Shock, Sepsis, and Multiple Organ Dysfunction Syndrome
Shock Syndrome
Description
Shock is a complex pathophysiologic process that often results in MODS and death. All types of shock involve ineffective tissue perfusion and acute circulatory failure. The shock syndrome is a pathway involving a variety of pathologic processes that may be categorized as four stages: initial, compensatory, progressive, and refractory. Progression through each stage varies with the patient’s prior condition, duration of initiating event, response to therapy, and correction of underlying cause (Fig. 35-1).
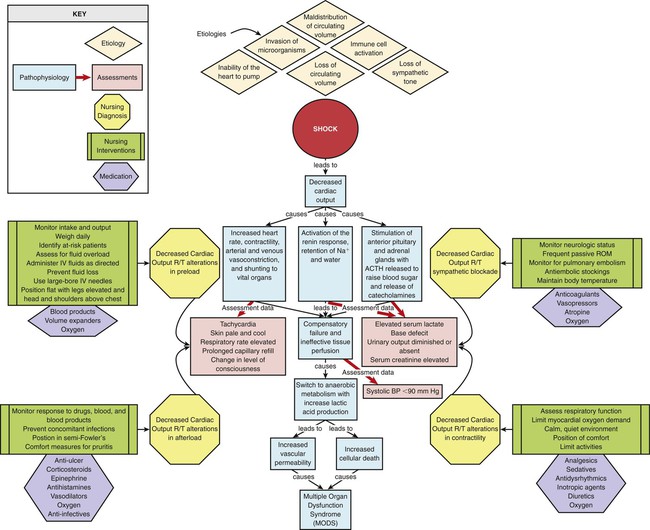
Pathophysiology
During the progressive stage, the compensatory mechanisms begin failing to meet tissue metabolic needs, and the shock cycle is perpetuated. As tissue perfusion becomes ineffective, the cells switch from aerobic to anaerobic metabolism to produce energy. Anaerobic metabolism produces small amounts of energy but large amounts of lactic acid, producing lactic acidemia. Vasodilation and increased vascular permeability from endothelial and epithelial hypoxia and inflammatory mediators results in intravascular hypovolemia, tissue edema, and further decline in tissue perfusion.1,2,3 A systemic release of inflammatory mediators in response to tissue hypoxia, especially in gut tissue, produces microcirculatory impairment and derangement of cellular metabolism, facilitating progression of the shock cycle.1–4 The patient is experiencing systemic inflammatory response syndrome (SIRS), and irreversible damage begins to occur. Some cells die as a result of apoptosis, an injury-activated, preprogrammed cellular suicide. Others die as the sodium–potassium pump in the cell membrane fails, causing the cell and its organelles to swell. Cellular energy production comes to a complete halt as the mitochondria swell and rupture. At this point, the problem becomes one of oxygen use instead of oxygen delivery. Even if the cell were to receive more oxygen, it would be unable to use it because of damage to the mitochondria. The cell’s digestive organelles swell and leak destructive enzymes into the cell, accelerating cell death.4
Every system in the body is affected by this process (Box 35-1). Cardiac dysfunction develops as a result of the release of myocardial depressant cytokines.1–3 Ventricular failure eventually occurs, further perpetuating the entire process. Central nervous system (CNS) dysfunction develops as a result of cerebral hypoperfusion, leading to failure of the SNS, cardiac and respiratory depression, and thermoregulatory failure. Endothelial injury from hypoxia and inflammatory cytokines and impaired blood flow result in microvascular thrombosis. Hematologic dysfunction occurs as a result of consumption of clotting factors, release of inflammatory cytokines, and dilutional thrombocytopenia. Disseminated intravascular coagulation (DIC) eventually may develop. Pulmonary dysfunction occurs as a result of increased pulmonary capillary membrane permeability, pulmonary microemboli, and pulmonary vasoconstriction. Ventilatory failure and acute respiratory distress syndrome (ARDS) develop. Renal dysfunction develops as a result of renal vasoconstriction and renal hypoperfusion, leading to acute kidney injury (AKI). Gastrointestinal dysfunction occurs as a result of splanchnic vasoconstriction and hypoperfusion and leads to failure of the gut organs. Disruption of the intestinal epithelium releases gram-negative bacteria into the system, which further perpetuates the entire shock syndrome.5
During the refractory stage, shock becomes unresponsive to therapy and is considered irreversible. As the individual organ systems die, MODS—defined as failure of two or more body systems—occurs. Death is the final outcome. Regardless of the etiologic factors, death occurs from ineffective tissue perfusion because of the failure of the circulation to meet the oxygen needs of the cell.4
Assessment and Diagnosis
The patient with a mean arterial blood pressure (MAP) less than 60 mm Hg or with evidence of global tissue hypoperfusion is considered to be in a shock state.1,3 Because shock is a dynamic physiologic phenomenon, hypotension may occur late in the process or may normalize even when tissue perfusion is still inadequate.6–9 Clinical manifestations vary according to the underlying cause of shock, the stage of the shock, and the patient’s response to shock.
Compensatory mechanisms may produce normal hemodynamic values even when tissue perfusion is compromised.3,5,8,10–11 Global indicators of systemic perfusion and oxygenation include serum lactate, arterial base deficit, serum bicarbonate, and central or mixed venous oxygen saturation levels. Inadequate cellular oxygenation with anaerobic metabolism and increased metabolic lactate production increase the serum lactate level.8,12 The level and duration of this hyperlactatemia are predictive of morbidity and mortality,7,10–12 and management guided by lactate levels has been effective in improving outcomes.13–14 The base deficit derived from arterial blood gas (ABG) values also reflects global tissue acidosis and is useful to assess the severity of shock.6,9–11 Studies have demonstrated serum bicarbonate to be an equivalent alternative to arterial base deficit in predicting mortality in surgical and trauma patients.15–16 The use of mixed venous oxygen saturation (Svo2) measured by means of a pulmonary artery catheter or central venous oxygen saturation (Scvo2) measured with a central venous catheter allows assessment of the balance of oxygen delivery and oxygen consumption and the ratio of oxygen extraction.3,17–19 After years of recommended use to guide the care of patients with severe sepsis, this measure of global oxygen balance is being evaluated for use in other critically ill populations.17–21 Noninvasive indicators of regional tissue perfusion or oxygenation such as sublingual capnometry and subcutaneous or skeletal muscle tissue oxygen saturation (Sto2) measured with near-infrared spectroscopy are also being evaluated.3,8,19 The sections on different types of shock discuss clinical assessment and diagnosis of the patient in shock.
Medical Management
The major focus of the treatment of shock is the improvement and preservation of tissue perfusion. Adequate tissue perfusion depends on an adequate supply of oxygen being transported to the tissues and the cell’s ability to use it. Oxygen transport is influenced by pulmonary gas exchange, CO, and hemoglobin level. Oxygen use is influenced by the internal metabolic environment and mitochondrial function. Management of the patient in shock focuses on supporting oxygen delivery.1,3
The quantity and choice of fluid is a subject of debate and depends on the situation.3,22–27 Excessive volume expansion, more than what increases preload and stroke volume (SV), worsens organ function and may produce coagulopathy, cytokine activation, and abdominal compartment syndrome.3,24 Methods to measure preload responsiveness include respiratory or positional variation in pulse pressure, systolic pressure, and SV and are more accurate than central venous pressure (CVP).3 Fluid resuscitation with normal saline or with albumin produces similar outcomes regardless of baseline serum albumin level.26,28 Crystalloid solutions are inexpensive and effective. Advantages of colloids include faster restoration of intravascular volume and use of smaller amounts. Colloids are believed to stay in the intravascular space, unlike crystalloids, which readily leak into the extravascular space. Disadvantages include expense, allergic reactions, and difficulties in typing and cross-matching blood. Colloids also can leak out of damaged capillaries and cause a variety of additional problems, particularly in the lungs. Hypertonic or hyperoncotic fluids offer no additional benefit over isotonic crystalloids and are not recommended.3,22,27,29–30
Blood should be considered to augment oxygen transport if the patient’s hemoglobin level is critically low, although what threshold value should be used is still undetermined.3,5,31 Transfusion of stored red blood cells does not substantially increase oxygen consumption and has been associated with immunosuppression, infection, impairment of microcirculatory flow, increased pulmonary vascular resistance, coagulopathy, and increased mortality. Restrictive transfusion practice has demonstrated lower mortality.3,5,17,31–32 Transfusion-related acute lung injury (TRALI) resulting from immune and nonimmune neutrophil activation has become the leading cause of transfusion-related death and may occur with transfusion of any plasma-containing blood or blood product.32–35
Vasoconstrictor agents are used to increase afterload by increasing the systemic vascular resistance (SVR) and improving the patient’s blood pressure level. Vasodilator agents are used to decrease preload or afterload, or both, by decreasing venous return and SVR. Positive inotropic agents are used to increase contractility. Antidysrhythmic agents are used to influence heart rate. Box 35-2 provides examples of each of these agents.
Sodium bicarbonate is not recommended in the treatment of shock-related lactic acidosis.17,36–37 No overall benefit has been found, and the risks associated with its use are significant. They include shifting of the oxyhemoglobin dissociation curve to the left, rebound increase in lactic acid production, development of hyperosmolar state, fluid overload resulting from excessive sodium, and rapid cellular electrolyte shifts.36–37
The critically ill patient should be started on enteral nutritional support therapy within 24 to 48 hours.38 The type of nutritional supplementation initiated varies according to the cause of shock, and it should be tailored to the individual patient’s needs, as indicated by the underlying condition, laboratory data, and treatment. When enteral feeding is contraindicated parental nutrition should be considered, though a delay of seven days is recommended for better outcomes.38–41 Supplementation of enteral feeding with parenteral nutrition to increase caloric intake is a subject of debate but has not been shown to improve patient outcomes.38,41–42 A delay of one week is recommended before consideration of this strategy.38
Glucose control to a target level of 140 to 180 mg/dL is recommended for all critically ill patients.43–44 Benefits of glucose control in the critically ill include lower incidences of infection, renal failure, sepsis, and death.43–46
Nursing Management
The psychosocial needs of the patient and family dealing with shock are extremely important. These needs are based on situational, familial, and patient-centered variables. Nursing interventions for the psychosocial stress of critical illness include providing information on patient status, explaining procedures and routines, supporting the family, encouraging the expression of feelings, facilitating problem solving and shared decision making, individualizing visitation schedules, involving the family in the patient’s care, and establishing contacts with necessary resources.47 The consensus of all relevant professional organizations is that patients and families should be given the option of family presence during invasive procedures and resuscitation.47–50
Collaborative management of the patient with shock is outlined in Box 35-3.
Hypovolemic Shock
Description
Hypovolemic shock occurs from inadequate fluid volume in the intravascular space. The lack of adequate circulating volume leads to decreased tissue perfusion and initiation of the general shock response. Hypovolemic shock is the most commonly occurring form of shock (Fig. 35-2).
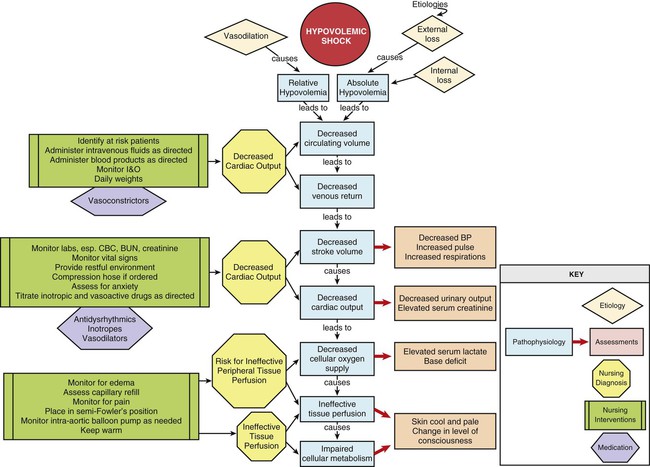
Etiology
Hypovolemic shock can result from absolute or relative hypovolemia. Absolute hypovolemia occurs when there is a loss of fluid from the intravascular space. This can result from an external loss of fluid from the body or from internal shifting of fluid from the intravascular space to the extravascular space. Fluid shifts can result from a loss of intravascular integrity, increased capillary membrane permeability, or decreased colloidal osmotic pressure. Relative hypovolemia occurs when vasodilation produces an increase in vascular capacitance relative to circulating volume (Box 35-4).
Assessment and Diagnosis
The clinical manifestations of hypovolemic shock depend on the severity of fluid loss and the patient’s ability to compensate for it. Clinical classes developed by the American College of Surgeons to describe the levels of severity of hypovolemic shock in the trauma setting have been widely accepted, but a recent test of the validity of these classes suggests that modifications are necessary.51 A simpler approach of classifying hypovolemic shock as mild, moderate, or severe is also commonly used. Class I, or mild shock, indicates a fluid volume loss up to 15% or an actual volume loss up to 750 mL. Compensatory mechanisms maintain CO, and the patient appears free of symptoms other than possibly slight anxiety.1,5,51
Class II hypovolemia occurs with a fluid volume loss of 15% to 30% or an actual volume loss of 750 to 1500 mL. As volume loss worsens, the patient moves from mild to moderate hypovolemic shock. Falling CO activates more intense compensatory responses. Anxiety increases.1 The heart rate may increase to more than 100 beats/minute in response to increased SNS stimulation unless blocked by pre-existing beta-blocker therapy. The pulse pressure narrows as the diastolic blood pressure increases because of vasoconstriction. Postural hypotension develops.1 The respiratory rate increases as blood loss worsens and ABG specimens drawn during this phase may reveal respiratory alkalosis, as evidenced by a low partial pressure of carbon dioxide (Paco2). Urine output starts to decline to 20 to 30 mL/hour as renal perfusion decreases. The urine sodium level decreases, whereas urinary osmolality and specific gravity increase as the kidneys start to conserve sodium and water. The patient’s skin becomes pale and cool with delayed capillary refill because of peripheral vasoconstriction. Jugular veins appear flat as a result of decreased venous return.1,5
Hypovolemic shock that is class III occurs with a fluid volume loss of 30% to 40% or an actual volume loss of 1500 to 2000 mL. This level of severity may produce the progressive stage of shock as compensatory mechanisms become overwhelmed and ineffective tissue perfusion develops. Blood pressure decreases, but often after tissue hypoperfusion is already significant.6,9 The heart rate may increase to more than 120 beats/minute, and dysrhythmias may develop as myocardial ischemia ensues. During this phase serum lactate levels increase and ABG values reveal metabolic acidosis evidenced by a low bicarbonate (HCO3−) and elevated base deficit. Decreased renal perfusion results in the development of oliguria. Blood urea nitrogen (BUN) and serum creatinine levels start to rise as the kidneys begin to fail. The patient’s skin becomes ashen, cold, and clammy, with marked delayed capillary refill. The patient may appear confused as cerebral perfusion decreases.1,5,51
Class IV hypovolemic shock is severe shock and usually refractory in nature. It occurs with a fluid volume loss of greater than 40% or an actual volume loss of more than 2000 mL. As the compensatory mechanisms of the body become insufficient, tachycardia and hemodynamic instability worsen and hypotension ensues. Severe lactic acidosis is present. Peripheral pulses and capillary refill become absent because of marked peripheral vasoconstriction. The skin may appear cyanotic, mottled, and extremely diaphoretic. Organ failure occurs. Urine output ceases. The patient may be confused and agitated, eventually becoming unresponsive. Various clinical manifestations associated with failure of the different body systems will develop.1,5
Assessment of the hemodynamic parameters of a patient in hypovolemic shock varies by stage but commonly reveals a decreased CO and cardiac index (CI). Loss of circulating volume leads to a decrease in venous return to the heart, which results in a decrease in the preload of the right and left ventricles. This is evidenced by a decline in the CVP or right atrial pressure (RAP) and pulmonary artery occlusion pressure (PAOP). Vasoconstriction of the arterial system results in an increase in the afterload of the heart, as evidenced by an increase in the SVR. This vasoconstriction may produce inaccurate systolic and diastolic blood pressure values when measured by arterial catheter or noninvasive oscillometry. MAP is more accurate in this low-flow state.18
Medical Management
Aggressive fluid resuscitation in trauma and surgical patients is the subject of great debate. The benefit of limited or hypotensive (systolic blood pressure 60 to 80 mm Hg or MAP 40 to 60 mm Hg) volume resuscitation in patients with uncontrolled hemorrhage is postulated to lessen bleeding and improve survival22,52–54 and has been demonstrated in the preliminary results of a randomized controlled trial.53 The type and amount of solutions used for fluid resuscitation and the rate of administration influence immune function, inflammatory mediator release, coagulation, and the incidence of cardiac, pulmonary, renal, and gastrointestinal complications.3,22,24–25,27 Consensus on the optimal resuscitative strategy for hypovolemic shock is lacking and is likely situation specific.18,22,24,52,54
Nursing Management
Management of the patient in hypovolemic shock requires continuous evaluation of intravascular volume, tissue perfusion, and response to therapy. The patient in hypovolemic shock may have any number of nursing diagnoses, depending on the progression of the process (Box 35-5). Nursing interventions include minimizing fluid loss, administering volume replacement, assessing response to therapy, providing comfort and emotional support, and preventing and maintaining surveillance for complications.
Measures to minimize fluid loss include limiting blood sampling, observing lines for accidental disconnection, and applying direct pressure to bleeding sites. Measures to facilitate the administration of volume replacement include insertion of large-bore peripheral intravenous catheters, rapid administration of prescribed fluids, and positioning the patient with the legs elevated, trunk flat, and head and shoulders above the chest. Monitoring the patient for clinical manifestations of fluid overload or complications related to fluid and blood product administration is essential for preventing further problems. It is also essential to monitor the patient for SIRS, which may occur for up to several days after resuscitation.55
Cardiogenic Shock
Description
Cardiogenic shock is the result of failure of the heart to effectively pump blood forward. It can occur with dysfunction of the right or the left ventricle, or both. The lack of adequate pumping function leads to decreased tissue perfusion and circulatory failure (Fig. 35-3). It occurs in approximately 5% to 8% of the patients with an ST-segment myocardial infarction (MI), and it is the leading cause of death of patients hospitalized with MI.56–58 The mortality rate for cardiogenic shock has decreased with the advent of early revascularization therapy and is currently about 47% to 65%.56–60
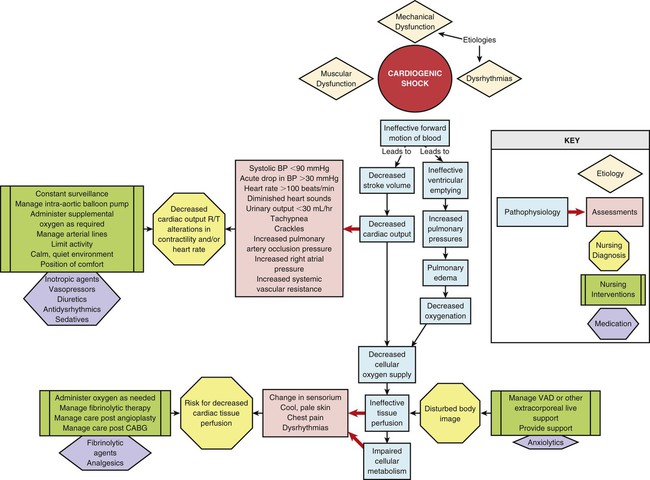
Etiology
Cardiogenic shock can result from problems affecting the muscular function or the mechanical function of the heart or the cardiac rhythm.57–59,61 The most common cause is acute MI resulting in the loss of 40% or more of the functional myocardium. It can occur with ST-elevation or non–ST-elevation MI.57–59 The damage to the myocardium may occur after one massive MI (usually of the anterior wall), or it may be cumulative as a result of several smaller MIs or a small MI in a patient with pre-existing ventricular dysfunction.56–57 Cardiomyopathy may cause cardiogenic shock as left ventricular function becomes unable to maintain adequate CO. Examples of problems affecting the mechanical function of the heart to fill and eject adequately include severe valvular disease; acute papillary muscle, chordal, or septal rupture; cardiac tamponade; and massive pulmonary embolus (Box 35-6).57–59,61
Pathophysiology
Cardiogenic shock results from the impaired ability of the ventricle to pump blood forward, which leads to a decrease in SV and an increase in the blood left in the ventricle at the end of systole. The decrease in SV results in a decrease in CO, which leads to decreased cellular oxygen supply and ineffective tissue perfusion. Typically, myocardial performance spirals downward as compensatory vasoconstriction increases myocardial afterload and low blood pressure worsens myocardial ischemia. As left ventricular contractility declines and ventricular compliance decreases, an increase in end-systolic volume results in blood backing up into the pulmonary system and the subsequent development of pulmonary edema. Pulmonary edema causes impaired gas exchange and decreased oxygenation of the arterial blood, which further impair tissue perfusion. In a substantial number of patients, the pathophysiology may follow a different course due to activation of inflammatory cytokines. A SIRS response results with systemic vasodilation and, possibly, normalization of the CO.57–58,62 Whether this process contributes to the genesis or the outcome of cardiogenic shock is uncertain, but it is thought to be activated by acute MI and to facilitate development of sepsis.57,62 Death due to cardiogenic shock results from cardiopulmonary collapse or multiple organ failure.59
Assessment and Diagnosis
A variety of clinical manifestations occur in the patient in cardiogenic shock, depending on etiologic factors, the patient’s underlying medical status, and the severity of the shock state. Some clinical manifestations are caused by failure of the heart as a pump, whereas many are related to the overall shock response (Box 35-7).
Initially, clinical manifestations reflect the decline in CO. These signs and symptoms include systolic blood pressure less than 90 mm Hg or an acute drop in systolic or mean blood pressure of 30 mm Hg or more; decreased sensorium; cool, pale, moist skin; and urine output of less than 30 mL/hour.57,59,63 The patient also may complain of chest pain. Tachycardia develops to compensate for the decrease in CO. A weak, thready pulse develops, and diminished S1 and S2 heart sounds may occur as a result of the decreased contractility. The respiratory rate increases to improve oxygenation. ABG values at this point indicate respiratory alkalosis, as evidenced by a decrease in Paco2. Urinalysis findings demonstrate a decrease in urine sodium level and an increase in urine osmolality and specific gravity as the kidneys start to conserve sodium and water. Serum B-type natriuretic peptide (BNP) levels will likely be elevated.
As the left ventricle fails, auscultation of the lungs may disclose crackles and rhonchi, indicating the development of pulmonary edema. Hypoxemia occurs, as evidenced by a fall in Pao2 and Sao2 as measured by ABG values. Heart sounds may reveal an S3 and S4. Jugular venous distention is evident with right-sided failure. The patient also may experience dysrhythmias in response to tissue hypoxia, the underlying problem, and drug therapy.58–59
Assessment of the hemodynamic parameters of a patient in cardiogenic shock reveals a decreased CO with a CI less than 2.2 L/min/m2 in the presence of an elevated PAOP of more than 15 mm Hg.57,59,63 A proportional pulse pressure (systolic BP/pulse pressure) less than 25% is indicative of left ventricular failure and a CI less than 2.2 and may be useful when direct measurement of CI is unavailable.64 Increased filling pressures are necessary to rule out hypovolemia as the cause of circulatory failure. The increase in PAOP reflects an increase in the left ventricular end-diastolic pressure (LVEDP) and left ventricular end-diastolic volume (LVEDV) resulting from decreased SV. With right ventricular failure, the RAP also increases. Compensatory vasoconstriction typically results in an increase in the afterload of the heart, as evidenced by an increase in the SVR, unless SIRS produces vasodilation and a normal or decreased SVR. Echocardiography confirms the diagnosis of cardiogenic shock, provides noninvasive estimates of PAOP and ejection fraction, and often clarifies etiologic factors.57–59