Scope of Microbiology
After reading this chapter, the student will be able to:
• Describe the achievements of scientists in the early years of microbiology
• Discuss the different types of microscopes and their use
• Explain the theory of spontaneous generation
• Discuss the germ theory of disease and its significance
• Name and describe Koch’s postulates
• Discuss the origin and evolution of microorganisms
• Describe the differences between prokaryotes and eukaryotes
• Explain the role of taxonomy
• Describe the different ways of microbial transmission
• Name and briefly describe the different uses of microorganisms in everyday life
Origins of Microbiology and Microscopy
Microbiology is the study of microorganisms, using a variety of techniques for purposes of visualization, identification, and study of their function. The science of microbiology originated with the invention and development of the microscope. Microscopy allowed humans to magnify objects and microorganisms not detectable by the naked eye. Technological advances then have led to the improvement of microscopes, which became an essential investigative tool for biology in general and for the study of cells, tissues, and microorganisms (Figure 1.1) in particular.
Microscopy and Its Founding Fathers
Table 1.1 lists some significant events in the history of microbiology.
TABLE 1.1
Significant Events in Microbiology
Name | Year | Event |
Zaccharias Janssen | 1590 | Invention of the first compound microscope |
Robert Hooke | 1660 | Explores living and nonliving matter with a compound microscope |
Francesco Redi | 1668 | Experiments to disprove spontaneous generation |
Antony van Leeuwenhoek | 1676 | Observes bacteria and protozoan “animalcules” with simple microscope |
Francesco Redi | 1688 | Published experiments on spontaneous generation of maggots |
Lazzaro Spallanzani | 1776 | Conducts further experiments to disprove spontaneous generation |
Edward Jenner | 1796 | Introduction of smallpox vaccination |
Ignaz Semmelweis | 1847–1850 | First use of antiseptics to reduce hand-borne disease |
Louis Pasteur | 1857 | Proves that fermentation is caused by microorganisms; introduces pasteurization |
Louis Pasteur | 1861 | Completes experiments that show without doubt that spontaneous generation does not occur |
Joseph Lister | 1867 | Antiseptic surgery—begins the trend toward modern aseptic techniques |
Robert Koch | 1876–1877 | Studies anthrax in cattle and implicates Bacillus anthracis as causative agent |
Louis Pasteur | 1881 | Develops anthrax vaccine for animals |
Robert Koch | 1882 | Identifies causative agent of tuberculosis |
Robert Koch | 1884 | Describes his postulates |
Types of Microscopes
All light microscopes use visible light to illuminate, and optical lenses to observe, enlarged images of specimens. They are classified as either simple or compound. A simple light microscope, such as van Leeuwenhoek’s, has a single magnifying lens and a visible light source, and can magnify objects approximately ×266. A compound light microscope also uses visible light, usually provided by an electric source, but uses multiple lenses for magnification. The lens or lenses close to the eye are called ocular lenses and are located in the headpiece of the microscope. The lenses closer to the specimen are located in the body of the microscope and are referred to as objective lenses. Each lens has its own magnifying power, and the final magnification of a compound microscope is the product of the enlarging power of the ocular lens multiplied by the power of the objective lens. Most often the ocular lenses, either single (monocular) or in pairs (binocular), magnify by a power of 10 (×10). The objective lenses are mounted on a revolving nosepiece and usually magnify ×4 or ×5, ×10, ×40, and ×100. In general, compound microscopes can magnify an object up to 1000 times (i.e., an ocular lens with a magnification of ×10 times the objective lens with a power of ×100 = ×1000). The specimens for compound light microscopy can either be visualized as whole (i.e., bacteria and other microorganisms) or are specially prepared for viewing with a given type of microscope. After specific dehydration procedures larger specimens are cut into 1.0- to 10-µm sections. Both smear preparations, for single cells, and sections are usually stained for better visual images (see Chapter 4, Microbiological Laboratory Techniques). Photographs taken through a microscope are referred to as photomicrographs or micrographs.
Bright-field Microscopes
The bright-field microscope, a type of compound microscope, can be used to examine small specimens and some of their details. The microscope exhibits a background brighter than the observed specimen and is dependent on altering the light path (refraction) only. For this reason most specimens require staining for optimal observation. Bright-field microscopes are most commonly used to observe sectioned and stained tissues, organs, and microorganisms (Figure 1.2).
Dark-field Microscopes
Unfixed, unstained specimens such as living organisms (i.e., bacteria) can be observed with a dark-field microscope. Because of its design, the background of the microscope slide is dark, whereas the organism itself is bright (Figure 1.3). Some bacteria that are difficult to stain, including spirochetes (see Chapter 6, Bacteria and Archaea), can best be observed by dark-field microscopy. Bacterial capsules are also good candidates to be observed with dark-field microscopes.
Fluorescence Microscopes
If a specimen can emit light (fluoresce) of one color when illuminated by ultraviolet radiation then fluorescence microscopy may be the method of choice. Fluorescence microscopes are used to visualize specimens that contain natural fluorescent substances such as chlorophyll or those stained with a fluorescent dye such as fluorescein, auramine, or rhodamine B (Figure 1.5). Fluorescence microscopy is an important and widely used tool in the diagnosis of infectious disease and in studies in microbial ecology. Fluorescence techniques are applied to identify specific antibodies, which are proteins produced in response to antigens (see Chapter 20, The Immune System). By attaching a fluorescent dye to these protein molecules, labeled antibodies are created that can be visualized, monitored, and studied.
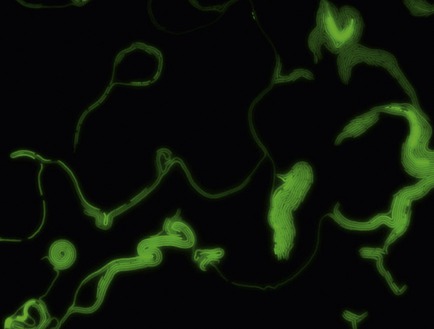
The fluorescence microscope uses an ultraviolet lamp to illuminate specimens stained with fluorescent stain. This is a fluorescence microscopy photo of a modified strain of Pseudomonas. (Approximate magnification: ×1000.) Courtesy Dr. Larry Halverson, Iowa State University.
Transmission Electron Microscopes
In a transmission electron microscope the electron beam travels through an ultrathin sectioned specimen (approximately 100 nm in thickness) and provides a two-dimensional image of the cell or other object. The resolving power of a TEM is approximately 0.002 µm, which is 100 times greater than can be achieved with a light microscope. The usual magnification of a TEM ranges from 500,000 to 1,000,000. Pictures taken of images created with an electron microscope are called electron micrographs (Figure 1.6).
Scanning Electron Microscopes
A scanning electron microscope also provides images of high resolution, but in contrast to the TEM, the SEM does not require ultrathin sections. It scans the surface of an object, producing a three-dimensional image (Figure 1.7). Moreover, the SEM has a large depth of field that allows the surface areas of large samples to be in focus at the same time. The usual magnification that can be achieved with an SEM ranges from ×10 to ×100,000.