Respiratory Care Practice Review
Oxygen Therapy
Patients with cardiovascular and pulmonary impairments frequently need oxygen supplementation.1 Respiratory care practitioners and the nursing staff are generally responsible for administration of this drug under a physician’s order. Therapists have a variety of methods from which to choose to deliver oxygen in the most effective way (individual methods are discussed later in this chapter). Long-term oxygen therapy for patients with chronic obstructive pulmonary disease (COPD) who are hypoxemic has been reported to improve quality of life and life expectancy.2–5 However, even though the benefits of long-term oxygen therapy have been established, U.S. congressional mandates for competitive bidding and caps in rental agreement programs have led to cutbacks. As a consequence, the dose of oxygen and its mode of delivery may need to be modified at rest and during activity.6 This is an issue of significant concern. As practitioners working with patients requiring oxygen, physical therapists need to advocate for their patients to ensure that they receive appropriate oxygen therapy.
The purpose of oxygen therapy is to treat and prevent hypoxemia, excessive work of breathing, and excessive myocardial work.7 Although individual oxygen appliances offer suggested guidelines regarding oxygen administration, the only way to ensure effective delivery from a given device is by blood gas monitoring of PaO2 (partial pressure of oxygen in the arterial blood) or monitoring hemoglobin saturation by oximetry. Medicare and third-party payers have specific indications for oxygen therapy. These indications may differ and physical therapists need to identify these in the practice setting and provide the documentation required for patients to receive the oxygen they need. Initially, a patient may need oxygen only with higher levels of activity or exercise; that needs to be documented by an exercise test that shows desaturation by oximetry. As a patient’s disease progresses, continuous oxygen may be indicated. Careful monitoring of patients will determine when the need for continuous oxygen occurs. Oxygen therapy is usually administered by one of two methods: low-flow or high-flow systems.
Low-Flow Systems
A low-flow oxygen system is one that is not intended to meet the total oxygen requirements of the patient (does not deliver atmospheric oxygen concentration to the patient). For example, using a normal minute ventilation (minute ventilation = tidal volume [TV] × respiratory rate [RR]) of 8 L/min (500 mL × 16 breaths per minute) with a patient receiving 1 L/min of oxygen, the patient is breathing 8 L/min but the device only provides 1 L/min. Thus 7 L/min are contributed from room air. Each time there is a change in the patient’s breathing pattern, tidal volume, and respiratory rate, the fraction of inspired oxygen (FiO2) may also change. Ideally, for efficient use of a nasal cannula, the patient should have a normal tidal volume, respiratory rate, and breathing pattern. This is often not the case, but the cannula is used because it is well tolerated by the patient. This method of oxygen delivery is not used when a specific concentration of oxygen is needed.8
Nasal Cannulas
The nasal cannulas (also called nasal prongs) are one of the most common low-flow devices encountered because of low expense and high patient compliance (Figure 43-1). This device supplies an FiO2 of approximately 24% to 40% oxygen with flow rates from 1 L/min to 6 L/min. Flow rates greater than 6 L/min can cause nasal mucosal irritation and drying. The approximate liter flow and resultant FiO2 values are shown in Table 43-1.
Table 43-1
Approximate Liter Flow and Resultant FiO2 Values
Flow (L/min) | FiO2 (%) |
1 | 24 |
2 | 28 |
3 | 32 |
4 | 36 |
5 | 40 |
6 | 44 |
Adapted from the American College of Sports Medicine: Guidelines for exercise testing and prescription, ed 6, Philadelphia: Lippincott, Williams & Wilkins, 2000.
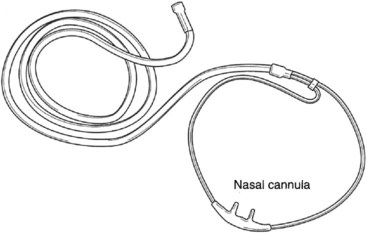
Nasal cannulas deliver 100% oxygen; however, this percentage significantly lessens as the oxygen mixes with inspired air from the room. The amount of oxygen delivered depends on the flow rate and the ventilatory pattern of the patient. A larger minute volume (TV × RR) would dilute the oxygen at any given flow rate and cause a greater decrease in the percentage delivered to the lungs. In other words, the faster and deeper a patient breathes, the more diluted the oxygen becomes. On the other hand, if a patient has a low minute volume, the oxygen percentage delivered increases.7 If precise control of FiO2 is needed, the nasal cannula should not be used.9 In these cases a high-flow system (i.e., a Venturi mask) may be used.
The patient should be encouraged to breathe through the nose to receive maximum benefit from the nasal cannula; however, mouth breathing does not mean the patient will not receive appropriate oxygen.10 Clinically, it is found that some patients who mouth-breathe do so because of nasal polyps, sinus congestion, deviated septum, or other physical issues. If the work of breathing is increased when the patient tries to breathe through the nose, it may be counterproductive.
Simple Mask
The simple mask is another commonly used low-flow oxygen-delivery device. It can deliver from 40% to 60% oxygen, depending on the flow rate and the patient’s ventilatory pattern. This device requires a flow rate of 5 to 6 L/min to prevent rebreathing carbon dioxide and excessive respiratory work.11 As with the nasal cannula, this type of oxygen delivery method should not be used if precise control of oxygen concentration (FiO2) is required because the simply mask is still considered a low-flow oxygen system.
Partial Rebreathing Mask
The partial rebreathing mask is basically a mask with a reservoir bag attached. The oxygen source supplies the bag with 100% oxygen, which then mixes with exhaled anatomical dead-space air that has not taken place in gas exchange. This exhaled air is rich in oxygen. The exhaled air that has taken place in gas exchange and contains CO2 is vented through the open port on each side of the mask. This mask can deliver oxygen concentrations from 70% to more than 80%. It requires flows between 7 and 10 L/min to keep the bag from fully collapsing during inspiration.7
Nonrebreathing Mask
Another low-flow delivery device, the nonrebreathing mask, also contains a bag reservoir, but it can deliver up to 100% oxygen. This mask has valves on the reservoir bag and side vents. This feature prevents ambient air mixing on inspiration and exhaled air mixing on expiration. For this mask to operate effectively, a good seal between the patient’s face and the mask must be achieved. The bag should partially deflate during inspiration (Figure 43-2).
Transtracheal Oxygen Catheter
Another form of low-flow oxygen delivery is the transtracheal oxygen catheter. This has been used for pediatric and adult patients.12,13 It is a cannula that is surgically placed into the trachea via a small incision between the second and third tracheal rings. Generally these devices are used by patients at home who need continuous oxygen therapy. Transtracheal oxygen catheters are more efficient than nasal cannulas, and they have a high patient acceptance rate with low complications.14 Because the oxygen is administered directly into the trachea, 50% less oxygen is needed.7 For people who use portable oxygen systems and for those who require high oxygen flow rates, transtracheal oxygen catheters may be beneficial.15 The catheter can be covered by the patient’s clothing and is therefore cosmetically appealing. Complications such as plugging of the catheter and subcutaneous emphysema have been reported.16,17 These complications may be avoided with proper care of the catheter, good hydration, and maintenance as directed by the patient’s health care team. The transtracheal procedure is not routine in many practice settings; thus if a patient is interested in this form of oxygen therapy, it is wise to find a team that has done the procedure frequently enough to become highly skilled at it.
High-Flow Systems
Venturi Mask
The Venturi mask is a common method of delivering high-flow oxygen concentrations from 24% to 50%. This mask operates via the Venturi principle, which provides for a mixing of 100% oxygen and entrained ambient air. The oxygen flows though a narrow orifice at a high velocity, causing a subatmospheric pressure. This drop in pressure is what causes the ambient air to be entrained through a port. The size of the port determines the amount of air that is entrained, thus the percentage of oxygen delivered. The Venturi mask has a rotating air entrainment port that allows the health care provider to “dial in” the desired FiO2 (Figure 43-3). Flow rates of 40 to 80+ L/min provide minute ventilation at rates that are higher than those the patient could breathe without assistance; consequently, the mask provides the entire inspired atmosphere to the patient. This means that regardless of the patient’s respiratory rate, tidal volume, or breathing pattern, the oxygen delivery (FiO2) will be consistent.
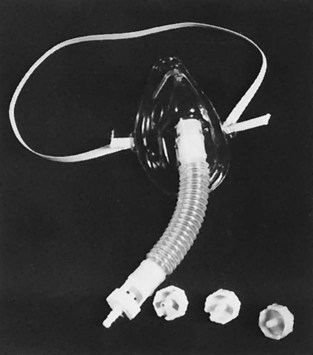
Mechanical aerosol systems also operate via air entrainment, but the mask is connected to the aerosol unit by large-bore tubing to allow a specific FiO2 to be delivered with high humidity (Figure 43-4). Although drainage bags are usually attached to the tubing to collect condensation, the tubing must be monitored for possible pooling of water, which causes flow obstruction to the patient. If partial obstruction occurs, a mild back pressure results in the tubing, causing less air entrainment. This means that a higher concentration of oxygen results and potentially a higher dosage of oxygen than desired may be delivered to the patient.
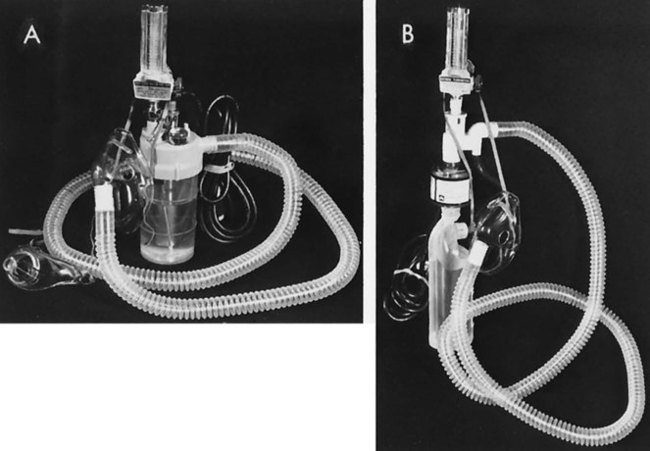
Portable Oxygen
Home oxygen-delivery systems include high-pressure oxygen cylinders, low-pressure liquid oxygen,18 and oxygen concentrators.8 Additional options include continuous flow versus demand or pulsed oxygen delivery.19–21 There are also a number of oxygen-conserving devices from which to choose.22 When a continuous system is changed to either a demand or pulsed system, or when a new oxygen-conserving device is started, the patient must be monitored carefully. Clinical experience has shown that some patients may not tolerate the same liter flow with a pulsed system, and oximetry and perceived exertion must be evaluated when there is any change in oxygen delivery. The oxygen delivery may not be as effective as a continuous flow device when changing to a pulsed oxygen system. Unfortunately, there is no mandate for clinical testing of the devices before they come to market, so documented effectiveness of one versus another in clinical use is not available.23
Heliox, a combination of 21% oxygen and 79% helium, has been used in acute care for patients who have upper airway obstruction and increased work of breathing (spontaneously breathing patients, as well as those on a ventilator). However, use of heliox has not been widespread because of its cost, which has been estimated to be 13 times that of oxygen. Heliox for portable oxygen and home use has been documented as effective for patients with asthma and COPD. It has been shown to decrease airway resistance and decrease the work of breathing and has also been effective with patients who have increased mucus secretions. Studies have demonstrated an increase in patients’ ability to exercise using heliox; however, it has not been shown to significantly decrease dyspnea.24–26
Oxygen concentrators are commonly used in the home health setting, especially because Medicare offers coverage for these devices (Figure 43-5). They are electrically powered and create oxygen by drawing ambient air across a semipermeable membrane, separating oxygen from nitrogen. They generally operate at 2 L/min and provide FiO2 90% oxygen output. Long oxygen tubing, up to 50 feet, allows patients extra mobility. Patients using concentrators should have a back-up device, such as a portable tank, in case of a power outage. In addition, the electrical power company should be notified of any patient who is using oxygen by concentrator so that the patient’s location is designated as a high priority for backup and restoration of power if an outage occurs.