Pulmonary Disorders
Understanding the pathology of the disease, the areas of assessment on which to focus, and the usual medical management allows the critical care nurse to more accurately anticipate and plan nursing interventions. This chapter focuses on pulmonary disorders commonly seen in the critical care environment.
Acute Lung Failure
Description
Acute lung failure (ALF),1 also known as acute respiratory failure, is a clinical condition in which the pulmonary system fails to maintain adequate gas exchange.1,2 It is the most common type of organ failure seen in the critical care unit, with approximately 56% of the patients in the critical care unit experiencing it.1 The mortality rate for patients with ALF is between 30% to 40%, with more than one third of patients not surviving to discharge.3
ALF results from a deficiency in the performance of the pulmonary system (Fig. 20-1).2,4 It usually occurs secondary to another disorder that has altered the normal function of the pulmonary system in such a way as to decrease the ventilatory drive, decrease muscle strength, decrease chest wall elasticity, decrease the lung’s capacity for gas exchange, increase airway resistance, or increase metabolic oxygen requirements.1,5
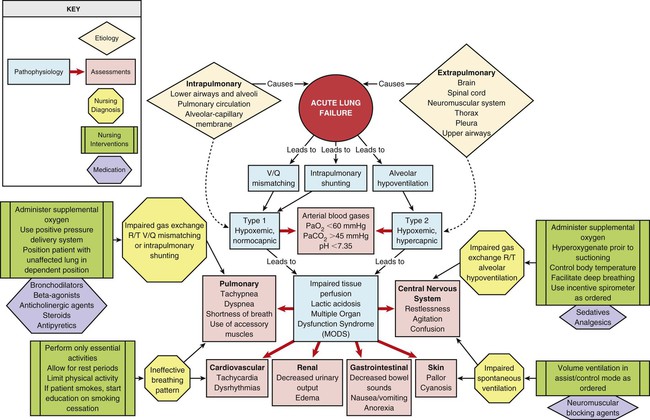
ALF can be classified as hypoxemic normocapnic respiratory failure (type I) or hypoxemic hypercapnic respiratory failure (type II), depending on analysis of the patient’s arterial blood gases (ABGs). In type I respiratory failure, the patient presents with a low Pao2 and a normal Paco2, whereas in type II respiratory failure, Pao2 is low and Paco2 is high.2,4
Etiology
The etiologies of ALF may be classified as extrapulmonary or intrapulmonary, depending on the component of the respiratory system that is affected. Extrapulmonary causes include disorders that affect the brain, the spinal cord, the neuromuscular system, the thorax, the pleura, and the upper airways. Intrapulmonary causes include disorders that affect the lower airways and alveoli, the pulmonary circulation, and the alveolar-capillary membrane.1,6 Table 20-1 lists the different etiologies of ALF and their associated disorders.
TABLE 20-1
ETIOLOGIES OF ACUTE LUNG FAILURE
AFFECTED AREA | DISORDERS* |
Extrapulmonary | |
Brain | Drug overdose |
Central alveolar hypoventilation syndrome | |
Brain trauma or lesion | |
Postoperative anesthesia depression | |
Spinal cord | Guillain-Barré syndrome |
Poliomyelitis | |
Amyotrophic lateral sclerosis | |
Spinal cord trauma or lesion | |
Neuromuscular system | Myasthenia gravis |
Multiple sclerosis | |
Neuromuscular-blocking antibiotics | |
Organophosphate poisoning | |
Muscular dystrophy | |
Thorax | Massive obesity |
Chest trauma | |
Pleura | Pleural effusion |
Pneumothorax | |
Upper airways | Sleep apnea |
Tracheal obstruction | |
Epiglottitis | |
Intrapulmonary | |
Lower airways and alveoli | Chronic obstructive pulmonary disease (COPD) |
Asthma | |
Bronchiolitis | |
Cystic fibrosis | |
Pneumonia | |
Pulmonary circulation | Pulmonary emboli |
Alveolar-capillary membrane | Acute respiratory distress syndrome (ARDS) |
Inhalation of toxic gases | |
Near-drowning |
Pathophysiology
Hypoxemia is the result of impaired gas exchange and is the hallmark of ALF. Hypercapnia may be present, depending on the underlying cause of the problem. The main causes of hypoxemia are alveolar hypoventilation, ventilation/perfusion (V/Q) mismatching, and intrapulmonary shunting.1,2,7 Type I respiratory failure usually results from V/Q mismatching and intrapulmonary shunting, whereas type II respiratory failure usually results from alveolar hypoventilation, which may or may not be accompanied by V/Q mismatching and intrapulmonary shunting.2
Alveolar Hypoventilation
Alveolar hypoventilation occurs when the amount of oxygen being brought into the alveoli is insufficient to meet the metabolic needs of the body.6 This can be the result of increasing metabolic oxygen needs or decreasing ventilation.5 Hypoxemia caused by alveolar hypoventilation is associated with hypercapnia and commonly results from extrapulmonary disorders.1,2,7
Ventilation/Perfusion Mismatching
V/Q mismatching occurs when ventilation and blood flow are mismatched in various regions of the lung in excess of what is normal. Blood passes through alveoli that are underventilated for the given amount of perfusion, leaving these areas with a lower-than-normal amount of oxygen. V/Q mismatching is the most common cause of hypoxemia and is usually the result of alveoli that are partially collapsed or partially filled with fluid.1,2,7
Intrapulmonary Shunting
The extreme form of V/Q mismatching, intrapulmonary shunting, occurs when blood reaches the arterial system without participating in gas exchange. The mixing of unoxygenated (shunted) blood and oxygenated blood lowers the average level of oxygen present in the blood. Intrapulmonary shunting occurs when blood passes through a portion of a lung that is not ventilated. This may be the result of 1) alveolar collapse secondary to atelectasis; or 2) alveolar flooding with pus, blood, or fluid.1,2,7
If allowed to progress, hypoxemia can result in a deficit of oxygen at the cellular level. As the tissue demands for oxygen continue and the supply diminishes, an oxygen supply/demand imbalance occurs and tissue hypoxia develops. Decreased oxygen to the cells contributes to impaired tissue perfusion and the development of lactic acidosis and multiple organ dysfunction syndrome.8
Assessment and Diagnosis
The patient with ALF may experience a variety of clinical manifestations, depending on the underlying cause and the extent of tissue hypoxia. The clinical manifestations commonly seen in the patient with ALF are usually related to the development of hypoxemia, hypercapnia, and acidosis (Fig. 20-2).9 Because the clinical symptoms are so varied, they are not considered reliable in predicting the degree of hypoxemia or hypercapnia2 or the severity of ALF.
Diagnosing and following the course of respiratory failure is best accomplished by ABG analysis. ABG analysis confirms the level of Paco2, Pao2, and blood pH. ALF is generally accepted as being present when the Pao2 is less than 60 mm Hg. If the patient is also experiencing hypercapnia, the Paco2 will be greater than 45 mm Hg. In patients with chronically elevated Paco2 levels, these criteria must be broadened to include a pH less than 7.35.9
A variety of additional tests are performed depending on the patient’s underlying condition. These include bronchoscopy for airway surveillance or specimen retrieval, chest radiography, thoracic ultrasound, thoracic computed tomography (CT), and selected lung function studies.10
Medical Management
Medical management of the patient with ALF is aimed at treating the underlying cause, promoting adequate gas exchange, correcting acidosis, initiating nutrition support, and preventing complications. Medical interventions to promote gas exchange are aimed at improving oxygenation and ventilation.1
Oxygenation
Actions to improve oxygenation include supplemental oxygen administration, either with a low flow system or a high flow system,11 and the use of positive airway pressure.12 The purpose of oxygen therapy is to correct hypoxemia, and although the absolute level of hypoxemia varies in each patient, most treatment approaches aim to keep the arterial hemoglobin oxygen saturation greater than 90%.9 The goal is to keep the tissues’ needs satisfied but not produce hypercapnia or oxygen toxicity.9 Supplemental oxygen administration is effective in treating hypoxemia related to alveolar hypoventilation and V/Q mismatching. When intrapulmonary shunting exists, supplemental oxygen alone is ineffective.11 In this situation, positive pressure is necessary to open collapsed alveoli and facilitate their participation in gas exchange. Positive pressure is delivered via invasive and noninvasive mechanical ventilation. To avoid intubation, positive pressure is usually administered initially noninvasively via a mask.13 For further information on supplemental oxygen therapy and noninvasive ventilation, see Chapter 21.
Ventilation
Interventions to improve ventilation include the use of noninvasive and invasive mechanical ventilation. Depending on the underlying cause and the severity of the ALF, the patient may be treated initially with noninvasive ventilation.13 However, one study found that those patients with a pH of less than 7.25 at initial presentation had an increased likelihood of the need for invasive mechanical ventilation.14 The selection of ventilatory mode and settings depends on the patient’s underlying condition, severity of respiratory failure, and body size. Initially the patient is started on volume ventilation in the assist/control mode. In the patient with chronic hypercapnia, the settings should be adjusted to keep the ABG values within the parameters expected to be maintained by the patient after extubation.15 For further information on mechanical ventilation see Chapter 21.
Pharmacology
Medications to facilitate dilation of the airways may also be of benefit in the treatment of the patient with ALF. Bronchodilators, such as beta2-agonists and anticholinergic agents, aid in smooth muscle relaxation and are of particular benefit to patients with airflow limitations. Methylxanthines, such as aminophylline, are no longer recommended because of their negative side effects. Steroids also are often administered to decrease airway inflammation and enhance the effects of the beta2-agonists. Mucolytics and expectorants are also no longer used since they have been found to be of no benefit in this patient population.16
Sedation is necessary in many patients to assist with maintaining adequate ventilation. It can be used to comfort the patient and decrease the work of breathing, particularly if the patient is fighting the ventilator. Analgesics should be administered for pain control.17,18 In some patients, sedation does not decrease spontaneous respiratory efforts enough to allow adequate ventilation. Neuromuscular paralysis may be necessary to facilitate optimal ventilation. Paralysis also may be necessary to decrease oxygen consumption in the severely compromised patient.18
Acidosis
Acidosis may occur in the patient for a number of reasons. Hypoxemia causes impaired tissue perfusion, which leads to the production of lactic acid and the development of metabolic acidosis. Impaired ventilation leads to the accumulation of carbon dioxide and the development of respiratory acidosis. Once the patient is adequately oxygenated and ventilated, the acidosis should correct itself. The use of sodium bicarbonate to correct the acidosis has been shown to be of minimal benefit to the patient and thus is no longer recommended as first-line treatment. Bicarbonate therapy shifts the oxygen-hemoglobin dissociation curve to the left and can worsen tissue hypoxia. Sodium bicarbonate may be used if the acidosis is severe (pH less than 7.1), refractory to therapy, and causing dysrhythmias or hemodynamic instability.19
Nutrition Support
The initiation of nutrition support is of utmost importance in the management of the patient with ALF. The goals of nutrition support are to meet the overall nutritional needs of the patient while avoiding overfeeding, to prevent nutrition delivery-related complications, and to improve patient outcomes.20 Failure to provide the patient with adequate nutrition support results in the development of malnutrition. Both malnutrition and overfeeding can interfere with the performance of the pulmonary system, further perpetuating ALF. Malnutrition decreases the patient’s ventilatory drive and muscle strength, whereas overfeeding increases carbon dioxide production, which then increases the patient’s ventilatory demand, resulting in respiratory muscle fatigue.21
The enteral route is the preferred method of nutrition administration. If the patient cannot tolerate enteral feedings or cannot receive enough nutrients enterally, he or she will be started on parenteral nutrition. Because the parenteral route is associated with a higher rate of complications, the goal is to switch to enteral feedings as soon as the patient can tolerate them.20,21 Nutrition support should be initiated before the third day of mechanical ventilation for the well-nourished patient and within 24 hours for the malnourished patient.20,21
Complications
The patient with ALF may experience a number of complications including ischemic-anoxic encephalopathy,22 cardiac dysrhythmias,23 venous thromboembolism (VTE),24 and gastrointestinal bleeding.25 Ischemic-anoxic encephalopathy results from hypoxemia, hypercapnia, and acidosis.22 Dysrhythmias are precipitated by hypoxemia, acidosis, electrolyte imbalances, and the administration of beta2-agonists.23 Maintaining oxygenation, normalizing electrolytes, and monitoring medication levels will facilitate the prevention and treatment of encephalopathy and dysrhythmias.22,23 VTE is precipitated by venous stasis resulting from immobility and can be prevented through the use of intermittent pneumatic compression devices and low-dose unfractionated heparin or low–molecular-weight heparin (LMWH).24 Gastrointestinal bleeding can be prevented through the use of histamine receptor antagonists, cytoprotective agents, or proton pump inhibitors.25 In addition, the patient is at risk for the complications associated with an artificial airway, mechanical ventilation, enteral and parenteral nutrition, and peripheral arterial cannulation.
Nursing Management
Nursing management of the patient with ALF incorporates a variety of nursing diagnoses (Box 20-1). Nursing care is directed by the specific cause of the respiratory failure, although some common interventions are used. The nurse has a significant role in optimizing oxygenation and ventilation, providing comfort and emotional support, maintaining surveillance for complications, and educating the patient and family.
Optimizing Oxygenation and Ventilation
Nursing interventions to optimize oxygenation and ventilation include positioning, preventing desaturation, and promoting secretion clearance.
Positioning.
Positioning of the patient with ALF depends on the type of lung injury and the underlying cause of hypoxemia. For those patients with V/Q mismatching, positioning is used to facilitate better matching of ventilation with perfusion to optimize gas exchange.26 Because gravity normally facilitates preferential ventilation and perfusion to the dependent areas of the lungs, the best gas exchange would take place in the dependent areas of the lungs.11 Thus the goal of positioning is to place the least affected area of the patient’s lung in the most dependent position. Patients with unilateral lung disease should be positioned with the healthy lung in a dependent position.26,27 Patients with diffuse lung disease may benefit from being positioned with the right lung down, because it is larger and more vascular than the left lung.27,28 For those patients with alveolar hypoventilation, the goal of positioning is to facilitate ventilation. These patients benefit from nonrecumbent positions such as sitting or a semierect position.29 In addition, semirecumbency has been shown to decrease the risk of aspiration and inhibit the development of hospital-associated pneumonia.30 Frequent repositioning (at least every 2 hours) is beneficial in optimizing the patient’s ventilatory pattern and V/Q matching.31
Preventing Desaturation.
A number of activities can prevent desaturation from occurring. These include performing procedures only as needed, hyperoxygenating the patient before suctioning, providing adequate rest and recovery time between various procedures, and minimizing oxygen consumption. Interventions to minimize oxygen consumption include limiting the patient’s physical activity, administering sedation to control anxiety, and providing measures to control fever.29 The patient should be continuously monitored with a pulse oximeter to warn of signs of desaturation.
Promoting Secretion Clearance.
Interventions to promote secretion clearance include providing adequate systemic hydration, humidifying supplemental oxygen, coughing, and suctioning. Postural drainage and chest percussion and vibration have been found to be of little benefit in the critically ill patient32,33 and thus are not discussed here.
To facilitate deep breathing, the patient’s thorax should be maintained in alignment and the head of the bed elevated 30 to 45 degrees. This position best accommodates diaphragmatic descent and intercostal muscle action.
Once the patient is extubated, deep breathing and incentive spirometry should be started as soon as possible. Deep breathing involves having the patient take a deep breath and holding it for approximately 3 seconds or longer. Incentive spirometry involves having the patient take at least 10 deep, effective breaths per hour using an incentive spirometer. These actions help prevent atelectasis and re-expand any collapsed lung tissue. The chest should be auscultated during inflation to ensure that all dependent parts of the lung are well ventilated and to help the patient understand the depth of breath necessary for optimal effect. Coughing should be avoided unless secretions are present because it promotes collapse of the smaller airways.
Educating the Patient and Family
Early in the patient’s hospital stay, the patient and family should be taught about ALF, its etiologies, and its treatment. As the patient moves toward discharge, teaching should focus on the interventions necessary for preventing the reoccurrence of the precipitating disorder (Box 20-2). If the patient smokes, he or she should be encouraged to stop smoking and be referred to a smoking cessation program (Box 20-3). In addition, the importance of participating in a pulmonary rehabilitation program should be stressed.
Collaborative management of the patient with ALF is outlined in Box 20-4.
Acute Respiratory Distress Syndrome
Description
Acute respiratory distress syndrome (ARDS) is a systemic process that is considered to be the pulmonary manifestation of multiple organ dysfunction syndrome.34 It is characterized by noncardiac pulmonary edema and disruption of the alveolar-capillary membrane as a result of injury to either the pulmonary vasculature or the airways.35
Many different diagnostic criteria have been used to identify ARDS, which has led to confusion, particularly among researchers. In 2012, in an attempt to address the limitations of the existing definition of ARDS, the ARDS Definition Task Force drafted a new definition (known as the Berlin Definition) of ARDS. This definition eliminated the term “acute lung injury” and proposed three distinct categories (mild, moderate, and severe) of ARDS based on the severity of hypoxemia. The Berlin Definition of ARDS is as follows:
• Timing—within 1 week of known clinical insult or new or worsening respiratory symptoms
• Chest imaging—bilateral opacities not fully explained by effusions, lobar/lung collapse or nodules
• Origin of edema—respiratory failure not fully explained by heart failure or fluid overload; need objective assessment to exclude hydrostatic edema if no risk factor present
• Oxygenation—mild (200 mg Hg less than Pao2/Fio2 less than or equal to 300 mm Hg with positive end-respiratory airway pressure [PEEP] or constant positive airway pressure [CPAP] greater than or equal to 5 cm H2O); Moderate (100 mg Hg less than Pao2/Fio2 less than or equal to 200 mm Hg with PEEP greater than or equal to 5 cm H2O); or Severe (Pao2/Fio2 less than or equal to 100 mm Hg with PEEP greater than or equal to 5 cm H2O).36
The mortality rate for ARDS is estimated to be 34% to 58%.37
Etiology
A wide variety of clinical conditions is associated with the development of ARDS. These are categorized as direct or indirect, depending on the primary site of injury (Box 20-5).35,38 Direct injuries are those in which the lung epithelium sustains a direct insult. Indirect injuries are those in which the insult occurs elsewhere in the body and mediators are transmitted via the bloodstream to the lungs. Sepsis, aspiration of gastric contents, diffuse pneumonia, and trauma were found to be major risk factors for the development of ARDS.37
Pathophysiology
The progression of ARDS can be described in three phases: exudative, fibroproliferative, and resolution. ARDS is initiated with stimulation of the inflammatory-immune system as a result of a direct or indirect injury (Fig. 20-3). Inflammatory mediators are released from the site of injury, resulting in the activation and accumulation of the neutrophils, macrophages, and platelets in the pulmonary capillaries. These cellular mediators initiate the release of humoral mediators that cause damage to the alveolar-capillary membrane.38
Exudative Phase
Within the first 72 hours after the initial insult, the exudative phase or acute phase ensues. Once released, the mediators cause injury to the pulmonary capillaries, resulting in increased capillary membrane permeability leading to the leakage of fluid filled with protein, blood cells, fibrin, and activated cellular and humoral mediators into the pulmonary interstitium. Damage to the pulmonary capillaries also causes the development of microthrombi and elevation of pulmonary artery pressures. As fluid enters the pulmonary interstitium, the lymphatics are overwhelmed and unable to drain all the accumulating fluid, resulting in the development of interstitial edema. Fluid is then forced from the interstitial space into the alveoli, resulting in alveolar edema. Pulmonary interstitial edema also causes compression of the alveoli and small airways. Alveolar edema causes swelling of the type I alveolar epithelial cells and flooding of the alveoli. Protein and fibrin in the edema fluid precipitate the formation of hyaline membranes over the alveoli. Eventually, the type II alveolar epithelial cells are also damaged, leading to impaired surfactant production. Injury to the alveolar epithelial cells and the loss of surfactant lead to further alveolar collapse.38,39
Hypoxemia occurs as a result of intrapulmonary shunting and V/Q mismatching secondary to compression, collapse, and flooding of the alveoli and small airways. Increased work of breathing occurs as a result of increased airway resistance, decreased functional residual capacity (FRC), and decreased lung compliance secondary to atelectasis and compression of the small airways. Hypoxemia and the increased work of breathing lead to patient fatigue and the development of alveolar hypoventilation. Pulmonary hypertension occurs as a result of damage to the pulmonary capillaries, microthrombi, and hypoxic vasoconstriction leading to the development of increased alveolar dead space and right ventricular afterload. Hypoxemia worsens as a result of alveolar hypoventilation and increased alveolar dead space. Right ventricular afterload increases and leads to right ventricular dysfunction and a decrease in cardiac output (CO).38
Fibroproliferative Phase
This phase begins as disordered healing and starts in the lungs. Cellular granulation and collagen deposition occur within the alveolar-capillary membrane. The alveoli become enlarged and irregularly shaped (fibrotic) and the pulmonary capillaries become scarred and obliterated. This leads to further stiffening of the lungs, increasing pulmonary hypertension, and continued hypoxemia.38,39
Resolution Phase
Recovery occurs over several weeks as structural and vascular remodeling take place to re-establish the alveolar-capillary membrane. The hyaline membranes are cleared and intra-alveolar fluid is transported out of the alveolus into the interstitium. The type II alveolar epithelial cells multiply, some of which differentiate to type I alveolar epithelial cells, facilitating the restoration of the alveolus. Alveolar macrophages remove cellular debris.38,39
Assessment and Diagnosis
Initially the patient with ARDS maybe seen with a variety of clinical manifestations, depending on the precipitating event. As the disorder progresses, the patient’s signs and symptoms can be associated with the phase of ARDS that he or she is experiencing (Table 20-2). During the exudative phase, the patient presents with tachypnea, restlessness, apprehension, and moderate increase in accessory muscle use. During the fibroproliferative phase, the patient’s signs and symptoms progress to agitation, dyspnea, fatigue, excessive accessory muscle use, and fine crackles as respiratory failure develops.40,41
TABLE 20-2
PHYSIOLOGY AND ASSOCIATED PHYSICAL EXAMINATION OF PATIENT WITH ARDS
PHASE | PHYSIOLOGY | PHYSICAL EXAMINATION |
Exudative Phase | ||
Parenchymal surface hemorrhage | Restless, apprehensive, tachypneic | |
Interstitial or alveolar edema | Respiratory alkalosis | |
Compression of terminal bronchioles | Pao2 normal | |
Destruction of type I alveolar cells | CXR: normal | |
Chest examination: moderate use of accessory muscles, lungs clear | ||
Pulmonary artery pressures: elevated | ||
Pulmonary artery occlusion pressure: normal or low | ||
Fibroproliferative Phase | ||
Destruction of type II alveolar cells | Pulmonary artery pressures: elevated | |
Gas exchange compromised | Increased workload on right ventricle | |
Increased peak inspiratory pressure | Increased use of accessory muscles | |
Decreased compliance (static and dynamic) | Fine crackles | |
Increasing agitation related to hypoxia | ||
Refractory hypoxemia: | CXR: interstitial or alveolar infiltrates; elevated diaphragm | |
Modified from Phillips JK. Management of patients with acute respiratory distress syndrome. Crit Care Nurs Clin North Am.1999;11(2):233.
Arterial blood-gas analysis reveals a low Pao2, despite increases in supplemental oxygen administration (refractory hypoxemia).40 Initially the Paco2 is low as a result of hyperventilation, but eventually the Paco2 increases as the patient fatigues. The pH is high initially but decreases as respiratory acidosis develops.40,41
Initially the chest x-ray film may be normal, because changes in the lungs do not become evident for up to 24 hours. As the pulmonary edema becomes apparent, diffuse, patchy interstitial and alveolar infiltrates appear. This progresses to multifocal consolidation of the lungs, which appears as a “whiteout” on the chest x-ray film.40
Medical Management
Medical management of the patient with ARDS involves a multifaceted approach. This strategy includes treating the underlying cause, promoting gas exchange, supporting tissue oxygenation, and preventing complications. Given the severity of hypoxemia, the patient is intubated and mechanically ventilated to facilitate adequate gas exchange.42
Ventilation
Traditionally the patient with ARDS was ventilated with a mode of volume ventilation, such as assist/control ventilation (A/CV) or synchronized intermittent mandatory ventilation (SIMV), with tidal volumes adjusted to deliver 10 to 15 mL/kg. Current research now indicates that this approach may have actually led to further lung injury. It is now known that repeated opening and closing of the alveoli cause injury to the lung units (atelectrauma), resulting in inhibited surfactant production, and increased inflammation (biotrauma), resulting in the release of mediators and an increase in pulmonary capillary membrane permeability. In addition, excessive pressure in the alveoli (barotrauma) or excessive volume in the alveoli (volutrauma) leads to excessive alveolar wall stress and damage to the alveolar-capillary membrane, resulting in air escaping into the surrounding spaces.42 Thus several different approaches have been developed to facilitate the mechanical ventilation of the patient with ARDS.
Low Tidal Volume.
Low tidal volume ventilation uses smaller tidal volumes (6 mL/kg) to ventilate the patient, in an attempt to limit the effects of barotrauma and volutrauma. The goal is to provide the maximum tidal volume possible while maintaining end-inspiratory plateau pressure less than 30 cm H2O. To allow for adequate carbon dioxide elimination, the respiratory rate is increased to 20 to 30 breaths/min.42,43
Permissive Hypercapnia.
Permissive hypercapnia uses low tidal volume ventilation in conjunction with normal respiratory rates, in an attempt to limit the effects of atelectrauma and biotrauma. Normally, to maintain normocapnia the patient’s respiratory rate would have to be increased to compensate for the small tidal volume. In ARDS though, increasing the respiratory rate can lead to worsening alveolar damage. Thus the patient’s carbon dioxide level is allowed to rise, and the patient becomes hypercapnic. As a general rule, the patient’s Paco2 should not rise faster than 10 mm Hg per hour and overall should not exceed 80 to 100 mg Hg. Because of the negative cardiopulmonary effects of severe acidosis, the arterial pH is generally maintained at 7.20 or greater. To maintain the pH, the patient is given intravenous sodium bicarbonate or the respiratory rate and/or tidal volume are increased. Permissive hypercapnia is contraindicated in patients with increased intracranial pressure, pulmonary hypertension, seizures, and heart failure.44
Pressure Control Ventilation.
In pressure control ventilation (PCV) mode, each breath is delivered or augmented with a preset amount of inspiratory pressure as opposed to tidal volume, which is used in volume ventilation. Thus the actual tidal volume the patient receives varies from breath to breath. PCV is used to limit and control the amount of pressure in the lungs and decrease the incidence of volutrauma. The goal is to keep the patient’s plateau pressure (end-inspiratory static pressure) lower than 30 cm H2O. A known problem with this mode of ventilation is that as the patient’s lungs get stiffer, it becomes harder and harder to maintain an adequate tidal volume and severe hypercapnia can occur.42,43
Inverse Ratio Ventilation.
Another alternative ventilatory mode that is used in managing the patient with ARDS is inverse ratio ventilation (IRV), either pressure controlled or volume controlled. IRV prolongs the inspiratory (I) time and shortens the expiratory (E) time, thus reversing the normal I : E ratio. The goal of IRV is to maintain a more constant mean airway pressure throughout the ventilatory cycle, which helps keep alveoli open and participating in gas exchange. It also increases FRC and decreases the work of breathing. In addition, as the breath is delivered over a longer period of time, the peak inspiratory pressure in the lungs is decreased. A major disadvantage to IRV is the development of auto–PEEP. As the expiratory phase of ventilation is shortened, air can become trapped in the lower airways, creating unintentional PEEP (or auto-PEEP), which can cause hemodynamic compromise and worsening gas exchange. Patients on IRV usually require heavy sedation with neuromuscular blockade to prevent them from fighting the ventilator.42,43
High-Frequency Oscillatory Ventilation.
Another alternative ventilatory mode that is used in patients who remain severely hypoxemic despite the treatments previously described is high-frequency oscillatory ventilation (HFOV). The goal of this method of ventilation is similar to that of IRV in that it uses a constant airway pressure to promote alveolar recruitment while avoiding overdistention of the alveoli. HFOV uses a piston pump to deliver very low tidal volumes at very high rates or oscillations (300 to 3000 breaths/min).45
Oxygen Therapy
Oxygen is administered at the lowest level possible to support tissue oxygenation. Continued exposure to high levels of oxygen can lead to oxygen toxicity, which perpetuates the entire process. The goal of oxygen therapy is to maintain an arterial hemoglobin oxygen saturation of 90% or greater using the lowest level of oxygen—preferably less than 0.50.35
Positive End-Expiratory Pressure.
Because the hypoxemia that develops with ARDS is often refractory or unresponsive to oxygen therapy, it is necessary to facilitate oxygenation with PEEP. The purpose of using PEEP in the patient with ARDS is to improve oxygenation while reducing Fio2 to less toxic levels. PEEP has several positive effects on the lungs, including opening collapsed alveoli, stabilizing flooded alveoli, and increasing FRC. Thus PEEP decreases intrapulmonary shunting and increases compliance. PEEP also has several negative effects including: 1) decreasing CO as a result of decreasing venous return secondary to increased intrathoracic pressure; and 2) barotrauma, as a result of gas escaping into the surrounding spaces secondary to alveolar rupture. The amount of PEEP a patient requires is determined by evaluating both arterial hemoglobin oxygen saturation and CO. In most cases, a PEEP of 10 to 15 cm H2O is adequate. If PEEP is too high, it can result in overdistention of the alveoli, which can impede pulmonary capillary blood flow, decrease surfactant production, and worsen intrapulmonary shunting. If PEEP is too low, it allows the alveoli to collapse during expiration, which can result in more damage to alveoli.42
Extracorporeal and Intracorporeal Gas Exchange.
Extracorporeal and intracorporeal gas exchanges are last-resort techniques used in the treatment of severe ARDS when conventional therapy has failed. These methods allow the lungs to rest by facilitating the removal of carbon dioxide and providing oxygen external to the lungs by means of an “artificial lung,” or membrane/fiber oxygenator. Extracorporeal membrane oxygenation (ECMO), extracorporeal carbon dioxide removal (ECCO2R), and intravascular oxygenation (IVOX) are three techniques that employ this type of technology. ECMO is similar to cardiopulmonary bypass in that blood is removed from the body and pumped through a membrane oxygenator, where CO2 is removed and O2 is added, and then returned to the body. ECCO2R is a variation of ECMO in which the primary focus is removal of CO2. IVOX facilitates oxygenation and ventilation with the use of a fiber oxygenator that is implanted in the inferior vena cava. All of these techniques pose serious bleeding problems to the patient, and none has been shown to improve patient outcome.46,47
Tissue Perfusion
Adequate tissue perfusion depends on an adequate supply of oxygen being transported to the tissues. An adequate CO and hemoglobin level is critical to oxygen transport. CO depends on heart rate, preload, afterload, and contractility. A variety of fluids and medications are used to manipulate this parameter. Newer approaches to fluid management include maintaining a very low intravascular volume (pulmonary artery occlusion pressure of 5 to 8 mm Hg) with fluid restriction and diuretics, while supporting the CO with vasoactive and inotropic medications. The goal is to decrease the amount of fluid leakage into the lungs.48
Nursing Management
Nursing management of the patient with ARDS incorporates a variety of nursing diagnoses (Box 20-6). Nursing interventions include optimizing oxygenation and ventilation, providing comfort and emotional support, and maintaining surveillance for complications.
Optimizing Oxygenation and Ventilation
Nursing interventions to optimize oxygenation and ventilation include positioning, preventing desaturation, and promoting secretion clearance. For further discussion on these interventions, see Nursing Management of Acute Lung Failure earlier in this chapter. One additional nursing intervention that can be used to improve the oxygenation and ventilation of the patient with ARDS is prone positioning.
Prone Positioning.
A number of studies have shown that prone positioning the patient with ARDS results in an improvement in oxygenation. Although a number of theories propose how prone positioning improves oxygenation, the discovery that with ARDS there is greater damage to the dependent areas of the lungs probably provides the best explanation. It was originally thought that ARDS was a diffuse homogenous disease that affected all areas of the lungs equally. It is now known that the dependent lung areas are more heavily damaged than the nondependent lung areas. Turning the patient prone improves perfusion to less damaged parts of lungs and improves V/Q matching and decreases intrapulmonary shunting. Prone positioning appears to be more effective when initiated during the early phases of ARDS.49 For more information on prone positioning see Chapter 21.
Collaborative management of the patient with ARDS is outlined in Box 20-7.
Pneumonia
Description
Pneumonia is an acute inflammation of the lung parenchyma that is caused by an infectious agent that can lead to alveolar consolidation. Pneumonia can be classified as community-acquired pneumonia (CAP), hospital-acquired pneumonia (HAP), or health care-associated pneumonia (HCAP).50 Pneumonia is referred to as community-acquired when it occurs outside of the hospital or within 48 hours of admission to the hospital.51 Severe CAP requires admission to the critical care unit and accounts for about 22% of all patients with pneumonia. The mortality for this patient group is approximately 18% to 56%, with increasing age as a major risk factor.52 Pneumonia is referred to as hospital-acquired when it occurs while in the hospital for at least 48 hours.50 Ventilator-associated pneumonia (VAP) is a subgrouping of HAP that refers to development of pneumonia after the insertion of an artificial airway.50 VAP is the most common critical care unit-acquired infection.53 Pneumonia is referred to as health care-associated when it is acquired in health care environments outside of the traditional hospital setting.54
Etiology
The spectra of etiologic pathogens of pneumonia vary with the type of pneumonia, as do the risk factors for the disease.
Severe Community-Acquired Pneumonia
Pathogens that can cause severe CAP include Streptococcus pneumoniae, Legionella species, Haemophilus influenzae, Moraxella catarrhalis, Staphylococcus aureus, Mycoplasma pneumoniae, respiratory viruses, Chlamydia pneumoniae, and Pseudomonas aeruginosa.51 A number of factors increase the risk for developing CAP, including alcoholism, chronic obstructive pulmonary disease (COPD), and comorbid conditions such as diabetes, malignancy, and coronary artery disease. Impaired swallowing and altered mental status also contribute to the development of CAP, because they result in an increased exposure to the various pathogens due to chronic aspiration of oropharyngeal secretions.51
Hospital-Acquired Pneumonia
Pathogens that can cause HAP include Escherichia coli, H. influenzae, methicillin-sensitive S. aureus, S. pneumoniae, P. aeruginosa, Acinetobacter baumannii, methicillin-resistant S. aureus (MRSA), Klebsiella spp., and Enterobacter spp. Two of the pathogens most frequently associated with VAP are S. aureus and P. aeruginosa. Risk factors for HAP can be categorized as host-related, treatment-related, and infection control-related (Box 20-8).55
Health Care-Associated Pneumonia
Pathogens that can cause HCAP are similar to those causing both CAP and HAP with P. aeruginosa and MRSA being the most common in the United States.54 Risk factors for HCAP include prior hospitalization (hospitalized for 2 or more days within the last 90 days), receiving hemodialysis, receiving intravenous antibiotic therapy, chemotherapy or wound care within 30 days, residing in a long-term care facility or with family member with multidrug-resistant infection, and an immunocompromised state. These patients are at a higher risk of developing multidrug-resistant organisms.56
Pathophysiology
Development of acute pneumonia implies a defect in host defenses, a particularly virulent organism, or an overwhelming inoculation event. Bacterial invasion of the lower respiratory tract can occur by inhalation of aerosolized infectious particles, aspiration of organisms colonizing the oropharynx, migration of organisms from adjacent sites of colonization, direct inoculation of organisms into the lower airway, spread of infection to the lungs from adjacent structures, spread of infection to the lung through the blood, and reactivation of latent infection (usually in the setting of immunosuppression). The most common mechanism appears to be aspiration of oropharyngeal organisms.57 Table 20-3 lists the precipitating conditions that can facilitate the development of pneumonia.
TABLE 20-3
PRECIPITATING CONDITIONS OF PNEUMONIA
CONDITION | ETIOLOGIES |
Depressed epiglottal and cough reflexes | Unconsciousness, neurologic disease, endotracheal or tracheal tubes, anesthesia, aging |
Decreased cilia activity | Smoke inhalation, smoking history, oxygen toxicity, hypoventilation, intubation, viral infections, aging, COPD |
Increased secretion | COPD, viral infections, bronchiectasis, general anesthesia, endotracheal intubation, smoking |
Atelectasis | Trauma, foreign body obstruction, tumor, splinting, shallow ventilations, general anesthesia |
Decreased lymphatic flow | Heart failure, tumor |
Fluid in alveoli | Heart failure, aspiration, trauma |
Abnormal phagocytosis and humoral activity | Neutropenia, immunocompetent disorders, patients receiving chemotherapy |
Impaired alveolar macrophages | Hypoxemia, metabolic acidosis, cigarette smoking history, hypoxia, alcohol use, viral infections, aging |
Figure 20-4 depicts the pathophysiology of HAP. Colonization of the patient’s oropharynx with infectious organisms is a major contributor to the development of HAP. Normally the oropharynx has a stable population of resident flora that may be anaerobic or aerobic. When stress occurs, such as with illness, surgery, or infection, pathogenic organisms replace normal resident flora. Previous antibiotic therapy also affects the resident flora population, making replacement by pathologic organisms more likely. The pathogens are then able to invade the sterile lower respiratory tract.55
Disruption of the gag and cough reflexes, altered consciousness, abnormal swallowing, and artificial airways all predispose the patient to aspiration and colonization of the lungs and subsequent infection. Histamine2 agonists, antacids, and enteral feedings also contribute to this problem because they raise the pH of the stomach and promote bacterial overgrowth. The nasogastric tube then acts as a wick, facilitating the movement of bacteria from the stomach to the pharynx, where the bacteria can be aspirated.55
Infection results in pulmonary inflammation with or without significant exudates. Increased capillary permeability occurs, leading to increased interstitial and alveolar fluid. V/Q mismatching and intrapulmonary shunting occurs, resulting in hypoxemia as lung consolidation progresses. Untreated pneumonia can result in ALF and initiation of the inflammatory-immune response. In addition, the patient may develop a pleural effusion. This is the result of the vascular response to inflammation, whereby capillary permeability is increased and fluid from the pulmonary capillaries diffuses into the pleural space.58
Prevention of VAP is discussed under the mechanical ventilation section of Chapter 21.
Assessment and Diagnosis
The clinical manifestations of pneumonia will vary with the offending pathogen. The patient may first be seen with a variety of signs and symptoms including dyspnea, fever, and cough (productive or nonproductive). Coarse crackles on auscultation and dullness to percussion may also be present.53 Patients with severe CAP may manifest confusion and disorientation, tachypnea, hypoxemia, uremia, leukopenia, thrombocytopenia, hypothermia, and hypotension.57
Chest radiography is used to evaluate the patient with suspected pneumonia. The diagnosis is established by the presence of a new pulmonary infiltrate. The radiographic pattern of the infiltrates will vary with the organism.51 A sputum Gram stain and culture are done to facilitate the identification of the infectious pathogen. In 50% of cases, though, a causative agent is not identified.51 A diagnostic bronchoscopy may be needed, particularly if the diagnosis is unclear or current therapy is not working.55 In addition, a complete blood count with differential, chemistry panel, blood cultures, and ABGs is obtained.51
Medical Management
Medical management of the patient with pneumonia should include antibiotic therapy, oxygen therapy for hypoxemia, mechanical ventilation if ALF develops, fluid management for hydration, nutritional support, and treatment of associated medical problems and complications. For patients having difficulty mobilizing secretions, a therapeutic bronchoscopy may be necessary.58
Antibiotic Therapy
Although bacteria-specific antibiotic therapy is the goal, this may not always be possible because of difficulties in identifying the organism and the seriousness of the patient’s condition. The time involved obtaining cultures should be balanced against the need to begin some treatment based on the patient’s condition. Empiric therapy has become a generally acceptable approach. In this approach, choice of antibiotic treatment is based on the most likely etiologic organism while avoiding toxicity, superinfection, and unnecessary cost. If available, Gram stain results should be used to guide choices of antibiotics. Antibiotics should be chosen that offer broad coverage of the usual pathogens in the hospital or community. Failure to respond to such therapy may indicate that the chosen antibiotic regimen does not appropriately cover all of the etiologic pathogens or that a new source of infection has developed.51,55
Currently the Centers for Medicare and Medicaid Services (CMS) and The Joint Commission (TJC) standards for managing patients with CAP require that the first dose of antibiotics be administered within 6 hours of arrival to the hospital. This timeframe is very controversial and has been the subject of much debate. Those in favor of the standard believe that early antibiotic administration leads to improved outcomes while those not in favor of the standard believe it leads to the overuse of antibiotics. More research is needed to clarify the issue.59
Independent Lung Ventilation
In patients with unilateral pneumonia or severely asymmetric pneumonia, this alternative mode of mechanical ventilation may be necessary to facilitate oxygenation. As the alveoli in the affected lung become flooded with pus, the lung becomes less compliant and difficult to ventilate. This results in a shifting of ventilation to the good lung without a concomitant shift in perfusion and thus an increase in V/Q mismatching. Independent lung ventilation (ILV) allows each lung to be ventilated separately, thus controlling the amount of flow, volume, and pressure each lung receives. A double-lumen endotracheal tube is inserted, and each lumen is usually attached to a separate mechanical ventilator. The ventilator settings are then customized to the needs of each lung to facilitate optimal oxygenation and ventilation.60
Nursing Management
Nursing management of the patient with pneumonia incorporates a variety of nursing diagnoses (Box 20-9). Nursing interventions include optimizing oxygenation and ventilation, preventing the spread of infection, providing comfort and emotional support, and maintaining surveillance for complications. The patient’s response to the antibiotic therapy should be monitored for adverse effects.
Preventing the Spread of Infection
Prevention should be directed at eradicating pathogens from the environment and interrupting the spread of organisms from person to person. Significant progress has been made in removing contaminants from the patient environment through proper disinfection of respiratory equipment and increased use of disposable supplies. Other possible environmental sources of pathogens include suctioning equipment and indwelling lines. These invasive tools must be given proper aseptic care.55
Proper hand hygiene is the single most important measure available to prevent the spread of bacteria from person to person (Box 20-10). Hand hygiene should occur before and after touching a patient or their surroundings, before performing a procedure, and after exposure to any body fluids.61 In addition, meticulous oral care, including suctioning of the secretions pooling above the cuff of the artificial airway, is critical to decreasing the bacterial colonization of the oropharynx.55 Oral care is further discussed in Chapter 21.
Collaborative management of the patient with pneumonia is outlined in Box 20-11.
Aspiration Pneumonitis
Description
The presence of abnormal substances in the airways and alveoli as a result of aspiration is misleadingly called aspiration pneumonia. This term is misleading because the aspiration of toxic substances into the lung may or may not involve an infection. Aspiration pneumonitis is a more accurate title, because injury to the lung can result from the chemical, mechanical, and/or bacterial characteristics of the aspirate.62
Etiology
A number of factors have been identified that place the patient at risk for aspiration (Table 20-4). Gastric contents and oropharyngeal bacteria (see Pneumonia earlier in this chapter) are the most common aspirates of the critically ill patient.62–64 The effects of gastric contents on the lungs will vary based on the pH of the liquid. If the pH is less than 2.5, the patient will develop a severe chemical pneumonitis resulting in hypoxemia. If the pH is greater than 2.5, the immediate damage to the lungs will be lessened but the elevated pH may have promoted bacterial overgrowth of the stomach.62,63 Once the bacteria-laden gastric contents are aspirated into the lungs, overwhelming bacterial pneumonia can develop.63
TABLE 20-4
RISK FACTORS FOR ASPIRATION/ASPIRATION-RELATED PNEUMONIA
RISK FACTOR | RATIONALE |
Decreased LOC, either because of CNS problems or use of sedatives | Decreased ability to protect airway from oropharyngeal secretions and regurgitated gastric contents |
Cough and gag reflexes diminish as LOC diminishes, whether from CNS disorder or sedation | |
Slowed gastric emptying | |
Decreased tone of lower esophageal sphincter | |
Supine position | Increases probability of gastroesophageal reflux |
Presence of a nasogastric tube | Interferes with closure of lower esophageal sphincter |
Biofilm on tube predisposes to aspiration of pathogenic organisms | |
Vomiting | Sudden and forceful entry of gastric contents into oropharynx predisposes to aspiration |
Predisposes to displacement of feeding tube ports into esophagus | |
Feeding tube ports positioned in esophagus | Infused feedings reflux into oropharynx |
Tracheal intubation | Reduction in upper airway defense related to ineffective cough, desensitization of the oropharynx and larynx, disuse atrophy of laryngeal muscles, and esophageal compression by an inflated cuff |
Mechanical ventilation | Positive abdominal pressure predisposes to aspiration of gastric contents, probably by increasing gastroesophageal reflux |
Accumulation of subglottic secretions above endotracheal cuff | Subglottic secretions can leak around cuff into the lower respiratory tract, especially when cuff is deflated |
Inadequate cuff inflation of tracheal devices | Persistent low cuff pressure (e.g., 20 cm H2O) predisposes to aspiration of oropharyngeal secretions and refluxed gastric contents |
Gastric feeding site when gastric emptying significantly impaired | Accumulation of formula and gastrointestinal secretions predisposes to gastroesophageal reflux and aspiration |
High GRVs | High GRVs predispose to gastroesophageal reflux and aspiration |
Bolus feedings | Volume of infused formula may exceed the tolerance of patients who have poor cough and gag reflexes |
Poor oral health | Colonized oropharyngeal secretions may be aspirated into respiratory tract |
Advanced age | Older patients tend to have a reduced swallowing ability and are more likely to have neurologic disorders that increase aspiration risks |
Strong association between advanced age and probability of developing pneumonia once aspiration has occurred | |
Hyperglycemia | Even mild hyperglycemia can cause delayed gastric emptying by disrupting postprandial antral contractions |
CNS, central nervous system; GRVs, gastric residual volumes; LOC, level of consciousness.
From Metheny NA. Strategies to prevent aspiration-related pneumonia in tube-fed patients. Respir Care Clin N Am. 2006;12:603.
Pathophysiology
The type of lung injury that develops after aspiration is determined by a number of factors, including the quality of the aspirate and the status of the patient’s respiratory defense mechanisms.
Acid Liquid
The aspiration of acid (pH less than 2.5) liquid gastric contents results in the development of bronchospasm and atelectasis almost immediately. Over the next 4 hours, tracheal damage, bronchitis, bronchiolitis, alveolar-capillary breakdown, interstitial edema, and alveolar congestion and hemorrhage occur.64 Severe hypoxemia develops as a result of intrapulmonary shunting and V/Q mismatching. As the disorder progresses, necrotic debris and fibrin fill the alveoli, hyaline membranes form, and hypoxic vasoconstriction occurs, resulting in elevated pulmonary artery pressures.63,64 The clinical course will follow one of three patterns: 1) rapid improvement in 1 week; 2) initial improvement followed by deterioration and development of ARDS or pneumonia; or 3) rapid death from progressive ALF.64
Nonacid Liquid
The aspiration of nonacid (pH greater than 2.5) liquid gastric contents is similar to acid liquid aspiration initially, but minimal structural damage occurs. Intrapulmonary shunting and V/Q mismatching usually start to reverse within 4 hours, and hypoxemia clears within 24 hours.63,64
Nonacid Food Particles
The aspiration of nonacid (pH greater than 2.5) nonobstructing food particles is similar to acid aspiration initially, with significant edema and hemorrhage occurring within 6 hours. After the initial reaction, the response changes to a foreign-body-type reaction with granuloma formation occurring around the food particles within 1 to 5 days.64 In addition to hypoxemia, hypercapnia and acidosis occur as a result of hypoventilation.62,63
Assessment and Diagnosis
Clinically, the patient presents with signs of acute respiratory distress, and gastric contents may be present in the oropharynx. The patient will have shortness of breath, coughing, wheezing, cyanosis, and signs of hypoxemia. Tachypnea, tachycardia, hypotension, fever, and crackles also are present. Copious amounts of sputum are produced as alveolar edema develops.62,63
ABGs reflect severe hypoxemia. Chest x-ray film changes appear 12 to 24 hours after the initial aspiration, with no one pattern being diagnostic of the event. Infiltrates will appear in a variety of distribution patterns depending on the position of the patient during aspiration and the volume of the aspirate. If bacterial infection becomes established, leukocytosis and positive sputum cultures occur.63
Medical Management
Management of the patient with aspiration lung disorder includes both emergency and follow-up treatment. When aspiration is witnessed, emergency treatment should be instituted to secure the airway and minimize pulmonary damage. The patient’s head should be turned to the side and the oral cavity and upper airway should be suctioned immediately to remove the gastric contents.63,64 Direct visualization by bronchoscopy is indicated to remove large particulate aspirate or to confirm an unwitnessed aspiration event. Bronchoalveolar lavage is not recommended because this practice disseminates the aspirate in lungs and increases damage.64
After airway clearance, attention should be given to supporting oxygenation and hemodynamics. Hypoxemia should be corrected with supplemental oxygen or mechanical ventilation with PEEP, if necessary.63,64 Hemodynamic changes result from fluid shifts into the lungs that can occur after massive aspirations. Monitoring intravascular volume is essential, and judicious amounts of replacement fluids should be instituted to maintain adequate urinary output and vital signs.63,64
Empiric antibiotic therapy is usually not indicated following aspiration of gastric contents. However, if VAP is suspected or the aspiration event occurred in the presence of a small bowel obstruction or colonized gastric contents, then antibiotic therapy should be considered.64 Corticosteroids have not demonstrated to be of any benefit in the treatment of aspiration pneumonitis and thus are not recommended.63,64
Nursing Management
Nursing management of the patient with aspiration lung disorder incorporates a variety of nursing diagnoses (Box 20-12). Nursing interventions include optimizing oxygenation and ventilation, preventing further aspiration events, providing comfort and emotional support, and maintaining surveillance for complications.
Preventing Aspiration
One of the most important interventions for preventing aspiration is identifying the patient at risk for aspiration (Box 20-13). Actions to prevent aspiration include confirming feeding tube placement, checking for signs and symptoms of feeding intolerance, elevating the head of the bed at least 30 to 45 degrees, feeding the patient via a small-bore feeding tube or gastrostomy tube, avoiding the use of a large-bore nasogastric tube, ensuring proper inflation of artificial airway cuffs, and frequent suctioning of the oropharynx of an intubated patient to prevent secretions from pooling above the cuff of the tube. For patients at risk for aspiration or intolerant of gastric feedings, the feeding tube should be placed in the small bowel.65
Collaborative management of the patient with aspiration pneumonitis is outlined in Box 20-14.
Pulmonary Embolism
Description
A pulmonary embolism (PE) occurs when a clot (thrombotic embolus) or other matter (nonthrombotic embolus) lodges in the pulmonary arterial system, disrupting the blood flow to a region of the lungs (Fig. 20-5). The majority of thrombotic emboli arise from the deep leg veins, particularly the iliac, femoral, and popliteal veins.66 Other sources include the right ventricle, the upper extremities, and the pelvic veins. Nonthrombotic emboli arise from fat, tumors, amniotic fluid, air, and foreign bodies. This section of the chapter focuses on thrombotic emboli.
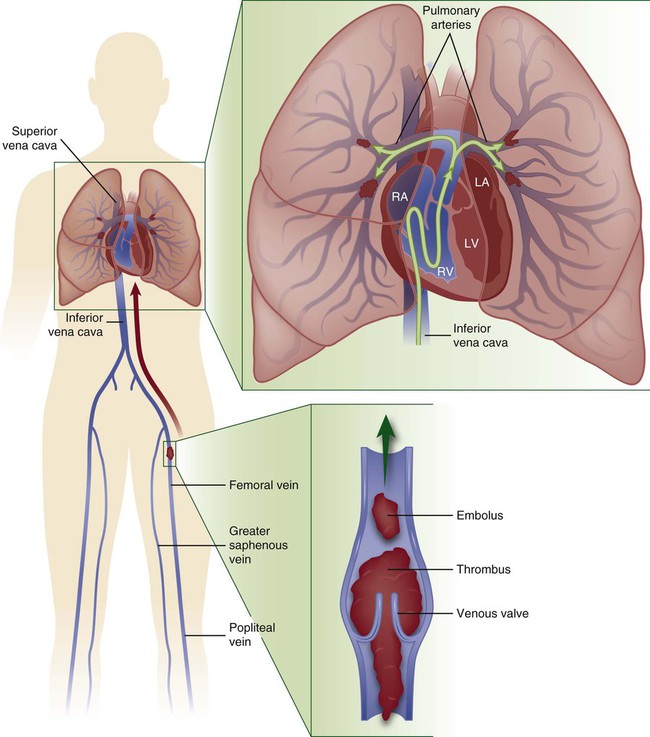
Pulmonary embolism usually originates from the deep veins of the legs, most commonly the calf veins. These venous thrombi originate predominantly in venous valve pockets and at other sites of presumed venous stasis (inset, bottom). If a clot propagates to the knee vein or above, or if it originates above the knee, the risk of embolism increases. Thromboemboli travel through the right side of the heart to reach the lungs. LA, left atrium; LV, left ventricle; RA, right atrium; RV, right ventricle. (Modified from Tapson VF. Acute pulmonary embolism. N Engl J Med. 2008;358:1037.)
Etiology
A number of predisposing factors and precipitating conditions put a patient at risk for developing a PE (Box 20-15). Of the three predisposing factors (i.e., hypercoagulability, injury to vascular endothelium, and venous stasis [Virchow’s triad]), endothelial injury appears to be the most significant.66
Pathophysiology
A massive PE occurs with the blockage of a lobar or larger artery, resulting in occlusion of more than 40% of the pulmonary vascular bed. Blockage of the pulmonary arterial system has both pulmonary and hemodynamic consequences.67 The effects on the pulmonary system are increased alveolar dead space, bronchoconstriction, and compensatory shunting.68 The hemodynamic effects include an increase in pulmonary vascular resistance and right ventricular workload.69,70
Increased Dead Space
An increase in alveolar dead space occurs because an area of the lung is receiving ventilation without being perfused. The ventilation to this area is known as wasted ventilation, because it does not participate in gas exchange. This effect leads to alveolar dead space ventilation and an increase in the work of breathing. To limit the amount of dead space ventilation, localized bronchoconstriction occurs.70
Bronchoconstriction
Bronchoconstriction develops as a result of alveolar hypocarbia, hypoxia, and the release of mediators. Alveolar hypocarbia occurs as a consequence of decreased carbon dioxide in the affected area and leads to constriction of the local airways, increased airway resistance, and redistribution of ventilation to perfused areas of the lungs. A variety of mediators are released from the site of the injury, either from the clot or the surrounding lung tissue, which further causes constriction of the airways. Bronchoconstriction promotes the development of atelectasis.70
Compensatory Shunting
Compensatory shunting occurs as a result of the unaffected areas of the lungs having to accommodate the entire CO. This creates a situation in which perfusion exceeds ventilation and blood is returned to the left side of the heart without participating in gas exchange. This leads to the development of hypoxemia.70
Hemodynamic Consequences
The major hemodynamic consequence of a PE is the development of pulmonary hypertension, which is part of the effect of a mechanical obstruction when more than 50% of the vascular bed is occluded. In addition, the mediators released at the injury site and the development of hypoxia cause pulmonary vasoconstriction, which further exacerbates pulmonary hypertension. As the pulmonary vascular resistance increases, so does the workload of the right ventricle as reflected by a rise in pulmonary artery (PA) pressures. Consequently, right ventricular failure occurs, which can lead to decreases in left ventricular preload, CO, and blood pressure, and shock.67–70
Assessment and Diagnosis
The patient with a PE may have any number of presenting signs and symptoms, with the most common being tachycardia and tachypnea. Additional signs and symptoms that may be present include dyspnea, apprehension, increased pulmonic component of the second heart sound (P1), fever, crackles, pleuritic chest pain, cough, evidence of deep vein thrombosis (DVT), and hemoptysis.67 Syncope and hemodynamic instability can occur as a result of right ventricular failure.69
Initial laboratory studies and diagnostic procedures that may be done are ABG analysis, d-dimer, electrocardiogram (ECG), chest radiography, and echocardiography (ECHO). ABGs may show a low Pao2, indicating hypoxemia; a low Paco2, indicating hypocarbia; and a high pH, indicating a respiratory alkalosis. The hypocarbia with resulting respiratory alkalosis is caused by tachypnea.68 An elevated d-dimer will occur with a PE and a number of other disorders. A normal d-dimer will not occur with a PE and thus can be used to rule out a PE as the diagnosis.67 The most frequent ECG finding seen in the patient with a PE is sinus tachycardia.66 The classic ECG pattern associated with a PE—S wave in lead I, and Q wave with inverted T wave in lead III—is seen in fewer than 20% of patients.69 Other ECG findings associated with a PE include right bundle branch block, new-onset atrial fibrillation, T-wave inversion in the anterior or inferior leads, and ST segment changes.70 Chest x-ray findings vary from normal to abnormal and are of little value in confirming the presence of a PE. Abnormal findings include cardiomegaly, pleural effusion, elevated hemidiaphragm, enlargement of the right descending pulmonary artery (Palla’s sign), a wedge-shaped density above the diaphragm (Hampton’s hump), and the presence of atelectasis.68 An ECHO, either transthoracic or transesophageal, is also useful in the identification of a PE, because it can provide visualization of any emboli in the central pulmonary arteries. In addition, it can be used for assessing the hemodynamic consequences of the PE on the right side of the heart.67
Differentiating a PE from other illnesses can be difficult because many of its clinical manifestations are found in a variety of other disorders.66 Thus a variety of other tests may be necessary, including a V/Q scintigraphy, pulmonary angiogram, and DVT studies.67,78,70 Given the advent of more sophisticated CT scanners, the spiral CT is also being used to diagnose a PE.68–70 A definitive diagnosis of a PE requires confirmation by a high-probability V/Q scan, an abnormal pulmonary angiogram or CT, or strong clinical suspicion coupled with abnormal findings on lower extremity DVT studies.68
Medical Management
Medical management of the patient with a PE involves both prevention and treatment strategies. Prevention strategies include the use of prophylactic anticoagulation with low-dose or adjusted-dose heparin, LMWH, or oral anticoagulants (Table 20-5). The use of pneumatic compression has also been demonstrated as effective methods of prophylaxis in low-risk patients.24
Unfractionated heparin 5000 units SC TID or
Dalteparin 5000 units SC QD or
Graduated compression stockings/intermittent pneumatic compression for patients with contraindications to anticoagulation
Consider combination pharmacological and mechanical prophylaxis for very high-risk patients
Consider surveillance lower extremity ultrasonography for intensive care unit patients
From Piazza G, Goldhaber SZ. Acute pulmonary embolism: part II treatment and prophylaxis. Circulation. 2006;114(3):e42.
Treatment strategies include preventing the recurrence of a PE, facilitating clot dissolution, reversing the effects of pulmonary hypertension, promoting gas exchange, and preventing complications. Medical interventions to promote gas exchange include supplemental oxygen administration, intubation, and mechanical ventilation.67
Prevention of Recurrence
Interventions to prevent the recurrence of a PE include the administration of unfractionated or LMWH and warfarin (Coumadin).69 Heparin is administered to prevent further clots from forming and has no effect on the existing clot. The heparin should be adjusted to maintain the activated partial thromboplastin time (aPTT) in the range of 2 to 3 times of upper normal.69 Warfarin should be started at the same time, and when the international normalized ratio (INR) reaches 3.0, the heparin should be discontinued. The INR should be maintained between 2.0 and 3.0. The patient should remain on warfarin for 3 to 12 months, depending on his or her risk for thromboembolic disease.69
Interruption of the inferior vena cava is reserved for patients in whom anticoagulation is contraindicated. The procedure involves placement of a percutaneous venous filter (e.g., Greenfield filter) into the vena cava, usually below the renal arteries. The filter prevents further thrombotic emboli from migrating into the lungs.71
Clot Dissolution
The administration of fibrinolytic agents in the treatment of PE has had limited success. Currently, fibrinolytic therapy is reserved for the patient with a massive PE and concomitant hemodynamic instability. Either recombinant tissue-type plasminogen activator (rt-PA) or streptokinase may be used. The therapeutic window for using fibrinolytic therapy is up to 14 days though the most benefit is usually obtained when given within 48 hours.72
If the fibrinolytic therapy is contraindicated, a pulmonary embolectomy may be performed to remove the clot. Generally it is performed as an open procedure while the patient is on cardiopulmonary bypass. An emerging alternative to surgical embolectomy is catheter embolectomy. It appears to be particularly useful if surgical embolectomy is not available or is contraindicated. It appears to be most successful when performed within 5 days of the occurrence of the PE.73
Reversal of Pulmonary Hypertension
To reverse the hemodynamic effects of pulmonary hypertension, additional measures may be taken. These include the administration of inotropic agents and fluid. Fluids should be administered to increase right ventricular preload, which would stretch the right ventricle and increase contractility, thus overcoming the elevated pulmonary arterial pressures. Inotropic agents also can be used to increase contractility to facilitate an increase in CO.69,70
Nursing Management
Prevention of PE should be a major nursing focus, because the majority of critically ill patients are at risk for this disorder. Nursing actions are aimed at preventing the development of DVT (Box 20-16), which is a major complication of immobility and a leading cause of PE. These measures include the use of pneumatic compression devices, active/passive range-of-motion exercises involving foot extension, adequate hydration, and progressive ambulation.24
Nursing management of the patient with a PE incorporates a variety of nursing diagnoses (Box 20-17). Nursing interventions include optimizing oxygenation and ventilation, monitoring for bleeding, providing comfort and emotional support, maintaining surveillance for complications, and educating the patient and family.
Monitoring for Bleeding
The patient receiving anticoagulant or fibrinolytic therapy should be observed for signs of bleeding. The patient’s gums, skin, urine, stool, and emesis should be screened for signs of overt or covert bleeding. In addition, monitoring the patient’s INR or aPTT is critical to managing the anticoagulation therapy.
Educating the Patient and Family
Early in the patient’s hospital stay, the patient and family should be taught about pulmonary embolus, its etiologies, and its treatment (Box 20-18). As the patient moves toward discharge, teaching should focus on the interventions necessary for preventing the reoccurrence of DVT and subsequent emboli, signs and symptoms of DVT and anticoagulant complications, and measures to prevent bleeding. If the patient smokes, he or she should be encouraged to stop smoking and be referred to a smoking cessation program.
Collaborative management of the patient with a pulmonary embolus is outlined Box 20-19.
Status Asthmaticus
Description
Asthma is a COPD that is characterized by partially reversible airflow obstruction, airway inflammation, and hyperresponsiveness to a variety of stimuli.74 Status asthmaticus is a severe asthma attack that fails to respond to conventional therapy with bronchodilators, which may result in ALF.75,76
Etiology
The precipitating cause of the attack is usually an upper respiratory infection, allergen exposure, or a decrease in antiinflammatory medications. Other factors that have been implicated include overreliance on bronchodilators, environmental pollutants, lack of access to health care, failure to identify worsening airflow obstruction, and noncompliance with the health care regimen.75
Pathophysiology
An asthma attack is initiated when exposure to an irritant or trigger occurs, resulting in the initiation of the inflammatory-immune response in the airways. Bronchospasm occurs along with increased vascular permeability and increased mucus production. Mucosal edema and thick, tenacious mucus further increase airway responsiveness. The combination of bronchospasm, airway inflammation, and hyperresponsiveness results in narrowing of the airways and airflow obstruction. These changes have significant effects on the pulmonary and cardiovascular systems.75
Pulmonary Effects
As the diameter of the airways decreases, airway resistance increases, resulting in increased residual volume, hyperinflation of the lungs, increased work of breathing, and abnormal distribution of ventilation. V/Q mismatching occurs, which results in hypoxemia. Alveolar dead space also increases as hypoxic vasoconstriction occurs, resulting in hypercapnia.75
Cardiovascular Effects
Inspiratory muscle force also increases in an attempt to ventilate the hyperinflated lungs. This results in a significant increase in negative intrapleural pressure, leading to an increase in venous return and pooling of blood in the right ventricle. The stretched right ventricle causes the intraventricular septum to shift, thereby impinging on the left ventricle. In addition, the left ventricle has to work harder to pump blood from the markedly negative pressure in the thorax to elevated pressure in systemic circulation. This leads to a decrease in CO and a fall in systolic blood pressure on inspiration (pulsus paradoxus).75
Assessment and Diagnosis
Initially the patient may present with a cough, wheezing, and dyspnea. As the attack continues, the patient develops tachypnea, tachycardia, diaphoresis, increased accessory muscle use, and pulsus paradoxus greater than 25 mm Hg. Decreased level of consciousness, inability to speak, significantly diminished or absent breath sounds, and inability to lie supine herald the onset of ALF.74–76
Initial ABGs indicate hypocapnia and respiratory alkalosis caused by hyperventilation. As the attack continues and the patient starts to fatigue, hypoxemia and hypercapnia develop.76 Lactic acidosis also may occur as a result of lactate overproduction by the respiratory muscles. The end result is the development of respiratory and metabolic acidosis.77
Deterioration of pulmonary function tests despite aggressive bronchodilator therapy is diagnostic of status asthmaticus and indicates the potential need for intubation. A peak expiratory flow rate (PEFR) less than 40% of predicted or forced expiratory volume in 1 second (FEV1; maximum volume of gas that the patient can exhale in 1 second) less than 20% of predicted indicates severe airflow obstruction, and the need for intubation with mechanical ventilation may be imminent.78
Medical Management
Medical management of the patient with status asthmaticus is directed toward supporting oxygenation and ventilation. Bronchodilators, corticosteroids, oxygen therapy, and intubation and mechanical ventilation are the mainstays of therapy.76
Bronchodilators
Inhaled beta2-agonists and anticholinergics are the bronchodilators of choice for status asthmaticus. Beta2-agonists promote bronchodilation and can be administered by nebulizer or metered-dose inhaler (MDI). Usually larger and more frequent doses are given, and the medication is titrated to the patient’s response. Anticholinergics that inhibit bronchoconstriction are not very effective by themselves, but in conjunction with beta2-agonists, they have a synergistic effect and produce a greater improvement in airflow. The routine use of xanthines is not recommended in the treatment of status asthmaticus because they have been shown to have no therapeutic benefit.75–78
A number of studies have focused on the bronchodilator abilities of magnesium. Although it has been demonstrated that magnesium is inferior to beta2-agonists as a bronchodilator, in patients who are refractory to conventional treatment, magnesium may be beneficial. A bolus of 1 to 4 g of intravenous magnesium given over 10 to 40 minutes has been reported to produce desirable effects.75,76,78
A number of other studies are evaluating the effects of leukotriene inhibitors such as zafirlukast, montelukast, and zileuton in the treatment of status asthmaticus. Leukotrienes are inflammatory mediators known to cause bronchoconstriction and airway inflammation. Research suggests that these agents may be beneficial as bronchodilators in those patients who are refractory to beta2-agonists.78
Systemic Corticosteroids
Intravenous or oral corticosteroids also are used in the treatment of status asthmaticus. Their anti-inflammatory effects limit mucosal edema, decrease mucus production, and potentiate beta2-agonists. It usually takes 6 to 8 hours for the effects of the corticosteroids to become evident.76 The use of inhaled corticosteroids for the treatment of status asthmaticus remains undecided at this time.75,76 Initial studies indicate they may be beneficial in certain patient populations.76
Oxygen Therapy
Initial treatment of hypoxemia is with supplemental oxygen. High-flow oxygen therapy is administered to keep the patient’s Spo2 greater than 92%.75 Another therapy currently under investigation is the use of heliox. A mixture of helium and oxygen, heliox has a lower density and higher viscosity than an oxygen and air mixture. Heliox is believed to reduce the work of breathing and improve gas exchange because it flows more easily through constricted areas. Studies have shown that it reduces air trapping and carbon dioxide and helps relieve respiratory acidosis.75
Intubation and Mechanical Ventilation
Indications for mechanical ventilation include cardiac or respiratory arrest, disorientation, failure to respond to bronchodilator therapy, and exhaustion.75,77,78 A large endotracheal tube (8 mm) should be used to decrease airway resistance and to facilitate suctioning of secretions. Ventilating the patient with status asthmaticus can be very difficult. High inflation pressures should be avoided because they can result in barotrauma. The use of PEEP should be monitored closely because the patient is prone to developing air trapping. Patient–ventilator asynchrony also can be a major problem. Sedation and neuromuscular paralysis may be necessary to allow for adequate ventilation of the patient.75,77
Nursing Management
Nursing management of the patient with status asthmaticus incorporates a variety of nursing diagnoses (Box 20-20). Nursing interventions include optimizing oxygenation and ventilation, providing comfort and emotional support, maintaining surveillance for complications, and educating the patient and family.
Educating the Patient and Family
Early in the patient’s hospital stay, the patient and family should be taught about asthma, its triggers, and its treatment (Box 20-21). As the patient moves toward discharge, teaching should focus on the interventions necessary for preventing the recurrence of status asthmaticus, early warning signs of worsening airflow obstruction, correct use of an inhaler and a peak flowmeter, measures to prevent pulmonary infections, and signs and symptoms of a pulmonary infection. If the patient smokes, he or she should be encouraged to stop smoking and be referred to a smoking cessation program. In addition, the importance of participating in a pulmonary rehabilitation program should be stressed.
Collaborative management of the patient with status asthmaticus is outlined in Box 20-22.
Air Leak Disorders
Description
Air leak disorders consist of those conditions that result in extra-alveolar air accumulation. These disorders are commonly divided into two categories: pneumothorax79,80 and barotrauma.81 A pneumothorax occurs with the accumulation of air or other gas in the pleural space.79,80 Barotrauma is the result of excessive pressure in the alveoli that can lead to extreme alveolar wall stress and damage to the alveolar-capillary membrane, causing air to escape into the surrounding spaces.81 The individual air leak disorders are described in Table 20-6.
TABLE 20-6
TYPE | DESCRIPTION |
Pneumothorax | |
Spontaneous | |
Primary | Disruption of the visceral pleura that allows air from the lung to enter the pleural space; occurs spontaneously in patients without underlying lung disease |
Secondary | Disruption of the visceral pleura that allows air from the lung to enter the pleural space; occurs spontaneously in patients with underlying lung disease |
Traumatic | |
Open | Laceration in the parietal pleura that allows atmospheric air to enter the pleural space; occurs as a result of penetrating chest trauma |
Closed | Laceration in the visceral pleura that allows air from the lung to enter the pleural space; occurs as a result of blunt chest trauma |
Iatrogenic | Laceration in the visceral pleura that allows air from the lung to enter the pleural space; occurs as a result of therapeutic or diagnostic procedures, such as central line insertion, thoracentesis, and needle aspiration |
Tension | Occurs when air is allowed to enter the pleural space but not to exit it; as pressure increases inside the pleural space, the lung collapses and the mediastinum shifts to the unaffected side; may result from a spontaneous or traumatic pneumothorax |
Barotrauma/Volutrauma | |
Pulmonary interstitial emphysema | Air in the pulmonary interstitial space |
Subcutaneous emphysema | Air in the subcutaneous tissues |
Pneumomediastinum | Air in the mediastinal space |
Pneumopericardium | Air in the pericardial space |
Pneumoperitoneum | Air in the peritoneal space |
Pneumoretroperitoneum | Air in the retroperitoneal space |
Etiology
The two main causes of air leak disorders are 1) disruption of the parietal or visceral pleura, which allows air to enter the pleural space79,80; and 2) rupture of alveoli, which allows air to enter the interstitial space.81 Disruption of the parietal pleura occurs as the result of penetrating trauma to the chest wall, which allows atmospheric air to enter the pleural space (traumatic open pneumothorax).79,80 Disruption of the visceral pleura occurs as the result of entry of air into the pleural space from the lung. This may be caused by blunt chest wall trauma (traumatic closed pneumothorax), diagnostic or therapeutic procedures (traumatic iatrogenic pneumothorax), diseases of the pulmonary system (secondary spontaneous pneumothorax), or ruptured subpleural blebs (primary spontaneous pneumothorax).79,80 Alveolar rupture occurs as the result of a change in the pressure gradient between the alveoli and the surrounding interstitial space. An increase in alveolar pressure or a decrease in interstitial pressure can lead to overdistention of the alveoli, rupture, and air leakage into the interstitial space. One of the most common causes of barotrauma is mechanical ventilation.81
Pathophysiology
The pathologic consequences of pneumothorax and those of barotrauma or volutrauma are different.
Pneumothorax
Regardless of the cause, the entry of air into the pleural space compresses the affected lung. As the lung collapses, the alveoli become underventilated, causing V/Q mismatching and intrapulmonary shunting. If the pneumothorax is large, hypoxemia ensues and ALF quickly develops. Increased pressure within the chest can lead to shifting of the mediastinum, compression of the great vessels, and decreased CO.79,80
Barotrauma
After air enters the interstitial space, it travels though the pulmonary interstitium (pulmonary interstitial emphysema), out through the hilum, and into the mediastinum (pneumomediastinum), pleural space (pneumothorax), subcutaneous tissues (subcutaneous emphysema), pericardium (pneumopericardium), peritoneum (pneumoperitoneum), and retroperitoneum (pneumoretroperitoneum).81 Except for pneumothorax, the resultant disorders are usually fairly benign. Pneumomediastinum has been associated with decreased venous return and upper airway obstruction,82 and pneumopericardium has been associated with cardiac tamponade.83
Assessment and Diagnosis
The clinical manifestations of a pneumothorax depend on the degree of lung collapse. If the pneumothorax is large, decreased respiratory excursion on the affected side may be noticed, along with bulging intercostal muscles. The trachea may deviate away from the affected side. Percussion reveals hyperresonance with decreased or absent breath sounds over the affected area. ABG analysis demonstrates hypoxemia and hypercapnia.79,80 A chest radiograph confirms the pneumothorax with increased translucency evident on the affected side (Fig. 20-6).80,84
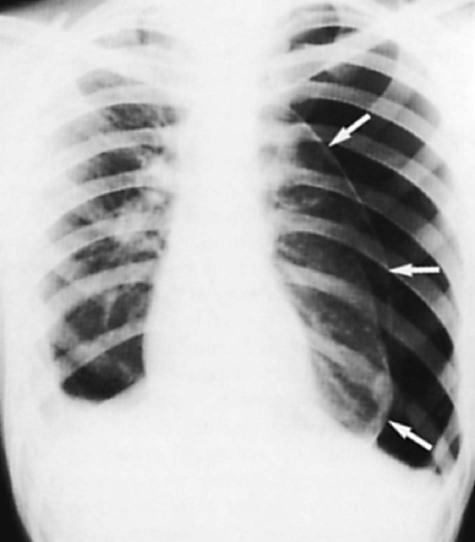
Notice the shift of the heart and mediastinum to the right. (From Des Jardin T, Burton GC. Clinical Management and Assessment of Respiratory Disease. 3rd ed. St. Louis: Mosby; 1995.)
The clinical manifestations of barotrauma are much more subtle. Subcutaneous emphysema is manifested by crepitus, usually around the face, neck, and upper chest.80 Stabbing substernal pain with position changes and with increased ventilation is the most commonly reported symptom of a pneumomediastinum.82 A clicking or crunching sound synchronous with the heart sounds may be heard over the apex of the heart (Hamman sign).82 A friction rub may be heard with a pneumopericardium.83 Barotrauma is also confirmed by radiography. Extra-alveolar air, as evidenced by increased translucency, is present in the affected area (e.g., chest, abdomen).85
Medical Management
Medical management of air leak disorders varies depending on the severity of the specific disorder. Usually, only a pneumothorax would require treatment, and that would depend on its size. A pneumothorax of less than 15% usually requires no treatment other than supplemental oxygen administration, unless complications occur or underlying lung disease or injury is present.79,80
A pneumothorax greater than 15% requires intervention to evacuate the air from the pleural space and facilitate re-expansion of the collapsed lung.80,86 Interventions include aspiration of the air with a needle and placement of a small-bore (12 to 20 Fr) or large-bore (24 to 40 Fr) chest tube. Chest tubes are inserted into the pleural space to remove fluid or air, reinstate the negative intrapleural pressure, and re-expand a collapsed lung.80,86 The trend appears to be toward placement of a small-bore tube, as the smaller tubes are more comfortable for the patient and just as effective as large-bore tubes. Patients on mechanical ventilation with a pneumothorax due to barotrauma are best managed with large-bore chest tubes.87
Chest tubes are usually inserted in the fourth or fifth intercostal space on the midaxillary line. After the tube is inserted, it is attached to a Heimlich valve or an underwater-seal drainage system. The Heimlich valve is a small one-way valve device that allows air to exit from the pleural space but not enter it (Fig. 20-7). It can be used alone or attached to a drainage bag.80
An underwater-seal drainage system is a disposable plastic unit that has three separate chambers: a water-seal chamber, a suction-control chamber, and a drainage-collection chamber (Fig. 20-8). Usually the water-seal chamber is filled to the 2-cm level and the suction-control chamber is adjusted to the desired level of suction. The water-seal chamber acts as a one-way valve, allowing air to escape from the chest but not to enter it. After the chest tubes are placed, the suction-control chamber is attached to an external suction regulator, which is adjusted until the desired level of suction is established (usually 20 cm H2O). Any fluid draining from the chest will be evident in the collection chamber. Connection points of the drainage tubing are sealed with tape, and an occlusive dressing is applied over the chest tube insertion site. After the tubes are inserted and connected to either device, a chest radiograph should be obtained to confirm re-expansion of the lung.80,88
Surgical intervention may be necessary in patients with a persistent air leak or failure of the lung to expand within 3 to 5 days. The preferred procedure is a video-assisted thoracoscopic surgery.86 Two conditions that require emergency intervention for immediate relief are a tension pneumothorax and a tension pneumopericardium.
Tension Pneumothorax
A tension pneumothorax develops when air enters the pleural space on inhalation and cannot exit on exhalation. As pressure inside the pleural space increases, it results in collapse of the lung and shifting of the mediastinum and trachea to the unaffected side (see Fig. 20-6). The resultant effect is decreased venous return and compression of the unaffected lung. Clinical signs include diminished breath sounds, hyperresonance to percussion, tachycardia, and hypotension. Treatment comprises administration of supplemental oxygen and insertion of a large-bore needle or catheter into the second intercostal space at the midclavicular line of the affected side. This action relieves the pressure within the chest. The needle should remain in place until the patient is stabilized and a chest tube is inserted.79,86
Tension Pneumopericardium
A tension pneumopericardium develops when air enters the pericardial space and has no outlet for exiting. As pressure inside the pericardium increases, it results in compression of the heart and the development of cardiac tamponade. A pericardiocentesis should be performed immediately to relieve the pressure within the pericardial sac.83
Nursing Management
Nursing management of the patient with an air leak disorder incorporates a variety of nursing diagnoses (Box 20-23). Nursing interventions should focus on optimizing oxygenation and ventilation, maintaining the chest tube system, providing comfort and emotional support, and maintaining surveillance for complications.
Maintaining the Chest Tube System
Maintaining the chest tube system involves careful attention to the suction applied and to maintenance of unobstructed drainage tubes. Kinks and large loops of tubing should be avoided, because they impede drainage and air evacuation, which may prevent timely lung re-expansion or may result in a tension pneumothorax. Retained drainage also becomes an excellent medium for bacterial growth. The water-seal chamber must routinely be observed for unexpected bubbling caused by an air leak in the system.88
If unexpected bubbling is present, the source must be identified. To determine whether the source is within the system or within the patient, systematic brief clamping of the drainage tube should be performed. The nurse should place a padded clamp on the drainage tubing as close to the occlusive dressing as possible. If the air bubbling stops, the air leak is located between the patient and the clamp. The leak can be within the patient or at the insertion site. The clamp should then be removed and the chest tube site exposed. The tube should be inspected at the site where it enters the chest to ensure that all the eyelets are within the patient. If an eyelet port is outside the chest, it can be a source of an air leak and must be occluded, which may require the attention of the physician. After the insertion site has been eliminated as a leakage source, the chest dressing should be reapplied, completely and securely covering the site. If the air bubbling does not stop when a clamp is placed on the chest tube, the leak must be located between the clamp and the drainage collector. It can be found by releasing the clamp and moving it down the tubing a few inches at a time until the bubbling stops. After the area of the leak is located, it can be taped to re-establish a seal, or the system can be replaced.88
A sterile occlusive dressing and a bottle of sterile water should be available at the patient’s bedside at all times. If the chest tube system is inadvertently interrupted, the tube should be placed a few centimeters into the bottle of water while the drainage system is re-established. A sterile occlusive dressing is applied to the chest wall if the chest tube is accidentally removed. Immediate implementation of these techniques minimizes or prevents the formation of a pneumothorax and avoids greater complications.
Throughout the duration of chest tube placement, the patient should be assessed periodically for re-expansion of the lung and for complications of chest tube drainage. The nurse should assess the thorax and lungs, paying particular attention to any tracheal deviation, asymmetry of chest movement, presence of subcutaneous emphysema, characteristics of breathing, quality of lung sounds, and presence of tympany or percussion sounds, which are indicative of pneumothorax.
Collaborative management of the patient with an air leak disorder is outlined in Box 20-24.
Long-Term Mechanical Ventilator Dependence
Description
Long-term mechanical ventilator dependence (LTMVD) is a secondary disorder that occurs when a patient requires assisted ventilation longer than expected given the patient’s underlying condition.89 It is the result of complex medical problems that do not allow the weaning process to take place in a normal and timely manner. A review of the literature reveals a great deal of confusion regarding an exact definition of LTMVD, particularly in regard to an actual time frame. In 2005, the National Association for Medical Direction of Respiratory Care (NAMDRC) Consensus Panel recommended that LTMVD (which they referred to as prolonged mechanical ventilation) be defined as “the need for ≥21 consecutive days of mechanical ventilation for ≥6 hours per day.”90
Etiology and Pathophysiology
A wide variety of physiologic and psychologic factors contribute to the development of LTMVD. Physiologic factors include those conditions that result in decreased gas exchange, increased ventilatory workload, increased ventilatory demand, decreased ventilatory drive, and increased respiratory muscle fatigue (Box 20-25).91 Psychologic factors include those conditions that result in loss of breathing pattern control, lack of motivation and confidence, and delirium (Box 20-26).92 The development of LTMVD also is affected by the severity and duration of the patient’s current illness and any underlying chronic health problems.93
Medical and Nursing Management
The goal of medical and nursing management of the patient with LTMVD is successful weaning. The Third National Study Group on Weaning from Mechanical Ventilation, sponsored by the American Association of Critical-Care Nurses, proposed the Weaning Continuum Model, which divides weaning into three stages: preweaning, weaning process, and weaning outcome.94 It is within this framework that the management of the long-term ventilator-dependent patient is described. In addition, the common nursing diagnoses for this patient population are listed in Box 20-27.
Preweaning Stage
For the long-term ventilator-dependent patient, the preweaning phase consists of resolving the precipitating event that necessitated ventilatory assistance and preventing the physiologic and psychologic factors that can interfere with weaning. Before any attempts at weaning, the patient should be assessed for weaning readiness, an approach should be determined, and a method should be selected.92,93
Weaning Preparedness.
The patient should be physiologically and psychologically prepared to initiate the weaning process by addressing those factors that can interfere with weaning. Aggressive medical management to prevent and treat V/Q mismatching, intrapulmonary shunting, anemia, heart failure, decreased lung compliance, increased airway resistance, acid–base disturbances, hypothyroidism, abdominal distention, and electrolyte imbalances should be initiated. In addition, interventions to decrease the work of breathing should be implemented, such as replacing a small endotracheal tube with a larger tube or a tracheostomy, suctioning airway secretions, administering bronchodilators, optimizing the ventilator settings and trigger sensitivity, and positioning the patient in straight alignment with the head of the bed elevated at least 30 degrees. Enteral or parenteral nutrition should be started and the patient’s nutritional state optimized. Physical therapy should be initiated for the patient with critical illness polyneuropathy because increased mobility facilitates weaning. A means of communication should be established with the patient. Sedatives can be administered to provide anxiety control, but the avoidance of respiratory depression is critical.95
Weaning Readiness.
Although a variety of different methods for assessing weaning readiness have been developed, none has proven to be very accurate in predicting weaning success in the patient with LTMVD. One study did indicate that the presence of left ventricular dysfunction, fluid imbalance, and nutritional deficiency increased the duration of mechanical ventilation. Another study suggested that the upward trending of the albumin level may be predictive of weaning success. Because so many variables can affect the patient’s ability to wean, any assessment of weaning readiness should incorporate these variables. Cardiac function, gas exchange, pulmonary mechanics, nutritional status, electrolyte and fluid balance, and motivation should all be considered when making the decision to wean. This assessment should be ongoing to reflect the dynamic nature of the process.96
Weaning Approach.
Although weaning the patient requiring short-term mechanical ventilation is a relatively simple process that can usually be accomplished with a nurse and respiratory therapist, weaning the patient with LTMVD is a much more complex process that usually requires a multidisciplinary team approach. Multidisciplinary weaning teams that use a coordinated and collaborative approach to weaning have demonstrated improved patient outcomes and decreased weaning times. The team should consist of a physician; a nurse; a respiratory therapist; a dietitian; a physical therapist; and a case manager, a clinical outcomes manager, or a clinical nurse specialist. Additional members, if possible, should include an occupational therapist, a speech therapist, a discharge planner, and a social worker. Working together, the team members should develop a comprehensive plan of care for the patient that is efficient, consistent, progressive, and cost effective.97 Several studies have demonstrated successful weaning through the use of nurse and respiratory therapist-managed protocols.98
Collaborative management of the patient requiring long-term mechanical ventilation is outlined in Fig. 20-9.
Weaning Method.
A variety of weaning methods are available, but no one method has consistently proven to be superior to the others. These methods include T-tube (T-piece), CPAP, pressure support ventilation (PSV), and SIMV. One recent multicenter study lends evidence to support the use of PSV for weaning over T-tube or SIMV weaning. Often these weaning methods are used in combination with each other, such as SIMV with PSV, CPAP with PSV, or SIMV with CPAP.91,93
Weaning Process Stage
For the long-term ventilator patient, the weaning process phase consists of initiating the weaning method selected and minimizing the physiologic and psychologic factors that can interfere with weaning.91,92 It is imperative that the patient not become exhausted during this phase, because this can result in a setback in the weaning process.95 During this phase, the patient is assessed for weaning progress and signs of weaning intolerance.93
Weaning Initiation.
Weaning should be initiated in the morning while the patient is rested. Before starting the weaning process, the patient is provided with an explanation of how the process works, a description of the sensations to expect, and reassurances that he or she will be closely monitored and returned to the original ventilator mode and settings if any difficulty occurs.93 This information should be reinforced with each weaning attempt. One study showed that family presence during the weaning trial was beneficial and that the trials were longer when the family was present.99
T-tube and CPAP weaning are accomplished by removing the patient from the ventilator and then placing the patient on a T-tube or by placing the patient on CPAP mode for a specified duration of time, known as a weaning trial, for a specified number of times per day. When the weaning trial is over, the patient is placed on the assist-control mode or similar mode and allowed to rest to prevent respiratory muscle fatigue. Gradually the duration of time spent weaning is increased, as is the frequency, until the patient is able to breathe spontaneously for 24 hours. If PSV is used in conjunction with CPAP, the PSV is initially set to provide the patient with an assisted tidal volume of 10 to 12 mL/kg, and this is gradually weaned until a level of 6 to 8 cm H2O of pressure support is achieved. SIMV and PSV weaning are accomplished by gradually decreasing the number of breaths or the amount of pressure support the patient receives by a specified amount until the patient is able to breathe spontaneously for 24 hours.93
Weaning Progress.
Weaning progress can be evaluated using various methods. Evaluation of weaning progress when using a weaning method that gradually withdraws ventilatory support, such as SIMV or PSV, can be accomplished by measuring the percentage of the minute ventilation requirement that is provided by the ventilator. If the percentage steadily decreases, weaning is progressing. Evaluation of weaning progress when using a weaning method that removes ventilatory support, such as T-tube or CPAP, can be accomplished by measuring the amount of time the patient remains free from support. If the time steadily increases, weaning is progressing.93
Weaning Intolerance.
Once the weaning process has begun, the patient should be continuously assessed for signs of intolerance. When present, these signs indicate when to place the patient back on the ventilator or to return the patient to the previous ventilator settings. Commonly used indicators include dyspnea; accessory muscle use; restlessness; anxiety; change in facial expression; changes in heart rate and blood pressure; rapid, shallow breathing; and discomfort.96 Table 20-7 lists the different weaning intolerance indicators and actions that can be taken to control or prevent them.
TABLE 20-7
WEANING INTOLERANCE INDICATIONS AND INTERVENTIONS
INDICATOR | ETIOLOGY | INTERVENTION |
Pulmonary Signs (Emotional) | ||
Altered breathing pattern | Inadequate understanding of weaning process | Build trust in staff; consistent care providers |
Dyspnea intensity | Encouragement; concrete goals for extubation | |
Change in facial expression | Inability to control breathing pattern | Involve patient in process and planning daily activities |
Efficient communication established | ||
Environmental factors | Organize care; avoid interruptions during weaning | |
Adequate sleep | ||
Calm, caring presence of nurse; nonsedating anxiolytics | ||
Measure dyspnea | ||
Fan; music | ||
Biofeedback; relaxation; breathing control | ||
Family involvement; normalizing daily activities | ||
Pulmonary Signs (Physiologic) | ||
Accessory muscle use | Airway obstruction | Suction/air-mask bag unit ventilation; manually ventilate patient |
Prolonged expiration | Secretions/atelectasis | |
Asynchronous movements of chest and abdomen | Bronchospasm Patient position/kinked |
Bronchodilators Sitting upright in bed or chair or per patient preference |
Retractions | ET tube | |
Facial expression changes | Increased workload or muscle | |
Dyspnea | Fatigue | |
Shortened inspiratory time | Caloric intake | Dietary assessment |
Increased breathing frequency, decreased VT | Electrolyte imbalances | Assess electrolytes; give replacements as necessary |
Inadequate rest | Rest between weaning trials (i.e., SIMV frequency rate >5) | |
Patient/ventilator interactions | Assess ventilator settings (i.e., flow rate, trigger sensitivity) | |
Increased VE requirement | Muscle training if appropriate | |
Infection | Check for infection (treat if indicated) | |
Overfeeding | Appropriate caloric intake | |
Respiratory alkalosis | Baseline ABGs achieved (ventilate according to pH) | |
Anxiety | Coaching to regularize breathing pattern; give nonsedating anxiolytics | |
Pain | Judicious use of analgesics | |
CNS Changes | ||
Restless/irritable | Hypoxemia/hypercarbia | Increase Fio2 |
Decreased responsiveness | Return to mechanical ventilation Discern etiology and treat |
|
CV Deterioration | ||
Excessive change in BP or HR | Heart failure | Diuretics as ordered |
Increase venous return | Beta-blockers | |
Dysrhythmias | Ischemia | Increase Fio2 |
Angina | Return to mechanical ventilation | |
Dyspnea | Discern etiology and treat |
Modified from Knebel AR. When weaning from mechanical ventilation fails. Am J Crit Care. 1992;1(3):19.
Facilitative Therapies.
Additional therapies may be needed to facilitate weaning in the patient who is having difficulty making weaning progress. These therapies include ventilatory muscle training and biofeedback. Inspiratory muscle training is used to enhance the strength and endurance of the respiratory muscles. Biofeedback can be used to promote relaxation and assist in the management of dyspnea and anxiety.93
Weaning Outcome Stage
Two outcomes are possible for a patient with LTMVD: weaning completed and incomplete weaning.93
Incomplete Weaning.
Weaning is deemed incomplete when a patient has reached a plateau (5 days at the same ventilatory support level without any changes) in the weaning process despite managing the physiologic and psychologic factors that impede weaning. Thus the patient is unable to breathe spontaneously for 24 hours without full or partial ventilatory support. Once this occurs, the patient should be placed in a subacute ventilator facility or discharged home on a ventilator with home care nursing follow-up.94
Summary
Acute Lung Failure
• ALF is a clinical condition in which the pulmonary system fails to maintain adequate gas exchange; it results from a deficiency in the performance of the pulmonary system.
• Hypoxemia is the hallmark of ALF and is the result of impaired gas exchange due to alveolar hypoventilation, V/Q mismatching, or intrapulmonary shunting.
• Medical management focuses on treatment of the underlying cause, promotion of adequate gas exchange, correction of acidosis, initiation of nutrition support, and prevention of complications (i.e., ischemic-anoxic encephalopathy, cardiac dysrhythmias, VTE, and gastrointestinal bleeding).
• Nursing actions include optimizing oxygenation and ventilation (by positioning, preventing desaturation, and promoting secretion clearance), providing comfort and emotional support, maintaining surveillance for complications, and educating the patient and family.
Acute Respiratory Distress Syndrome
• ARDS is characterized by noncardiac pulmonary edema and disruption of the alveolar-capillary membrane as a result of injury to the pulmonary vasculature or the airways.
• The hallmark of ARDS is refractory hypoxemia.
• Medical management focuses on treatment of the underlying cause, promotion of gas exchange, support of tissue oxygenation, and prevention of complications.
• Nursing actions include optimizing oxygenation and ventilation, providing comfort and emotional support, and maintaining surveillance for complications.
Pneumonia
• Pneumonia is an acute inflammation of the lung parenchyma caused by an infectious agent that can lead to alveolar consolidation and can be classified as community acquired or hospital acquired.
• Medical management focuses on the initiation of antibiotic therapy, administration of oxygen and mechanical ventilation, management of fluids and nutrition support, and treatment of complications.
• Nursing actions include optimizing oxygenation and ventilation, preventing the spread of infection, providing comfort and emotional support, and maintaining surveillance for complications.
Aspiration Pneumonitis
• Aspiration pneumonitis is the presence of abnormal toxic substances in the airways and alveoli, resulting in injury to the lungs.
• Medical management focuses on clearance of the toxic substance from the airways, support of oxygenation, and maintenance of hemodynamics.
• Nursing actions include optimizing oxygenation and ventilation, preventing further aspiration events, providing comfort and emotional support, and maintaining surveillance for complications.
Pulmonary Embolism
• A PE occurs when a clot (thrombotic embolus) or other matter (nonthrombotic embolus) lodges in the pulmonary arterial system, disrupting the blood flow to a region of the lungs.
• Medical management focuses on prevention of the recurrence of PE, initiation of clot dissolution, reversal of the effects of pulmonary hypertension, promotion of gas exchange, and prevention of complications.
• Nursing actions include optimizing oxygenation and ventilation, monitoring for bleeding, providing comfort and emotional support, maintaining surveillance for complications, and educating the patient and family.
Status Asthmaticus
• Status asthmaticus is a severe asthma attack that fails to respond to conventional therapy with bronchodilators; it may result in ALF.
• Medical management focuses on support of oxygenation (by bronchodilators, corticosteroids, and oxygen therapy) and ventilation.
• Nursing actions include optimizing oxygenation and ventilation, providing comfort and emotional support, maintaining surveillance for complications, and educating the patient and family.
Air Leak Disorders
• Air leak disorders consist of those conditions that result in extra-alveolar air accumulation; they are classified into two categories, pneumothorax and barotrauma/volutrauma.
• Two conditions that require emergency intervention for immediate relief are a tension pneumothorax and a tension pneumopericardium.
• Nursing actions include optimizing oxygenation and ventilation, maintaining the chest tube system, providing comfort and emotional support, and maintaining surveillance for complications.
Long-Term Mechanical Ventilation Dependence
• LTMVD is a secondary disorder that occurs when a patient requires assisted ventilation for longer than expected given the patient’s underlying condition.
• Weaning can be divided into three stages: preweaning, weaning process, and weaning outcome.
• The preweaning phase consists of resolving the precipitating event that necessitated ventilatory assistance and preventing the physiologic and psychologic factors that can interfere with weaning.
• The weaning process phase consists of initiating the weaning method selected and minimizing the physiologic and psychologic factors that can interfere with weaning.
• Weaning is deemed successful when the patient is able to breathe spontaneously for 24 hours without ventilatory support.