Chapter 4 Retina
The innermost coat of the eye is a neural layer, the retina, located between the choroid and the vitreous. It includes the macula, the area at the posterior pole used for sharpest acuity and color vision. The retina extends from the circular edge of the optic disc, where the nerve fibers exit the eye, to the ora serrata. It is continuous with the epithelial layers of the ciliary body, with which it shares embryologic origin. The retina is derived from neural ectoderm and consists of an outer pigmented layer, derived from the outer layer of the optic cup, and the neural retina, derived from the inner layer of the optic cup (see Chapter 7). The pigmented layer is tightly adherent to the choroid throughout, but the neural retina is attached to the pigmented epithelium and thus to the choroid only in a peripapillary ring around the disc and at the ora serrata. Although it contains millions of cell bodies and their processes, the neural retina has the appearance of a thin, transparent membrane.
The retina is the site of transformation of light energy into a neural signal. It contains the first three cells (photoreceptor, bipolar, and ganglion) in the visual pathway, the route by which visual information from the environment reaches the brain for interpretation. Photoreceptor cells transform photons of light into a neural signal through the process of phototransduction, then transfer this signal to bipolar cells, which in turn synapse with ganglion cells, which transmit the signal from the eye. Other retinal cells—horizontal cells, amacrine cells, and interplexiform neurons—modify and integrate the signal before it leaves the eye. This chapter discusses these cells and the detailed anatomy of the retina; the remainder of the visual pathway is described in Chapter 13.
Retinal Histologic Features
Under light microscopy, the retina has a laminar appearance in which 10 layers are evident (Figure 4-1). Closer examination reveals that these are not all “layers,” but rather a single layer of pigmented epithelium and three layers of neuronal cell bodies, between which lie their processes and synapses. This section describes the epithelial layer and the types and functions of the neural cells; the next section discusses the components of each of the 10 so-called retinal layers.
Retinal Pigment Epithelium
The retinal pigment epithelium (RPE), the outermost retinal layer, is a single cell thick and consists of pigmented hexagonal cells. These cells are columnar in the area of the posterior pole and are even longer, narrower, and more densely pigmented in the macular area.1 The cells become larger and more cuboidal as the layer nears the ora serrata, where the transition to the pigmented epithelium of the ciliary body is located. Because of the orientation of the embryologic cells, the basal aspect of the cell is adjacent to the choroid and the apical surface faces the neural retina. The basal aspect contains numerous infoldings and is adherent to its basement membrane, which forms a part of Bruch’s membrane of the choroid; therefore, its attachment to the choroid is strong. Despite its close association with the choroid, the RPE is considered a part of the retina because it is from the same embryologic germ cell layer—neural ectoderm.
The RPE cells contain numerous melanosomes, pigment granules, that extend from the apical area into the middle portion of the cell and somewhat obscure the nucleus, which is located in the basal region. Pigment density differs in various parts of the retina and in individual cells, which can give the fundus a mottled appearance when viewed with the ophthalmoscope. In the retina, melanin is densest in the RPE cells located in the macula and at the equator.2 Other pigmented bodies, lipofuscin granules, contain degradation products of phagocytosis, which increase in number with age.1,3–7 The cell cytoplasm also contains smooth and rough endoplasmic reticulum, Golgi apparatus, mitochondria, and numerous lysosomes.
The apical portion of an RPE cell consists of microvilli that extend into the layer of photoreceptors, enveloping the specialized outer segment tips (Figure 4-2). However, no intercellular junctions connect the RPE and photoreceptor cells. A potential space separates the epithelial cell and the photoreceptor.1 This subretinal space8 is a remnant of the gap formed between the two layers of the optic cup after invagination of the optic vesicle (see Chapter 7).
Terminal bars consisting of zonula occludens and zonula adherens join the RPE cells near their apices.1 Desmosomes are present throughout the layer, and gap junctions between the cells allow for electrical coupling, providing a low-resistance pathway for the passage of ions and metabolites.9
Photoreceptor Cells
Retinal Pigment Epithelium-Neuroretinal Interface
Several factors are involved in maintaining the close approximation between the photoreceptor cell layer and the RPE layer. Passive forces, such as intraocular pressure (IOP), osmotic pressure,10–12 fluid transport across the RPE,13,14 and presence of the vitreous,15 help preserve the position of the neural retina. Interdigitations between the RPE microvilli and the rod and cone outer segments provide a physical closeness between the two entities.1 The material that occupies the extracellular space between the two layers likely provides adhesive forces.16–18
An unstructured, amorphous material was once thought to occupy the extracellular space between the RPE cells and the photoreceptors, and to surround the outer and inner segments of the photoreceptors. More recent studies have described an organized honeycomb-like structure, the interphotoreceptor matrix (IPM), which is composed of proteins and glycosaminoglycans.19 The outer segments are surrounded completely by the IPM and extend through openings in its meshwork. The constituents of the IPM domains around rods differ from those around cones; those surrounding cones are described as a “matrix sheath.”19–21 The domains are believed to be bound together laterally, forming a highly coherent structural unit.19 The IPM constituents are bound tightly to both the epithelial cells and the photoreceptor cells, and may exceed the strength of the RPE cells. In laboratory experiments, a forceful separation between these two layers often ruptures the RPE cell, leaving remnants of pigment attached to the photoreceptors.3,22 The adhesive mechanism is attributed to molecular bonds within this extracellular material.16,18,22–24
The IPM provides a means for the exchange of metabolites25 and for interactions between the two layers.26 In addition, the IPM may be partly responsible for orienting the photoreceptor outer segments for optimal light capture.17 Despite these apparent forces, the interface between the RPE and the photoreceptor layer is the common location of separation in a retinal detachment.
Clinical Comment: Retinal Detachment
When a retinal detachment occurs, the separation usually lies between the RPE cells and the photoreceptors because no intercellular junctions join these cells. The RPE cells remain attached to the choroid and cannot be separated from it without difficulty. Bruch’s membrane contains fibronectin and laminin, large adhesive glycoproteins with many binding sites that help maintain the adherence of RPE cells to the membrane.27 Fluid can accumulate within the subretinal space separating the photoreceptors from the nutrients supplied by the choroid; if the layers are not repositioned quickly, the affected area of photoreceptor cells will necrose. An argon laser often is used to photocoagulate the border of a detachment, producing scar tissue. These act like a “spot weld” and prevent the detachment from enlarging and facilitates the repositioning of the photoreceptors.
Composition of Rods and Cones
Rods and cones are composed of several parts, starting nearest the RPE: (1) the outer segment, containing the visual pigment molecules for the conversion of light into a neural signal; (2) a connecting stalk, the cilium; (3) the inner segment, containing the metabolic apparatus; (4) the outer fiber; (5) the cell body; and (6) the inner fiber, which ends in a synaptic terminal (Figure 4-3).
Outer Segment
The outer segment is made up of a stack of membranous discs (600 to 1000 per rod)2 and is enclosed by the plasmalemma of the cell. Each disc is a flattened membrane sac with a narrow intradisc space; the discs are stacked on top of one another and are separated by an extradisc space. Visual pigment molecules are located within the disc membrane. A biochemical change is initiated within these molecules when they are activated by a photon of light. The tip of the outer segment is oriented toward the RPE, and the base is oriented toward the inner segment.
Cilium
A connecting stalk, or cilium, extends from the innermost disc, joining the outer segment with the inner segment and acting as a conduit between them (Figure 4-4). It is a modified cilium consisting of a series of nine pairs of tubules from which the central pair, usually present in motile cilia, is missing. The plasmalemma around the outer segment is continuous across the cilium with that of the inner segment.
Inner Segment
The inner segment contains cellular structures and can be divided into two parts. The ellipsoid is nearer the outer segment and contains the numerous mitochondria necessary for the many energy-dependent photoreceptor processes. The part closer to the cell body, which is sometimes called the myoid, contains other cellular organelles, such as the endoplasmic reticulum and Golgi apparatus; protein synthesis is concentrated in this area.28 The term “myoid,” however, is derived from a similar area in amphibians that contains a contractile structure that produces orientational movements of the outer segments of the cones.29 The human myoid does not have contractile properties,30 although the axis of the inner and outer segments is oriented toward the exit pupil of the eye, maximizing the ability of the photoreceptor to capture light. The radial orientation becomes more evident in cells located farther from the macula.8,31,32
Rod and Cone Morphology
Rods
The plasmalemma, enclosing the rod outer segment, is separate from the disc membrane except for a small region at the base where invaginations of the plasmalemma form discs, the intradisc space of which is continuous with the extracellular space (see Figure 4-3, A). The remainder of the discs form sacs that are closed at both ends and are free of attachment to the surrounding membrane and adjacent discs.8,33 The discs are fairly uniform in width, and the photosensitive pigment rhodopsin is located within the disc membrane.
Young28 applied a pulse of radiolabeled amino acids, which were incorporated into the disc components. He observed that the band of radioactivity moved from the inner segment, where protein is synthesized, into newly assembled discs in the outer segment. The labeled discs moved from the base to the tip, and the label was finally seen in phagosomes of the RPE cells. This study established that components of disc membranes are produced in the inner segment and move along the connecting stalk to be incorporated into discs at the outer segment base.28 The discs gradually are displaced outward by the formation of new discs and, as they reach the tip, are sloughed off, taken up by the RPE cells, and phagocytosed.34,35 This process, the rod outer segment renewal system, appears to involve active processes in both the RPE and the outer segment.28,36 The discs apparently are shed regularly, with most of the shedding occurring in the early morning.37–40
The rod inner and outer segments are approximately the same width. The inner segment is joined to the cell body by the relatively long and narrow outer fiber. The inner fiber extends from the cell body and terminates in a rounded, pear-shaped structure called a spherule (see Figure 4-3, A). The internal surface of the spherule is invaginated forming a synaptic complex that contains bipolar dendrites and horizontal cell processes.41,42 Rods release the neurotransmitter glutamate.
Cones
As in the rod, the outer segment of the cone is enclosed by a plasmalemma, but in this case the plasma membrane is continuous with the membranes forming most of the discs, and the discs are not separated easily from one another43 (Figure 4-5). In many cones the discs at the base are wider than those at the tip, giving the characteristic cone shape, although some cone outer segments have a shape similar to the rod.
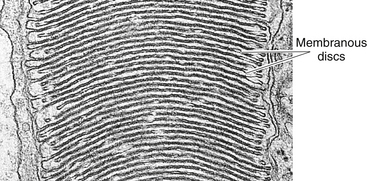
FIGURE 4-5 Cone outer segment. (Transmission electron microscope; ×56,000.)
(From Krause WJ, Cutts JH: Concise text of histology, Baltimore, 1981, Williams & Wilkins.)
The cone outer segment is shorter than that of the rod and may not reach the RPE layer. However, tubular processes protrude from the apical surface of the epithelial cell to surround the cone outer segment.44 One of three visual pigment molecules is contained within the disc membrane, and each pigment molecule is activated by the absorption of light in a specific range in the color spectrum. The peak absorptions occur at 420 nm (blue), 531 nm (green), and 588 nm (red).
Early studies of cone outer segment renewal theorized that the formation of discs at the cone base was not uniform and sequential because radiolabeled protein appeared to be distributed throughout the outer segment membrane, not merely in the disc membranes.35,45 More recently, electron-microscope studies suggest that formation of new discs does occur at the base, but because of the more extensive connections with the surrounding plasmalemma, the labeled molecules are able to diffuse throughout the cone outer segment membrane rather than being confined to discs, as occurs in the rods.43 Investigations continue into the mechanism by which the discs become narrower as they approach the tip.46 Cone discs too are shed periodically, often at the end of the day, and are phagocytosed by the RPE.40,43,47,48 The factors that regulate the cycle of disc shedding are still under investigation.
The shape of the inner segment also contributes to the cone shape. The ellipsoid area of the cone is wider and contains more mitochondria than the rod.1 The outer fiber is short and stout and may even be absent in the cone; thus, cone nuclei lie outer to rod nuclei. The inner fiber terminates in a broad, flattened structure called a pedicle, which has several invaginated areas within its flattened surface (see Figure 4-3, A). Cone pedicles have three types of synaptic contacts. Triads are found within the invaginations; contacts with bipolar cells occur on the flat surfaces; and gap junctions are located on the lateral expansions (telodendria) of the pedicle and permit electrical communication between adjacent rods or cones.8,49
As with rods, the neurotransmitter released by cones is glutamate.
Bipolar Cells
The bipolar cell is the second-order neuron in the visual pathway. The nucleus of the bipolar cell is large and contains minimal cell body cytoplasm. Its dendrite synapses with photoreceptor and horizontal cells, and its axon synapses with ganglion and amacrine cells; glutamate is its neurotransmitter. Bipolar cells relay information from photoreceptors to horizontal, amacrine, and ganglion cells and receive extensive synaptic feedback from amacrine cells.50 Their dendrites also have contact with interplexiform neuron processes.51 Eleven types of bipolar cells have been classified on the basis of morphology, physiology, and dendritic contacts with photoreceptors;51 all types except the rod bipolar cell are associated with cones.
Only one type of rod bipolar cell has been identified. It has a relatively large body and several spiky dendrites, usually arising from a single, thick process. Rod bipolar cells begin to appear 1 mm from the fovea and continue into the periphery. These are the only bipolar cells that contact rods.1,51 The expanse of the dendritic tree widens and the reach of the axonal terminals increases in the rod bipolar cells located in peripheral retina compared with those in central retina. The dendrites of a single rod bipolar cell contact 15 to 20 rods in central retina and up to 80 rods in the periphery, improving sensitivity.51,52 Often two dendrites lie within the spherule invagination flanked by two horizontal cell processes (Figure 4-6). The rod bipolar axon is large and unbranched, and rarely synapses directly with ganglion cells but instead synapses with amacrine processes, which then signal ganglion cells.53 This synaptic arrangement allows a ganglion cell to carry information from both the rod and the cone pathway.53
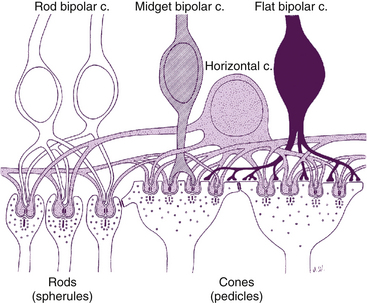
FIGURE 4-6 Rod spherule and cone pedicle and their synapses.
(From Hogan MJ, Alvarado JA, Weddell JE: Histology of the human eye, Philadelphia, 1971, Saunders.)
The midget bipolar cell has a relatively small body and can be either flat or invaginating. Dendritic terminals of the flat midget bipolar cell end in a flat expansion and make contact only with the flat area of the cone pedicle. In central retina, each flat midget bipolar cell contacts only one cone so its dendritic “bouquet” is small—the size of a single cone pedicle. In peripheral retina, each flat midget bipolar cell has two or three dendritic clusters and thus each cell contacts two or three neighboring cones.51,54 A single cone pedicle may have as many as 500 contacts on its flat surface.2 The axon of the flat midget bipolar cell has many endings and synapses with ganglion cells of all types.1
The invaginating midget bipolar cell is similar to the flat midget bipolar cell, but its dendritic processes are located within the pedicle invaginations, usually in groupings called a triad. A triad consists of a single central bipolar dendrite flanked by two horizontal cell processes within an invagination in the cone pedicle (see Figure 4-6). In central retina, the dendritic bouquet of an invaginating midget bipolar cell is the size of a single cone pedicle, implying that each cone is innervated by only one invaginating bipolar cell. Each pedicle can have 12 to 25 triads.2 In peripheral retina, each bipolar cell may have up to three such dendritic expansions, with the capacity to contact several pedicles.51 The axon of the invaginating midget bipolar cell synapses with the dendrite of a single midget ganglion cell and with amacrine processes.1
The two types of diffuse cone bipolar cells are designated type a and type b, called “flat bipolars” and “brush bipolars” by Polyak.55 In the central retina, the diffuse cone bipolar cell contacts approximately five neighboring cones, and in the periphery, each contacts 10 to 15 neighboring cones. The location of the axon terminal differentiates the two types.51
The blue cone bipolar cell synapses with up to three cone pedicles.56 It differs from diffuse cone bipolar cells in that it contacts widely spaced rather than neighboring cones.51
The giant cone bipolar cell derives its name from the extent of its dendritic tree. The major dendrite branches into three trees, and then clusters of processes branch from these, each group being the size of a cone pedicle. The two types, designated diffuse and bistratified, differ only in the location of their axon terminations.51,57
Figure 4-7 shows several of the bipolar cell types.
Ganglion Cells
The next cell in the visual pathway, the third-order neuron, is the ganglion cell. Ganglion cells can be bipolar (e.g., a single axon and a single dendrite) or multipolar (a single axon and more than one dendrite).58 Cell size varies greatly, with some large cell bodies measuring 28 to 36 μm.51 The various methods used to classify ganglion cells make classification rather confusing.
An older, broad classification groups ganglion cells into three types. W cells project to the midbrain, carrying information for the pupillary response and reflexive eye movements. Y cells project to the lateral geniculate nucleus (LGN), with some having collateral branches that travel to the midbrain, perhaps with pupillary information. X cells primarily respond in visual discrimination and project to the LGN.2
Ganglion cells have also been classified on the basis of cell body size, branching characteristics, termination of dendrites, and the expanse of the dendritic tree.51 Figure 4-8 shows several types of ganglion cells, both central and peripheral. These ganglion cell types are designated G3 to G23 (not all numbers are represented).
Another designation classifies ganglion cells based on the lateral geniculate nucleus layer in which they terminate. P cells terminate in the parvocellular layers. The P1 ganglion cell, also called the midget ganglion cell, is the most common P cell. This relatively small cell has a single dendrite and can be differentiated into two types, according to the stratification of the dendritic branching.51 Certain P1 midget cells are connected to only one midget bipolar cell, invaginating or flat, which in turn might be linked to a single cone receptor,59 providing a channel that processes high-contrast detail and color resolution. This situation is likely to occur in the fovea. A convergent pathway occurs in some P1 cells that receive input from two bipolar axons.
The P2 ganglion cell also terminates in the parvocellular layers but has a densely branched, compact dendritic tree that spreads horizontally. These cells can be differentiated into two types depending on the location of the dendrite termination.51
The M-type ganglion cell projects to the magnocellular layers of the LGN. The M cell has coarse dendrites (because of its shape can also be called a parasol ganglion cell) with spiny features, and the dendritic tree enlarges from central to peripheral retina.51
Each ganglion cell has a single axon, which emerges from the cell body and turns to run parallel to the inner surface of the retina; the axon releases glutamate at its synaptic cleft. The axons come together at the optic disc and leave the eye as the optic nerve. The termination for approximately 90% of these axons is the lateral geniculate nucleus; the other 10% project to subthalamic areas involved in processes such as the pupillary reflexes and the circadian rhythm.2,51
The photoreceptor cells, bipolar cells, and ganglion cells carry the neural signal in a three-step pathway through the retina. The neural signal is modified within the retina by other cells that create intraretinal cross-connections, provide feedback information, or integrate retinal function.1
Horizontal Cells
The horizontal cell transfers information in a horizontal direction parallel to the retinal surface. It has one long process, or axon, and several short dendrites with branching terminals; the processes spread out parallel to the retinal surface, and all terminate in the outer plexiform layer (Figure 4-9). Horizontal cells synapse with photoreceptors, bipolar cells, and other horizontal cells. Horizontal cells are joined to each other by an extensive network of gap junctions. One type of horizontal cell synapses only within a cone pedicle in the special triad junction. Horizontal cells can contact bipolar cells lying some distance from the photoreceptor that activated the horizontal cell. Horizontal cells can effect an inhibitory response, thus playing a role in the complex process of visual integration.57,60
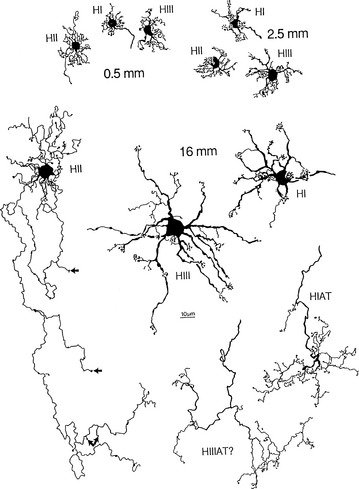
FIGURE 4-9 Whole-mount views of horizontal cells of human retina (scale bar = 10 mm).
(From Kolb H, Linberg KA, Fisher SK: Neurons of the human retina: a Golgi study, J Comp Neurol 318:147, 1992.)
Three types of horizontal cells have been differentiated: HI, HII, and HIII. HI cells have dendrites that synapse with 7 to 18 cones as lateral elements in triads and a large, thick axon ending in a fan-shaped expanse of terminals that end in rod spherules more than 1 mm away.61 All the HII processes (dendrites and axons) apparently contact cones and might be specific for blue cones.51 HIII cells have a large dendritic tree that synapses with many cones, (9 to 12 in the macular area and 20 to 25 in the periphery) not all of which are neighboring; evidence suggests that these horizontal cells avoid blue cones, thus being selective for red and green.51,62 The termination of the HIII axon has not yet been determined but probably contacts both rods and cones.60 Horizontal cells provide inhibitory feedback to photoreceptors or inhibitory feed forward to bipolar cells.61 Horizontal cells can modulate the cone response but are not thought to influence that of the rod.53,61
Amacrine Cells
The amacrine cell has a large cell body, a lobulated nucleus, and a single process with extensive branches that extend into the inner plexiform layer. The process, which has both dendritic and axonal characteristics and carries information horizontally, forms complex synapses with axons of bipolar cells, dendrites and the soma of ganglion cells; with processes of interplexiform neurons; and with other amacrine processes.1,63 Because of the extremely broad spread of its process, the amacrine cell plays an important role in modulating the information that reaches the ganglion cells.57
As many as 30 to 40 different amacrine cell types may be described as stratified or diffuse. They can also be classified into four groups—narrow field, small field, medium field, and large field—according to the extent of coverage by their intertwined branching processes. Each of these groups can be subdivided into different types according to the level of the retinal layer in which their nerve endings terminate.51,64
One of the most widely studied amacrine types is the AII cell (that is Roman numeral 2), a narrow-field type (Figure 4-10). The AII cells are the conduit by which the rod signal reaches ganglion cells.64 An AII cell may receive input from as many as 300 rods through 80 rod bipolar cells.31 The AII cell then synapses with a ganglion cell and may also relay information from the rod pathway to the cone pathway.
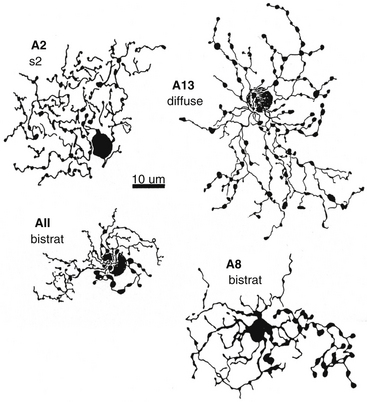
FIGURE 4-10 Whole-mount view of amacrine cells.
(From Kolb H: Amacrine cells of the mammalian retina: neurocircuitry and functional roles, Eye 11:904, 1997.)
Wide-field amacrine (A17) cells form reciprocal synapses with rod bipolar cells and appear to modify the signal transmitted from rod bipolar to AII cells.51 Most amacrine cells contain the inhibitory neurotransmitter gamma-aminobutyric acid (GABA) or glycine, and have both presynaptic and postsynaptic endings.2,64 Amacrine cells are joined to one another via gap junctions,64 and some cells have been found to combine information from rod and cone pathways before innervating a ganglion cell.59
Interplexiform Neurons
The interplexiform neuron has a large cell body and is found among the layer of amacrine cells. The processes extend into both synaptic layers and convey information between these layers (Figure 4-11), apparently providing feedback from inner to outer retinal layers.51,57 Some of their nerve endings are presynaptic and some are postsynaptic to amacrine processes or amacrine cell bodies in the inner plexiform layer. Interplexiform neurons are presynaptic to rod or cone bipolar cells in the outer plexiform layer.2,8,65
Neuroglial Cells
Müller Cells
Müller cells are large neuroglial cells that extend throughout much of the retina. There are 10 million Müller cells in the mammalian retina.66 They play a supportive role, providing structure. The apex of the Müller cell is in the photoreceptor layer, whereas the basal aspect is at the inner retinal surface. Cellular processes form a reticulum among the retinal cell bodies and fill in most of the space of the retina not occupied by neuronal elements (Figure 4-12). Müller cells ensheathe dendritic processes within the synaptic layers, giving structural support, and their processes envelop most ganglion axons.67
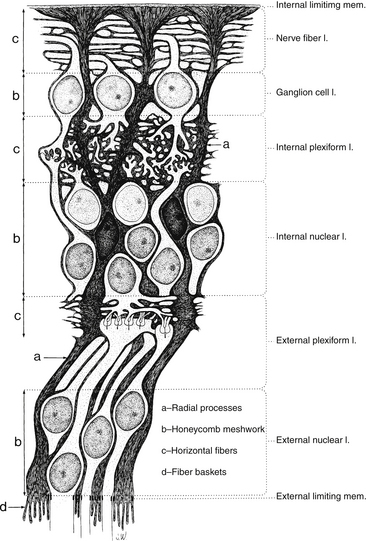
FIGURE 4-12 Structure of the Müller cell (dark gray). mem., membrane; l., layer.
(From Hogan MJ, Alvarado JA, Weddell JE: Histology of the human eye, Philadelphia, 1971, Saunders.)
Neuronal cell bodies and their processes appear to reside in tunnels within the Müller cell.52 Delicate apical villi, fiber baskets (of Schultze), terminate between the inner segments of the photoreceptors.1,29 On light microscopy, Müller cell processes can be seen passing through the layer containing the nerve fibers of the ganglion cells, perpendicular to the retinal surface. An expanded process, called the endfoot, along the basal aspect of the Müller cell contributes to the membrane separating the retina from the vitreous, and extensions of Müller cells wrap around blood vessels.1 The pervasiveness of the Müller cell results in very little extracellular space in the retina (see Figure 4-12). Besides providing structure, the Müller cell acts as a buffer by regulating the concentration of potassium ions (K+); they help maintain the extracellular pH by absorbing metabolic waste products68; they recycle GABA and glutamate, removing them from the extracellular space; and Müller cells metabolize, synthesize, and store glycogen.69–71
Microglial Cells and Astrocytes
Astrocytes are star-shaped fibrous cells found in the inner retina, usually in the nerve fiber and ganglion cell layers. These perivascular cells form an irregular supportive network that encircles nerve fibers and retinal capillaries.1 They may contribute to the internal limiting membrane as well as perform some of the same functions as the Müller cells.72
Ten Retinal Layers
The “10-layered” arrangement of the retina is actually a remarkable organization of alternate groupings of the retinal neurons just described and their processes. Traditionally, descriptive names were given to these so-called layers, and these designations are still in use today (Figure 4-13).
Retinal Pigment Epithelium
The RPE consists of a single layer of pigmented cells, as previously discussed. There are 4 to 6 million RPE cells, and each cell interacts with 30 to 40 photoreceptors.37,73,74 There is little cell division in the layer. The RPE is an active area with several functions that will be described in a later section.
External Limiting Membrane
The external limiting membrane (ELM, outer limiting membrane) is not a true membrane but is actually composed of zonula adherens junctions between photoreceptor cells and between photoreceptors and Müller cells at the level of the inner segments. On light microscopy, the so-called membrane appears as a series of dashes, resembling a fenestrated sheet through which processes of the rods and cones pass. This band of zonula adherens has the potential to act as a metabolic barrier restricting the passage of some large molecules.8,75
Outer Nuclear Layer
The outer nuclear layer (ONL) contains the rod and cone cell bodies; the cone cell body and nucleus are larger than those of the rod. Cone outer fibers are very short, and therefore the cone nuclei lie in a single layer close to the external limiting membrane; cell bodies of the rods are arranged in several rows inner to the cone cell bodies. The ONL is 8 to 9 cells thick on the nasal edge of the optic disc and 4 rows thick at the temporal edge and is thickest in the fovea, where it contains approximately 10 layers of cone nuclei.2
Outer Plexiform Layer
The outer plexiform layer (OPL; also outer synaptic layer) has a wide external band composed of inner fibers of rods and cones and a narrower inner band consisting of synapses between photoreceptor cells and cells from the inner nuclear layer. Rod spherules and cone pedicles synapse with bipolar cell dendrites and horizontal cell processes in the OPL. Many of these synapses consist of invaginations in the photoreceptor terminal; invaginations are deep in the spherule but more superficial in the pedicle.8 In these junctures the photoreceptor element contains a membranous plate, the synaptic ribbon8,59 (Figure 4-14). The invaginating synapse generally has three postsynaptic processes and is called a triad. The lateral elements are horizontal cell processes and are deep within the invagination, a bipolar dendrite is the center process (see Figure 4-6). Invaginating midget bipolar cells are involved in cone triads, and all cones have at least one invaginating midget bipolar and one flat midget bipolar contact.8
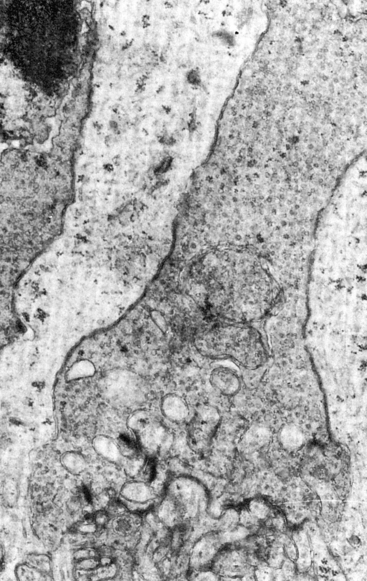
FIGURE 4-14 Electron micrograph of cone pedicle. Note dark linear bodies (synaptic ribbons) in pedicle. (×20,000.)
(From Leeson CR, Leeson ST: Histology, Philadelphia, 1976, Saunders.)
Synaptic contacts also occur outside invaginating synapses in the OPL. Horizontal cells make synaptic contact with bipolar dendrites and contact other horizontal cell processes via gap junctions.61,76 Bipolar dendrites synapse with photoreceptor cell endings; a single photoreceptor can have contact with more than one bipolar dendrite. The relatively long axon of the interplexiform neuron makes numerous synaptic connections with processes entering photoreceptor terminals.65,77
Desmosome-like attachments called synaptic densities are located within the arrangement of interwoven, branching, bipolar dendrites and horizontal cell processes in the OPL. These synaptic densities are seen as a series of dashed lines on light microscopy and resemble a discontinuous membrane, termed the middle limiting membrane. This “membrane” demarcates the extent of the retinal vasculature29 and may prevent retinal exudates and hemorrhages from spreading into the outer retinal layers.52
Inner Nuclear Layer
The inner nuclear layer (INL) consists of the cell bodies of horizontal cells, bipolar cells, amacrine cells, interplexiform neurons, Müller cells, and sometimes displaced ganglion cells. The nuclei of the horizontal cells are located next to the outer plexiform layer, where their processes synapse. The nuclei of the amacrine cells are located next to the inner plexiform layer, where their processes terminate. The bipolar cell has its dendrite in the outer plexiform layer and its axon in the inner plexiform layer (Figure 4-15). The interplexiform neuron also has processes in both synaptic layers. It is thought to receive input in the inner plexiform layer and project it to the outer plexiform layer.65 The retinal vasculature of the deep capillary network is located in the inner nuclear layer.