Respiratory and Cardiovascular Drug Actions
This chapter describes much of the basic respiratory and cardiovascular physiology that underlies the action of the drugs presented, with the goal of elucidating the relationship between basic physiology and the drug mechanism of action (Table 45-1). Some of the trade names of the medications mentioned in the text will be changing, but the generic names will remain the same. Not all pharmacological interventions for the respiratory and cardiovascular systems are covered. Certainly, no attempt has been made to describe the pharmacology of other systems or disease states. For further study, any of the texts listed in the reference section at the end of the chapter are highly recommended.
Table 45-1
Respiratory and Cardiovascular Drugs
Sympathetic neurotransmission | Adrenergic (sympathomimetic) drugs | Norepinephrine |
Epinephrine | ||
Isoproterenol | ||
Phenylephrine and metaraminol | ||
Ephedrine | ||
Amphetamine | ||
Beta2-receptor stimulants | ||
Alpha-adrenergic blocking drugs | Phentolamine | |
Phenoxybenzamine | ||
Prazosin, doxazosin, and terazosin | ||
Beta-adrenergic blocking drugs | Propranolol | |
Metoprolol and atenolol | ||
Sympatholytic drugs | Reserpine | |
Guanethidine | ||
Methyldopa and clonidine | ||
Parasympathetic neurotransmission | Cholinergic drugs | Acetylcholine |
Bethanecol | ||
Carbachol | ||
Pilocarpine | ||
Anticholinesterase drugs | Physostigmine | |
Neostigmine | ||
Edrophonium | ||
Donepezil, rivastigmine, and galantamine | ||
Cholinergic blocking drugs | Atropine | |
Ipratropium and tiotropium | ||
Homatropine and cyclopentolate | ||
Dicyclomine | ||
Trihexyphenidyl HCL and benztropine mesylate | ||
Trimethaphan and mecamylamine | ||
Special considerations | Adrenergic (sympathomimetic) drugs | Epinephrine |
Ephedrine | ||
Albuterol, metaproterenol, terbutaline, pirbuterol, formoterol, and salmeterol | ||
Anticholinergics | Ipratropium and tiotropium | |
Methylxanthines | Theophylline and aminophylline | |
Corticosteroids | Budesonide, flunisolide, fluticasone, and triamcinolone | |
Mediator inhibitors | Cromolyn sodium and nedocromil sodium | |
Antileukotrienes | Zileuton, zafirlukast, and montelukast | |
Mucolytics and expectorants | Acetylcysteine | |
Guaifenesin |
Sympathetic Neurotransmission
< ?xml:namespace prefix = "mml" />

This expression, cardiac output, is a common one, and it constitutes half of the blood pressure regulation equation: CO × TPR = BP, where CO is cardiac output, TPR is total peripheral resistance, and BP is blood pressure. TPR is determined by vasoconstriction or vasodilation in the arterioles. For example, vasoconstriction increases resistance; therefore TPR and BP go up.
Adrenergic (Sympathomimetic) Drugs
Norepinephrine
Beta2-Receptor Stimulants
As mentioned above, there are several drugs that act primarily at the beta2 smooth muscle receptor site, causing selective actions in the bronchioles and arterioles but not in the heart (Box 45-1). These drugs will produce a bronchodilation without increasing cardiac output. This particular lack of cardiovascular effect makes them safer than drugs like isoproterenol or ephedrine in treatment of bronchial asthma. This class of drugs is currently part of the mainstay of treatment for asthma and chronic obstructive pulmonary disease (COPD).
Beta-Adrenergic Blocking Drugs
Metoprolol and Atenolol
Metoprolol (Lopressor) and atenolol (Tenormin) are beta-adrenergic antagonists that have been designed to be cardioselective. This offers an advantage in safety when a beta-blocker must be used in patients with asthma because this disease would be aggravated if respiratory beta-receptors were blocked. The natural beta-adrenergic agonist, epinephrine, is a bronchodilator, and an important class of bronchodilating drugs acts at bronchiolar beta-adrenergic receptors.1
Parasympathetic Neurotransmission
Cholinergic Drugs
Acetylcholine
ACh is the endogenous cholinergic transmitter that accounts for nicotinic and muscarinic actions within the autonomic nervous system (Table 45-2). It is rapidly hydrolyzed by acetylcholinesterase and the nonspecific cholinesterase and therefore has a short duration of action if administered parenterally. Therefore, in general, this drug is not very efficacious.
Table 45-2
*With all of these toxic effects, atropine, a competitive muscarinic blocker, is the antidote of choice.
Anticholinesterase Drugs
Further effects of anticholinesterases are related to nicotinic functions of ACh. These include skeletal muscle twitching, weakness, and paralysis. CNS effects include depression of the respiratory and cardiovascular control centers, leading to respiratory collapse. At the time of death, respiratory paralysis is evident, caused by a combination of bronchoconstriction, bronchosecretions, respiratory muscle paralysis from overstimulation, and CNS/control center depression. The treatment of this toxicity is closely related to preserving respiratory function. Administration of atropine, a muscarinic blocker, will effectively decrease bronchoconstriction and secretion. Another drug, pralidoxime (Protopam), is used to reactivate the acetylcholinesterase. It is most effective shortly after exposure to the toxic agent because it breaks down the anticholinesterase so it can be removed from the enzyme site. Additional measures are related to physiological support of the patient. Maintenance of an airway, artificial respiration, and oxygen administration are important therapeutic applications for these patients.2
Cholinergic Blocking Drugs
Cardiovascular Reflex
Functional Reflex
As an example to demonstrate this reflex, let us assume we have just experienced a loss of blood pressure. The carotid baroreceptors shorten and slow their firing. This message is then delivered to the brain, and the vasomotor center responds by increasing sympathetic nerve activity. This control always responds to information regarding blood pressure in the carotid artery by directly opposing the baroreceptors. If the pressure had risen, the baroreceptors would have increased their firing rate and the vasomotor center would have responded by slowing the sympathetic nerve firing rate. Because blood pressure in this example is low, the vasomotor center increases sympathetic nerve firing, resulting in increased release of norepinephrine from the nerves that reach the heart and arterioles. Norepinephrine increases the heart rate and stroke volume, thereby producing an increase in cardiac output. In the arterioles, norepinephrine stimulates the alpha-receptors, producing vasoconstriction, which results in increased resistance and ultimately raised blood pressure. A third component of the sympathetic nervous system can be involved during sympathetic activation: epinephrine released from the adrenal medulla also increases cardiac output. Meanwhile the vagal nucleus responds to the decreased baroreceptor firing by decreasing its own activity. The vagus nerve to the heart releases less ACh, allowing the sympathetic effect to predominate. The total effect becomes an increase in blood pressure, which is the response required to return the systemic pressure to normal. If the original pressure alteration had been an increase to above normal blood pressure, the opposite reflexive actions would have occurred to return pressure to a normal range. The predominant effect would have been an increase in vagal nerve tone to release high amounts of ACh at the heart, causing a dramatic slowing of heart rate accompanied by a decrease in stroke volume. This combination produces a decrease in cardiac output. The sympathetic system would have responded to the increased baroreceptor firing by decreasing firing in all sympathetic nerves, thereby decreasing norepinephrine and epinephrine release. All of these factors combine to decrease blood pressure to normal.2
Other Drug Classes Used in the Treatment of Hypertension
As mentioned in the previous sections, many drugs have the potential to affect blood pressure, cardiac output, heart rate, etc. Many studies have been done to establish appropriate guidelines for patients with hypertension, which is an important risk factor for heart disease and stroke. The Antihypertensive and Lipid Lowering Treatment to Prevent Heart Attack Trial (ALLHAT) found that it takes at least two medications of different classes to treat most cases of mild hypertension, and three to four medications for those with severe hypertension.4 The latest Joint National Committee JNC report (2003)5 recommends starting patients on a two-drug regimen in which one of the drugs is a diuretic. There are many types of diuretics, all classified according to which part of the kidney is affected and what type of action is performed. Some examples are hydrochlorothiazide (HCTZ) (HydroDIURIL), furosemide (Lasix), and spironolactone (Aldactone). In addition to the diuretics and beta-blockers discussed previously, there are angiotensin-converting enzyme (ACE) inhibitors, angiotensin II receptor blockers (ARBs), calcium-channel blockers, and many drugs that combine two of these classes. These drugs are commonly used for the treatment of hypertension but are also used in heart failure and other heart diseases. Recently, more focus has been put on hypertension in the context of overall cardiovascular risk and the importance of the long view. Evidence from the Anglo-Scandinavian Cardiac Outcomes Trial (ASCOT)6 shows that in addition to blood pressure lowering medications, cholesterol-lowering medications (such as the statins) should be considered as part of primary therapy in certain patients.
ACE inhibitors and ARBs affect a cascade of events that ultimately leads to an increase in blood pressure. This is known as the renin-angiotensin system. The endogenous components of this system effect vascular tone and thereby affect blood pressure. Renin is an enzyme produced by the kidneys and released in response to changes in blood pressure. When renin is circulated throughout the blood stream, it comes in contact with angiotensinogen, which is synthesized by the liver and circulates throughout the body. The angiotensinogen is then converted to angiotensin I. With the aid of ACE, angiotensin I is converted to angiotensin II, which has vasoconstricting effects. Bradykinins are inflammatory mediators that are also broken down by ACE. ACE inhibitors block the enzyme and do not allow angiotensin I to be converted or bradykinins to be broken down.7 Excessive bradykinins can cause many systemic effects, one of them being chronic cough. Some patients need to be taken off their ACE inhibitor for that reason. Two examples of the many ACE inhibitors commonly used are enalapril (Vasotec) and lisinopril (Prinivil). There has been a significant debate over the recommendation for first-line drugs in the treatment of hypertension. Some feel beta-blockers should be used, and others feel ACE inhibitors are a better first line medication, but most agree that both should be used in combination with diuretics. Many ongoing studies continue to address those options. The JNC guidelines primarily are responsible for publishing these recommendations, and healthcare providers should frequently check for updates by going to the National Institute of Health (NIH) website (http://www.nih.gov/) and searching for “JNC Guidelines.”
Drugs Used in Obstructive Airway Disease
Adrenergic (Sympathomimetic) Drugs
Epinephrine
The muscle-relaxant bronchodilator effect attributed to the beta-adrenergic sympathomimetic compounds is most commonly required for asthmatic or allergic emergencies. During a severe exacerbation of asthma, when a patient is unable to coordinate aerosolized treatments, epinephrine can be given via subcutaneous routes. It is commonly used in systemic anaphylactic reactions as well. As mentioned earlier, epinephrine has 50% beta-adrenergic activity and exerts a great bronchodilating effect. Epinephrine may cause an anxiety reaction in a patient, along with headache, palpitations, and respiratory difficulty. It can also cause serious cardiac reactions (dysrhythmias), which have resulted in death.3
Corticosteroids
Budesonide, Flunisolide, Fluticasone, and Triamcinolone
In the treatment of persistent asthma, inhaled steroids are shown to be effective and safer treatments. They do not modify the progression of the disease; however, they improve the day-to-day symptoms. Some examples of these are budesonide (Pulmicort), flunisolide (Aerobid), fluticasone (Flovent), and triamcinolone (Azmacort). Oral steroids such as methylprednisolone or prednisone are reserved for acute exacerbations of asthma. In a life-threatening situation, intravenous methylprednisolone can be given and then switched to oral administration and tapered off over 2 to 3 weeks. If the patient is not on an inhaled steroid at the time, one is initiated. In rare cases when a patient has continued difficulty controlling asthma, the steroids can be taken every other day. This type of treatment is not routinely recommended because of the deleterious side effects of chronic steroid use. In the treatment of severe COPD with multiple exacerbations, evidence shows that inhaled steroids in combination with beta2 agonists reduce the frequency and severity of the exacerbations. As mentioned in the previous section, an example of a steroid/beta2 agonist is fluticasone/salmeterol (Advair). As in the treatment of asthma, oral steroids (methlyprednisolone, prednisone) are reserved for acute exacerbations of COPD, and usually patients are sent home on a short, tapered course.8
Mediator Inhibitors
Cromolyn Sodium and Nedocromil Sodium
Cromolyn sodium and nedocromil sodium can prevent asthma attacks that are normally triggered by specific stimulants, such as exercise or allergen exposure. The mechanism of action is presumed to be regulation of mast cell mediator release and eosinophil recruitment, therefore inhibiting responses to allergen challenge and exercise-induced bronchospasm. These drugs are administered by inhalation and are frequently used in the pediatric population and/or when a specific stimulant is identified. The antileukotrienes are becoming more popular for nonsteroid long-term treatment of asthma, slowly replacing this class of drugs.8
Drug Inhalation Devices
Most drugs administered by inhalation in respiratory disease are delivered by pressurized metered-dose inhalers (MDI) (Figure 45-1) or nebulizers. MDIs rely on pressurized chlorofluorocarbon or hydrofluoroalkane propellants to deliver very small drug particles (less than 5-micron diameter) into the airway. Each activation of the metered valve allows an accurately determined volume (dose) of propellant and drug mixture to be released at a high velocity. As of 2010, most CFC based MDIs have been removed from the market in attempts to protect the environment.9 A nebulizer is a machine that delivers the drug after it has been aerosolized with room air. One important advantage of a nebulizer is that the patient does not need hand/breathing coordination, which is further discussed below. The nebulizer, however, is not portable like an MDI and is more expensive than an MDI.
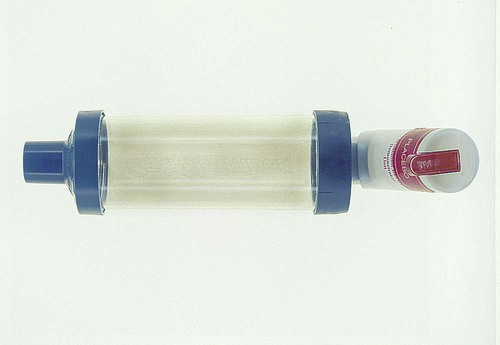
The delivery of drugs to the site of action in the airway by any type of inhalation is inefficient, and therefore, correct technique in MDI use is an important concern. The MDI will allow delivery of 20% of the metered drug to the airway if the patient correctly coordinates the proper rate of inhalation with activation of the device and then adequately holds his or her breath before exhalation. Unfortunately, it is estimated that 50% or fewer patients manage the drug delivery correctly. In response, patient education on correct MDI technique is an important part of therapy, and additional devices, called spacers, have been developed to allow success with variations in technique. The spacers come in various configurations, but they all provide a chamber between the patient and the MDI, which can be charged with the drug-propellant mixture that is then inhaled without concern for critical timing. Patients with poor MDI technique should benefit from the addition of a spacer (see Figure 45-1).
An alternative to the MDI, for patients who have difficulty with the inhalation technique, is the single-dose or multi-dose powder inhaler (Figure 45-2). This device offers a drug compounded into a fine powder that is delivered from a container on simple inhalation by the patient. Coordination of drug delivery is simple and not a problem because only inspiration by the patient drives powder delivery to the airway. The method offers the same or lower efficacy of drug delivery (6% to 20%) when compared with MDI. Also, a relatively high airflow is needed to suspend the powder properly. Therefore young children and the older adults may not benefit from this type of device. Overall, studies have shown that if the patient uses the correct technique, each of the delivery devices provides similar outcomes.10
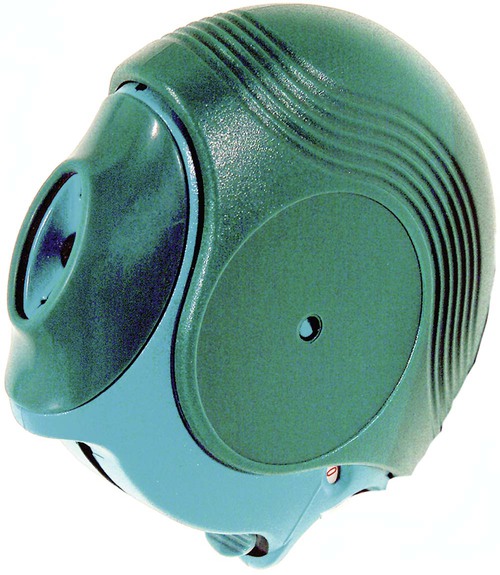