Chapter 11 Principles of Electropharmacology
Long before actual medicines were available for arrhythmias, much had been written about the theoretical mechanisms of arrhythmias.1,2 Today, these studies still provide a rational argument for why a certain drug with certain properties should be an effective antiarrhythmic agent. For example, as discussed in Chapter 2, re-entrant excitation involves a circulating excitatory wavefront along a certain path and has a certain conduction velocity (CV) and refractoriness (effective refractory period [ERP]); thus we have the concept that wavelength (λ) = CV × ERP. Depending on path length and the value of λ, the re-entrant circuit will have an excitable gap. Any change in λ would be expected to determine the inducibility and stability of re-entrant excitation. On the basis of this concept, antiarrhythmic drugs are known to affect properties of cardiac excitability (class I and IV drugs) and refractoriness (class III drugs). Class II drugs remain specific for blocking β-adrenergic receptors. Thus what has emerged is a classification of drugs that depends on a drug’s effect on certain ionic channels or receptors of the cardiac cell sarcolemma (Vaughan Williams classification).3 However, as a result of the Cardiac Arrhythmia Suppression Trial (CAST), which was designed to suppress premature ventricular depolarizations, some of these drugs lost favor, particularly for treatment of ventricular arrhythmias.
Thus most drugs currently used interrupt the direct mediators of electrogenesis, cardiac ion channels, by affecting the channel pore, the channel’s gating mechanism, or both. In 2001, the members of the Sicilian Gambit, while not disposing of the Vaughan Williams drug classification, emphasized the important and emerging role of new drug targets for pharmacological therapy and/or prevention (Figure 11-1).4 These suggestions were based on the concept that most hearts that need antiarrhythmic drugs are “remodeled,” which means that drugs are not working at all or not working well because the fundamental nature of the ion channel “pore” or channel gating mechanism has been altered by an underlying disease. A clear example here is the effect of flecainide in patients following myocardial infarction (CAST) and its effect on a re-entrant circuit and the remodeled sodium channels of cells surviving in the infarcted heart.5,6
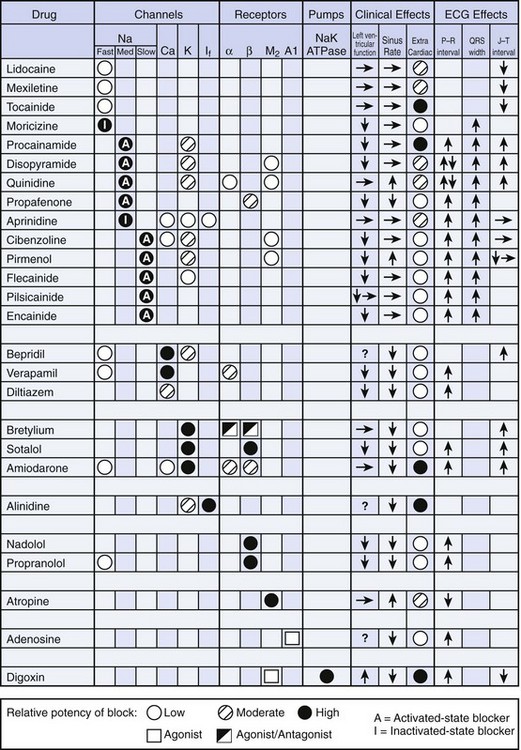
FIGURE 11-1 Drug targets for pharmacological therapy and intervention.
(From New approaches to antiarrhythmic therapy, part I: Emerging therapeutic applications of the cell biology of cardiac arrhythmias, Circulation 104:2865–2873, 2001.)
Biology and Biophysics of Cardiac Ion Channels
A drug that blocks inward plateau currents would be considered an anti–class III drug in that it should shorten APD. An antianginal drug, ranolazine, is being investigated for its antiarrhythmic properties, since it strongly inhibits the late INa current with little or no effect on peak INa.7 Thus ranolazine would shorten APD rather than reduce excitability in cardiac tissues. More interestingly, this drug, by blocking sodium (Na+) influx into the cardiac cell, would be expected to reduce intracellular Na+ and therefore would indirectly affect intracellular Ca2+ and all its sequelae (see below).
One form of re-entrant excitation is anisotropic re-entry, which was first considered in atrial samples.8 In this type of re-entry, conduction in the longitudinal direction is faster than that in the transverse direction. Spach and his colleagues suggested that a component of conduction slowing in the transverse direction in these cardiac tissues was caused by a change in gap junctional conductance. Thus slowing of conduction was brought about by the uncoupling of cells at the level of the gap junctional proteins, for example, connexins 43 and 40 (Cx43 and Cx40). While experiments have clearly shown that gap junctional uncouplers (e.g., heptanol) are, in fact, arrhythmogenic, a preferential effect appears to occur on transverse conduction velocities in various animal models. Thus a corollary would be that in re-entrant circuits in highly remodeled substrates such as those affected by cell uncoupling caused by ischemia, one would expect drugs that enhance gap junctional conductance to be antiarrhythmic. Rotigaptide is now under study as a gap junctional coupler. In experimental arrhythmia models, this drug improves conduction and abolishes lines of block that perpetuate circuits and therefore has been deemed antiarrhythmic. This experimental drug is thought to affect coupling by preventing dephosphorylation of Cx43, a ventricular gap junctional protein, or by maintaining phosphorylation of the protein.
Factors Contributing to Cardiac Remodeling
Altered Mediators of Electrogenesis
Cardiac remodeling is an all-encompassing term that can refer to both structural and functional changes in cardiac cells in response to a disease. It is an adaptive response of the heart that, when over-compensated, can be maladaptive.4
The cardiac cellular action potential changes differ in several types of disease, from chronic reduction in excitability (post-MI hearts), to APD prolongation in heart failure, to APD shortening in chronic AF. As such, the cellular ionic channel changes in different acquired diseases are varied, from a loss in Na+ current function in cells of the epicardial border zone after MI to the enhancement of the constitutively active IKAch in atrial cells in AF. In some cases, drugs have been developed to specifically target the remodeled channels of the diseased cell. The best example is the development of tertiapin-Q–type drugs that block IKAch to prolong atrial APDs shortened by muscarinic activation of IKAch. Tertiapin-Q is a highly selective blocker of Kir3, the ion channel subunit that underlies IKAch. It can significantly prolong the duration of the AF-remodeled atrial AP, where IKach activity is upregulated.9