the patient in cardiogenic shock, depending on etiological factors in pump failure, the patient’s underlying medical status, and the severity of the shock state. Some clinical manifestations are caused by failure of the heart as a pump, whereas many are related to the overall shock response (Box 26-5).
Initially, clinical manifestations reflect the decline in CO. These signs and symptoms include systolic blood pressure less than 90 mm Hg or an acute drop in systolic or mean blood pressure of 30 mm Hg or more; decreased sensorium; cool, pale, moist skin; and urine output of less than 30 mL/hour.57,60 The patient also may complain of chest pain. Tachycardia develops to compensate for the decrease in CO. A weak, thready pulse develops, and diminished S1 and S2 heart sounds may occur as a result of the decreased contractility. The respiratory rate increases to improve oxygenation. ABG values at this point indicate respiratory alkalosis, as evidenced by a decrease in PaCO2. Urinalysis findings demonstrate a decrease in urine sodium level and an increase in urine osmolality and specific gravity as the kidneys start to conserve sodium and water. The patient also may experience a variety of dysrhythmias, depending on the underlying problem.54
As the left ventricle fails, auscultation of the lungs may disclose crackles and rhonchi, indicating the development of pulmonary edema. Hypoxemia occurs, as evidenced by a fall in PaO2 and SaO2 as measured by ABG values. Heart sounds may reveal an S3 and S4. Jugular venous distention is evident with right-sided failure.
Assessment of the hemodynamic parameters of a patient in cardiogenic shock reveals a decreased CO with a CI less than 2.2 L/minute/m2 in the presence of an elevated PAOP of more than 15 to 18 mm Hg.53,54,57,59 A proportional pulse pressure (systolic BP/pulse pressure) less than 25% is indicative of left ventricular failure and a CI less than 2.2 and may be useful when direct measurement of CI is unavailable.61 Increased filling pressures are necessary to rule out hypovolemia as the cause of circulatory failure. The increase in PAOP reflects an increase in the left ventricular end-diastolic pressure (LVEDP) and left ventricular end-diastolic volume (LVEDV) resulting from decreased SV. With right ventricular failure, the RAP also increases. Compensatory vasoconstriction results in an increase in the afterload of the heart, as evidenced by an increase in the SVR. Echocardiography confirms the diagnosis of cardiogenic shock and rules out other causes of circulatory failure.53,54,57
As compensatory mechanisms fail and ineffective tissue perfusion develops, other clinical manifestations appear. Myocardial ischemia progresses, as evidenced by continued increases in heart rate, dysrhythmias, and chest pain. Pulmonary function deteriorates, which leads to respiratory distress. ABG values during this phase reveal respiratory and metabolic acidosis and hypoxemia, as indicated by a high PaCO2, low HCO3–, and low PaO2, respectively. Renal failure occurs, as exhibited by the development of anuria and increases in BUN and serum creatinine levels. Cerebral hypoperfusion manifests as a decreasing level of consciousness.
Medical Management
Treatment of the patient in cardiogenic shock requires an aggressive approach. The major goals of therapy are to treat the underlying cause, enhance the effectiveness of the pump, and improve tissue perfusion. This approach includes identifying the etiological factors of pump failure and administering pharmacological agents to enhance CO. Inotropic agents are used to increase contractility and maintain adequate blood pressure and tissue perfusion. A vasopressor may be necessary to maintain blood pressure when hypotension is severe.57,60 Diuretics are used for preload reduction. After blood pressure has been stabilized, vasodilating agents are used for preload and afterload reduction. Antidysrhythmic agents should be used to suppress or control dysrhythmias that can affect CO.53 Intubation and mechanical ventilation may be necessary to support oxygenation.
Intraaortic balloon pump (IABP) support should be instituted if drug therapy does not quickly reverse the shock state.54–57 The IABP is a temporary measure to decrease myocardial workload by improving myocardial supply and decreasing myocardial demand. It achieves this goal by improving coronary artery perfusion and reducing left ventricular afterload. Chapter 13 provides more information about IAPB therapy.
After the cause of pump failure has been identified, measures should be taken to correct the problem if possible. If the problem is related to an acute MI, early revascularization by coronary angioplasty or coronary artery bypass surgery provides significant survival benefit.53–57,62 Thrombolytic agents may be used in select patients. When conventional therapies fail, a ventricular assist device (VAD) or extracorporeal life support with a membrane oxygenator may be used to support the patient in acute cardiogenic shock.54,57,63,64 These mechanical circulatory assist devices provide an external means to sustain effective organ perfusion, allowing time for the patient’s ventricle to heal or for cardiac transplantation to take place.
Nursing Management
Prevention of cardiogenic shock is one of the primary responsibilities of the nurse in the critical care area. Preventive measures include the identification of patients at risk, facilitation of early reperfusion therapy for acute MI, and frequent assessment and management of the patient’s cardiopulmonary status.
The patient in cardiogenic shock may have any number of nursing diagnoses, depending on the progression of the process (Nursing Diagnosis Priorities Box on Cardiogenic Shock). Nursing priorities are directed toward (1) limiting myocardial oxygen demand, (2) enhancing myocardial oxygen supply, (3) providing comfort and emotional support, and (4) maintaining surveillance for complications. Measures to limit myocardial oxygen demand include administering analgesics, sedatives, and agents to control afterload and dysrhythmias; positioning the patient for comfort; limiting activities; providing a calm and quiet environment and offering support to reduce anxiety; and teaching the patient about the condition. Measures to enhance myocardial oxygen supply include administering supplemental oxygen, monitoring the patient’s respiratory status, and administering prescribed medications.
Effective nursing management of cardiogenic shock requires precise monitoring and management of heart rate, preload, afterload, and contractility. This is accomplished through accurate measurement of hemodynamic variables and controlled administration of fluids and inotropic and vasoactive agents. Close assessment and management of respiratory function is also essential to maintain adequate oxygenation. Dysrhythmias are common and require immediate recognition and treatment.
Patients who require IABP therapy need to be observed frequently for complications. Complications include embolus formation, infection, rupture of the aorta, thrombocytopenia, improper balloon placement, bleeding, improper timing of the balloon, balloon rupture, and circulatory compromise of the cannulated extremity.
Anaphylactic Shock
Anaphylactic shock, a type of distributive shock, is the result of an immediate hypersensitivity reaction. It is a life-threatening event that requires prompt intervention. The severe antibody-antigen response leads to decreased tissue perfusion and initiation of the general shock response.65,66
Etiology
Anaphylactic shock is caused by an antibody-antigen response. Almost any substance can cause a hypersensitivity reaction. These substances, known as antigens, can be introduced by injection or ingestion or through the skin or respiratory tract. A number of antigens have been identified that can cause a reaction in a hypersensitive person. This list includes foods, food additives, diagnostic agents, biological agents, environmental agents, drugs, and venoms (Box 26-6).66–68 In the hospital environment, latex is an extremely problematic antigen for patients and health care providers (Patient Safety Priorities Box on Latex Allergy).
Anaphylactic reactions can be IgE-mediated or non-IgE-mediated responses. IgE is an antibody that is formed as part of the immune response. The first time an antigen enters the body, an antibody IgE, specific for the antigen, is formed. The antigen-specific IgE antibody is then stored by attachment to mast cells and basophils. This initial contact with the antigen is known as a primary immune response. The next time the antigen enters the body, the preformed IgE antibody reacts with it, and a secondary immune response occurs. This reaction triggers the release of biochemical mediators from the mast cells and basophils and initiates the cascade of events that precipitates anaphylactic shock.66,69,70
Some anaphylactic reactions are non-IgE-mediated responses in that they occur in the absence of activation of IgE antibodies. These responses occur as a result of direct activation of the mast cells to release biochemical mediators. Direct activation of mast cells can be triggered by humoral mediators, such as the complement system and the coagulation-fibrinolytic system. Biochemical mediators can be released as a direct or indirect response to many drugs. This type of reaction, formerly known as anaphylactoid reaction, is produced in persons not previously sensitized, and it can occur with the first exposure to an antigen.66,68,70
Pathophysiology
The antibody-antigen response (immunological stimulation) or the direct triggering (nonimmunological activation) of the mast cells results in the release of biochemical mediators. These mediators include histamine, eosinophil chemotactic factor of anaphylaxis (ECF-A), neutrophil chemotactic factor of anaphylaxis (NCF), platelet-activating factor (PAF), proteinases, heparin, serotonin, leukotrienes (also known as slow-reacting substance of anaphylaxis), and prostaglandins. The activation of the biochemical mediators causes vasodilation; increased capillary permeability; laryngeal edema; bronchoconstriction; excessive mucus secretion; coronary vasoconstriction; inflammation; cutaneous reactions; and constriction of the smooth muscle in the intestinal wall, bladder, and uterus. Coronary vasoconstriction causes severe myocardial depression. Cutaneous reactions cause stimulation of nerve endings, followed by itching and pain.65,66,69
ECF-A promotes chemotaxis of eosinophils, facilitating the movement of eosinophils into the area. During allergic reactions, eosinophils phagocytose the antibody-antigen complex and other inflammatory debris and release enzymes that inhibit vasoactive mediators, such as histamine and leukotrienes. Secondary mediators such as bradykinin and plasmin are produced that enhance or inhibit the already released biochemical mediators. Peripheral vasodilation results in relative hypovolemia and decreased venous return. Increased capillary membrane permeability results in the loss of intravascular volume, worsening the hypovolemic state. Decreased venous return results in decreased end-diastolic volume and SV. The decline in SV leads to decreased CO and ineffective tissue perfusion. Death may result from airway obstruction or cardiovascular collapse, or both (Figure 26-3).65,66,69,70
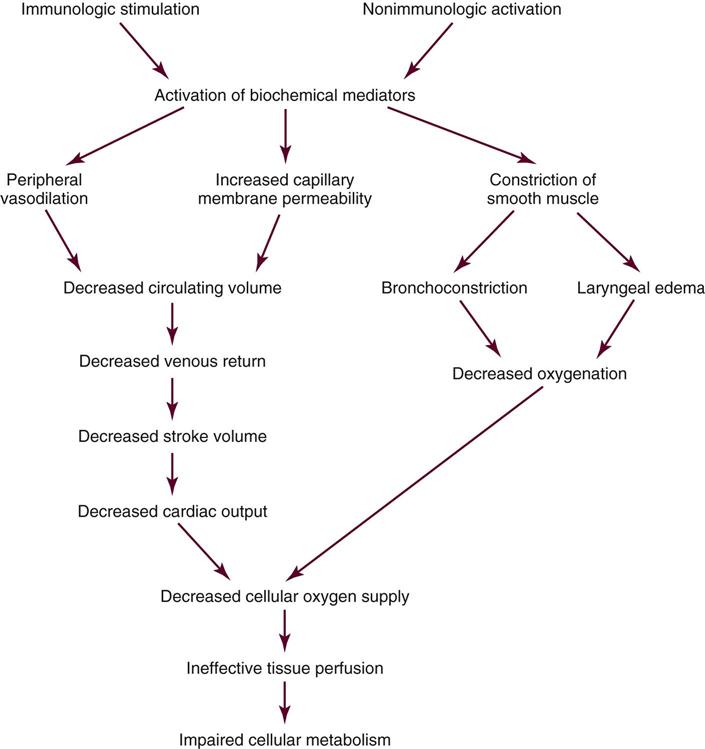
Assessment and Diagnosis
Anaphylactic shock is a severe systemic reaction that can affect multiple organ systems. A variety of clinical manifestations occur in the patient in anaphylactic shock, depending on the extent of multisystem involvement. The symptoms usually start to appear within minutes of exposure to the antigen, but they may not occur for up to 1 hour (Box 26-7).65 Symptoms may also reappear after a 1- to 72-hour window of resolution. These late-phase reactions may be similar to the initial anaphylactic response, milder, or more severe.65,71
The cutaneous effects may appear first and include pruritus, generalized erythema, urticaria, and angioedema. Commonly seen on the face and in the oral cavity and lower pharynx, angioedema develops as a result of fluid leaking into the interstitial space. The patient may appear restless, uneasy, apprehensive, and anxious and may complain of being warm. Respiratory effects include the development of laryngeal edema, bronchoconstriction, and mucous plugs. Clinical manifestations of laryngeal edema include inspiratory stridor, hoarseness, a sensation of fullness or a lump in the throat, and dysphagia. Bronchoconstriction causes dyspnea, wheezing, and chest tightness.65,66,69 Gastrointestinal and genitourinary manifestations, which may develop as a result of smooth muscle contraction, include vomiting, diarrhea, cramping, and abdominal pain.
As the anaphylactic reaction progresses, hypotension and reflex tachycardia develop. This occurs in response to massive vasodilation and loss of circulating volume. Jugular veins appear flat as right ventricular end-diastolic volume is decreased. The eventual outcome is circulatory failure and ineffective tissue perfusion.65,66,69 The patient’s level of consciousness may deteriorate to unresponsiveness.
Assessment of the hemodynamic parameters of a patient in anaphylactic shock reveals a decreased CO and CI. Venous vasodilation and massive volume loss lead to a decrease in preload, which results in a decline in the RAP and PAOP. Vasodilation of the arterial system results in a decrease in the afterload of the heart, as evidenced by a decrease in the SVR. Box 26-8 outlines the clinical criteria for diagnosing anaphylaxis.
Medical Management
Treatment of anaphylactic shock requires an immediate and direct approach. The goals of therapy are to remove the offending antigen, reverse the effects of the biochemical mediators, and promote adequate tissue perfusion. When the hypersensitivity reaction occurs as a result of administration of medications, dye, blood, or blood products, the infusion should be immediately discontinued. Often, it is not possible to remove the antigen because it is unknown or has already entered the patient’s system.
Reversal of the effects of the biochemical mediators involves the preservation and support of the patient’s airway, ventilation, and circulation. This is accomplished through oxygen therapy, intubation, mechanical ventilation, and administration of drugs and fluids.
Epinephrine is the first-line treatment of choice for anaphylaxis. It promotes bronchodilation and vasoconstriction and inhibits further release of biochemical mediators. In mild cases of anaphylaxis, 0.3 to 0.5 mg (0.3 to 0.5 mL) of a 1 : 1000 dilution of epinephrine is administered by intramuscular injection into the anterolateral thigh and repeated every 5 to 15 minutes until anaphylaxis is resolved.65,66,70–72 Subcutaneous injection is no longer recommended.71