38 Postoperative Pain Management for the Cardiac Patient
Adequate postoperative analgesia prevents unnecessary patient discomfort, may decrease morbidity, may decrease postoperative hospital length of stay, and thus may decrease cost. Because postoperative pain management has been deemed important, the American Society of Anesthesiologists has published practice guidelines regarding this topic.1 Furthermore, in recognition of the need for improved pain management, the Joint Commission on Accreditation of Healthcare Organizations has developed new standards for the assessment and management of pain in accredited hospitals and other health care settings.2 Patient satisfaction (no doubt linked to adequacy of postoperative analgesia) has become an essential element that influences clinical activity of not only anesthesiologists but all healthcare professionals.
Achieving optimal pain relief after cardiac surgery is often difficult. Pain may be associated with many interventions, including sternotomy, thoracotomy, leg-vein harvesting, pericardiotomy, and/or chest tube insertion, among others. Inadequate analgesia and/or an uninhibited stress response during the postoperative period may increase morbidity by causing adverse hemodynamic, metabolic, immunologic, and hemostatic alterations.3–5 Aggressive control of postoperative pain, associated with an attenuated stress response, may decrease morbidity and mortality in high-risk patients after noncardiac surgery6,7 and may also decrease morbidity and mortality in patients after cardiac surgery.8,9 Adequate postoperative analgesia may be attained via a wide variety of techniques (Table 38-1). Traditionally, analgesia after cardiac surgery has been obtained with intravenous opioids (specifically morphine). However, intravenous opioid use is associated with definite detrimental side effects (nausea/vomiting, pruritus, urinary retention, respiratory depression), and longer-acting opioids such as morphine may delay tracheal extubation during the immediate postoperative period via excessive sedation and/or respiratory depression. Thus, in the current era of early extubation (“fast-tracking”), cardiac anesthesiologists are exploring unique options other than traditional intravenous opioids for control of postoperative pain in patients after cardiac surgery.10,11 No single technique is clearly superior; each possesses distinct advantages and disadvantages. It is becoming increasingly clear that a multimodal approach/combined analgesic regimen (utilizing a variety of techniques) is likely the best way to approach postoperative pain (in all patients after surgery) to maximize analgesia and minimize side effects. When addressing postoperative analgesia in cardiac surgical patients, choice of technique (or techniques) is made only after a thorough analysis of the risk/benefit ratio of each technique in the specific patient in whom analgesia is desired.
TABLE 38-1 Techniques Available for Postoperative Analgesia
Pain and cardiac surgery
Surgical or traumatic injury initiates changes in the peripheral and central nervous systems (CNSs) that must be addressed therapeutically to promote postoperative analgesia and, it is hoped, positively influence clinical outcome (Boxes 38-1 and 38-2). The physical processes of incision, traction, and cutting of tissues stimulate free nerve endings and a wide variety of specific nociceptors. Receptor activation and activity are further modified by the local release of chemical mediators of inflammation and sympathetic amines released via the perioperative surgical stress response. The perioperative surgical stress response peaks during the immediate postoperative period and exerts major effects on many physiologic processes. The potential clinical benefits of attenuating the perioperative surgical stress response (above and beyond simply attaining adequate clinical analgesia) have received much attention during the 2000s and remain fairly controversial.12 However, it is clear that inadequate postoperative analgesia and/or an uninhibited perioperative surgical stress response has the potential to initiate pathophysiologic changes in all major organ systems, including the cardiovascular, pulmonary, gastrointestinal, renal, endocrine, immunologic, and/or CNSs, all of which may lead to substantial postoperative morbidity.
Pain after cardiac surgery may be intense and it originates from many sources, including the incision (sternotomy, thoracotomy, etc.), intraoperative tissue retraction and dissection, vascular cannulation sites, vein-harvesting sites, and chest tubes, among others.13,14 Patients in whom an internal mammary artery (IMA) is surgically exposed and used as a bypass graft may have substantially more postoperative pain.15
A prospective clinical investigation involving 200 consecutive patients undergoing cardiac surgery via median sternotomy assessed the location, distribution, and intensity of postoperative pain.13 All patients received 25 to 50 μg/kg intraoperative intravenous fentanyl, were subjected to routine cardiopulmonary bypass (CPB), had their arms positioned along their body on the operating table, had their sternum closed with five peristernal wires, and received mediastinal and thoracic drains passed through the rectus abdominis muscle just below the xiphoid. A subgroup (127 patients) also underwent long saphenous vein harvesting either from the calf (men) or thigh (women). All patients were extubated before the first postoperative morning. Postoperative analgesic management was standardized and included intravenous morphine, oral paracetamol, oral tramadol, and subcutaneous morphine. Pain location, distribution, and intensity were documented in the morning on the first, second, third, and seventh postoperative days using a standardized picture dividing the body into 32 anatomic areas. A numerical rating scale of 0 to 10 (with 0 representing no pain and 10 representing worst possible pain) was used to assess maximal pain intensity.
These investigators found that maximal pain intensity was highest on the first postoperative day and lowest on the third postoperative day. However, maximal pain intensity was only graded as “moderate” (mean pain score was approximately 3.8) and did not diminish during the first 2 postoperative days, yet started to decline between postoperative days 2 and 3. Pain distribution did not appear to vary throughout the postoperative period, yet location did (more shoulder pain observed on the seventh postoperative day). As time after surgery increased, pain usually moved from primarily incisional/epigastric to osteoarticular. Another source of postoperative pain in patients after cardiac surgery is thoracic cage rib fractures, which may be common.16,17 Furthermore, sternal retraction, causing posterior rib fracture, may lead to brachial plexus injury. In these patients, routine chest radiographs may be normal despite the presence of fracture. Thus, bone scans (better at detecting rib fractures than chest radiographs) are recommended whenever there is unexplained postoperative nonincisional pain in a patient who has undergone sternal retraction.17 Other studies have indicated that the most common source of pain in patients after cardiac surgery is the chest wall. Age also appears to impact pain intensity; patients younger than 60 often have greater pain intensity than patients older than 60. Although maximal pain intensity after cardiac surgery is usually only moderate, there remains ample room for clinical improvement in analgesic control to minimize pain intensity, especially during the first few postoperative days.
Persistent pain after cardiac surgery, although rare, can be problematic.18–20 The cause of persistent pain after sternotomy is multifactorial, yet tissue destruction, intercostal nerve trauma, scar formation, rib fractures, sternal infection, stainless-steel wire sutures, and/or costochondral separation may all play roles. Such chronic pain is often localized to the arms, shoulders, or legs. Postoperative brachial plexus neuropathies also may occur and have been attributed to rib fracture fragments, IMA dissection, suboptimal positioning of patients during surgery, and/or central venous catheter placement. Postoperative neuralgia of the saphenous nerve has also been reported after harvesting of saphenous veins for coronary artery bypass grafting (CABG). Younger patients appear to be at greater risk for development of chronic, long-lasting pain. The correlation of severity of acute postoperative pain and development of chronic pain syndromes has been suggested (patients requiring more postoperative analgesics may be more likely to develop chronic pain), yet the causative relation is still vague.
Ho and associates18 assessed via survey 244 patients after cardiac surgery and median sternotomy and found that persistent pain (defined as pain still present 2 or more months after surgery) was reported in almost 30% of patients. The incidence rate of persistent pain at any site was 29% (71 patients) and for sternotomy was 25% (61 patients). Other common locations of persistent pain reported to these investigators were the shoulders (17.4%), back (15.9%), and neck (5.8%). However, such persistent pain was usually reported as mild, with only 7% of patients reporting interference with daily living. The most common words used to describe the persistent pain were “annoying” (57%), “nagging” (33%), “dull” (30%), “sharp” (25%), “tiring” (22%), “tender” (22%), and “tight” (22%). The temporal nature of this pain was mostly reported as being brief/transient and periodic/intermittent. Twenty patients (8%) also described symptoms of numbness, burning pain, and tenderness over the IMA-harvesting site, symptoms suggestive of IMA syndrome. Thus, it was concluded that mild persistent pain after cardiac surgery and median sternotomy is common yet only infrequently substantially interferes with daily life.
Although the most common source of pain in patients after cardiac surgery remains the chest wall, leg pain from vein-graft harvesting can be problematic as well. Such pain may not become apparent until the late postoperative period, which may be related to the progression of patient mobilization, as well as the decreasing impact of sternotomy pain (unmasking leg incisional pain). The recent utilization of minimally invasive vein-graft harvesting techniques (endoscopic vein-graft harvesting) decreases postoperative leg pain intensity and duration compared with conventional open techniques.21 Although initial harvest times may be prolonged, harvest times become equivalent between the two techniques (endoscopic vs. conventional) once a short learning curve is overcome. Furthermore, leg morbidity (infection, dehiscence, etc.) may be less in patients undergoing endoscopic vein harvest compared with patients undergoing conventional open techniques because of different incisional lengths.
Patient satisfaction with quality of postoperative analgesia is as much related to the comparison between anticipated and experienced pain as it is to the actual level of pain experienced. Satisfaction is related to a situation that is better than predicted, dissatisfaction to one that is worse than expected. Patients undergoing cardiac surgery remain concerned regarding the adequacy of postoperative pain relief and tend to preoperatively expect a greater amount of postoperative pain than that which is actually experienced.14 Because of these unique preoperative expectations, patients after cardiac surgery who receive only moderate analgesia postoperatively will likely still be satisfied with their pain control. Thus, patients may experience pain of moderate intensity after cardiac surgery yet still express very high satisfaction levels.14,15
Potential clinical benefits of adequate postoperative analgesia
Inadequate analgesia (coupled with an uninhibited stress response) during the postoperative period may lead to many adverse hemodynamic (tachycardia, hypertension, vasoconstriction), metabolic (increased catabolism), immunologic (impaired immune response), and hemostatic (platelet activation) alterations. In patients undergoing cardiac surgery, perioperative myocardial ischemia (diagnosed by electrocardiography [ECG] and/or transesophageal echocardiography) is most commonly observed during the immediate postoperative period and appears to be related to outcome.22,23 Intraoperatively, initiation of CPB causes substantial increases in stress response hormones (norepinephrine, epinephrine, etc.) that persist into the immediate postoperative period and may contribute to myocardial ischemia observed during this time.24–26 Furthermore, postoperative myocardial ischemia may be aggravated by cardiac sympathetic nerve activation, which disrupts the balance between coronary blood flow and myocardial oxygen demand.27 Thus, during the pivotal immediate postoperative period after cardiac surgery, adequate analgesia (coupled with stress–response attenuation) may potentially decrease morbidity and enhance health-related quality of life.27,28
Evidence exists indicating that aggressive control of postoperative pain in patients after noncardiac surgery may beneficially affect outcome.6,7 In 1987, Yeager et al,7 in a small (n = 53 patients), randomized, controlled clinical trial involving patients undergoing major thoracic/vascular surgery, revealed that patients who were managed with more intense perioperative anesthesia and analgesia demonstrated decreased postoperative morbidity and improved operative outcome. In 1991, Tuman et al,6 in another small (n = 80 patients), randomized, controlled clinical trial involving patients undergoing lower extremity revascularization, revealed that patients who were managed with more intense perioperative anesthesia and analgesia demonstrated improved outcome compared with patients receiving routine on-demand narcotic analgesia.
Evidence also exists that aggressive control of postoperative pain in patients after cardiac surgery may beneficially affect outcome. Two intriguing clinical investigations published in 1992 hint at such possibilities.8,9 Mangano et al8 prospectively randomized 106 adult patients undergoing elective CABG to receive either standard postoperative analgesia or intensive analgesia during the immediate postoperative period. Standard-care patients received low-dose intermittent intravenous morphine for the first 18 postoperative hours, whereas intensive-analgesia patients received a continuous intravenous sufentanil infusion during the same time period. Patients receiving sufentanil demonstrated a lesser severity of myocardial ischemia episodes (detected by continuous ECG monitoring) during the immediate postoperative period. The authors postulated that the administration of intensive analgesia during the immediate postoperative period may have more completely suppressed sympathetic nervous system activation, thereby having numerous beneficial clinical effects, including beneficial alterations in sensitivity of platelets to epinephrine, beneficial alterations in fibrinolysis, enhanced regional left ventricular function, and decreased coronary artery vasoconstriction, all potentially leading to a reduced incidence/severity of myocardial ischemia. Anand and Hickey9 prospectively randomized 45 neonates undergoing elective cardiac surgery (mixed procedures) to receive either standard perioperative care or deep opioid anesthesia. Standard-care patients received a halothane/ketamine/morphine anesthetic with intermittent intravenous morphine for the first 24 postoperative hours, whereas deep-opioid patients received an intravenous sufentanil anesthetic with a continuous infusion of either intravenous fentanyl or intravenous sufentanil during the same postoperative time period. Neonates receiving continuous postoperative opioid infusions demonstrated a reduced perioperative stress response (assessed via multiple blood mediators), less perioperative morbidity (hyperglycemia, lactic acidemia, sepsis, metabolic acidosis, disseminated intravascular coagulation), and significantly fewer deaths than the control group (0/30 vs. 4/15, respectively; p < 0.01).
The accompanying editorial accurately summarizes this clinical investigation: “What Anand and Hickey have shown is that this reluctance to treat pain adequately is not a necessary evil. It markedly contributes to a bad outcome.”29 Unfortunately, aggressive control of postoperative pain in patients after cardiac surgery with relatively large amounts of intravenous opioids in this manner does not allow tracheal extubation in the immediate postoperative period (a goal of current practice).
Techniques available for postoperative analgesia
Although the mechanisms of postoperative pain and the pharmacology of analgesic drugs are relatively well understood, the delivery of effective postoperative analgesia remains far from universal. Many techniques are available (see Table 38-1). In general, the American Society of Anesthesiologists Task Force on Acute Pain Management in the Perioperative Setting reports that the existing literature supports the efficacy and safety of three techniques used by anesthesiologists for perioperative pain control: regional analgesic techniques (including but not limited to intercostal blocks, plexus blocks, and local anesthetic infiltration of incisions), patient-controlled analgesia (PCA) with systemic opioids, and intrathecal/epidural opioid analgesia.1 Regarding regional analgesic techniques, the existing literature supports the analgesic efficacy of peripheral nerve blocks and postincisional infiltration with local anesthetics for postoperative analgesia, yet is equivocal regarding the analgesic benefits of preincisional infiltration. Regarding PCA with systemic opioids, the existing literature supports its efficacy (compared with intramuscular techniques) for postoperative pain management, yet the existing literature is equivocal regarding the efficacy of PCA techniques compared with nurse- or staff-administered intravenous analgesia. In addition, the existing literature is equivocal regarding the comparative efficacy of epidural PCA versus intravenous PCA techniques.
Local anesthetic infiltration
Pain after cardiac surgery is often related to median sternotomy (peaking during the first 2 postoperative days). Because of problems associated with traditional intravenous opioid analgesia (nausea and vomiting, pruritus, urinary retention, respiratory depression) and the more recently introduced nonsteroidal anti-inflammatory drugs (NSAIDs) and cyclooxygenase (COX) inhibitors (gastrointestinal bleeding, renal dysfunction), alternative methods of achieving postoperative analgesia in cardiac surgical patients have been sought. One such alternative method that may hold promise is continuous infusion of local anesthetic (Box 38-3). In a prospective, randomized, placebo-controlled, double-blind clinical trial, White et al30 studied 36 patients undergoing cardiac surgery. Intraoperative management was standardized. All patients had two indwelling infusion catheters placed at the median sternotomy incision site at the end of surgery (one in the subfascial plane above the sternum, one above the fascia in the subcutaneous tissue). Patients received 0.25% bupivacaine (n = 12), 0.5% bupivacaine (n = 12), or normal saline (n = 12) via a constant-rate infusion through the catheter (4 mL/hr) for 48 hours after surgery. Average times to tracheal extubation were similar in the three groups (approximately 5 to 6 hours). Compared with the control group (normal saline), there was a statistically significant reduction in verbal rating scale pain scores and intravenous PCA morphine use in the 0.5% bupivacaine group. Patient satisfaction with their pain management was also improved in the 0.5% bupivacaine group (vs. control). However, there were no significant differences in PCA morphine use between the 0.25% bupivacaine and control groups. Although tracheal extubation time and the duration of the intensive care unit (ICU) stay (30 vs. 34 hours, respectively) were not significantly altered, time to ambulation (1 vs. 2 days, respectively) and duration of hospital stay (4.2 vs. 5.7 days, respectively) were lower in the 0.5% bupivacaine group than in the control group.
Another clinical investigation revealed the potential benefits of using a continuous infusion of local anesthetic in patients after cardiac surgery.31 In this prospective, randomized, placebo-controlled, double-blind clinical trial, Dowling et al31 studied 35 healthy patients undergoing cardiac surgery. Patients undergoing elective CABG via median sternotomy were randomized to either “ropivacaine” or “placebo” groups. At the end of the operation, before wound closure, bilateral intercostal nerve injections from T1 to T12 were performed using 20 mL of either 0.2% ropivacaine or normal saline. After sternal reapproximation with wires, two catheters with multiple side openings were placed anterior to the sternum (Figure 38-1). These catheters were connected to a pressurized elastomeric pump containing a flow regulator, which allowed for delivery of 0.2% ropivacaine or normal saline at approximately 4 mL/hr. The intraoperative anesthetic technique was standardized (short-acting anesthetics were used to minimize the presence of residual anesthetic agents in the postoperative period), as was postoperative pain management via intravenous PCA morphine (for 72 hours).
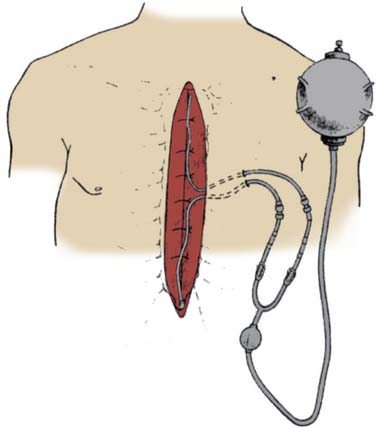
Figure 38-1 Intraoperative placement of the pressurized elastomeric pump and catheters.
(From Dowling R, Thielmeier K, Ghaly A, et al: Improved pain control after cardiac surgery: Results of a randomized, double-blind, clinical trial. J Thorac Cardiovasc Surg 126:1271, 2003.)
The management of postoperative pain with continuous direct infusion of local anesthetic into the surgical wound has been described after a wide variety of surgeries other than cardiac (inguinal hernia repair, upper abdominal surgery, laparoscopic nephrectomy, cholecystectomy, knee arthroplasty, shoulder surgery, and gynecologic operative laparoscopy).32 The infusion pump systems used for anesthetic wound perfusion are regulated by the U.S. Food and Drug Administration as medical devices. Thus, adverse events involving these infusion pump systems during direct local anesthetic infusion into surgical wounds are reported to this organization.
Complications encountered with these infusion pump systems reported to the U.S. Food and Drug Administration include tissue necrosis, surgical wound infection, and cellulitis after orthopedic, gastrointestinal, podiatric, and other surgeries. None of these reported adverse events has involved patients undergoing cardiac surgery. The most commonly reported complication is tissue necrosis, an adverse event almost never seen after normal surgical procedures. Furthermore, consequences of these reported adverse events were typically severe and required intervention and additional medical and/or surgical treatment. Although these initial reports may be isolated incidents, they may also represent an early warning that is representative of a problem that is widespread. Nevertheless, these reports provide a potentially important signal, suggesting the need for further investigation into the relation between use of these infusion pumps for direct continuous infusion of local anesthetics and other drugs into surgical wounds and tissue necrosis, serious infections, or cellulitis. Neither of the two clinical investigations involving local anesthetic infusion in patients after cardiac surgery with median sternotomy reported such wound complications.30,31 Regardless, these safety issues merit careful consideration because of the importance of sternal wound complications in this setting.
The anterior and posterior branches of the intercostal nerves innervate the sternum. Parasternal infiltration of local anesthetic, therefore, is a possible means of improving postoperative analgesia. Although the use of parasternal blocks has not been extensively investigated, one small, prospective, randomized, placebo-controlled, double-blind clinical study indicated that parasternal block and local anesthetic infiltration of the sternotomy wound and mediastinal tube sites with local anesthetic may be a useful analgesic adjunct for patients who are expected to undergo early tracheal extubation after cardiac surgery.33
Nerve blocks
With the increasing popularity of minimally invasive cardiac surgery, which uses nonsternotomy incisions (minithoracotomy), the use of nerve blocks for the management of postoperative pain has increased as well34–39 (Box 38-4). Thoracotomy incisions (transverse anterolateral minithoracotomy, vertical anterolateral minithoracotomy), because of costal cartilage trauma tissue damage to ribs, muscles, or peripheral nerves, may induce more intense postoperative pain than that resulting from median sternotomy. Adequate analgesia after thoracotomy is important because pain is a key component in alteration of lung function after thoracic surgery. Uncontrolled pain causes a reduction in respiratory mechanics, reduced mobility, and increases in hormonal and metabolic activity. Perioperative deterioration in respiratory mechanics may lead to pulmonary complications and hypoxemia, which may, in turn, lead to myocardial ischemia/infarction, cerebrovascular accidents, thromboembolism, and delayed wound healing, leading to increased morbidity and prolonged hospital stay. Various analgesic techniques have been developed to treat postoperative thoracotomy pain. The most commonly used techniques include intercostal nerve blocks, intrapleural administration of local anesthetics, and thoracic paravertebral blocks. Intrathecal techniques and epidural techniques also are effective in controlling postthoracotomy pain and are covered in detail later in this chapter.
Intercostal nerve blocks have been used extensively for analgesia after thoracic surgery.34–36 They can be performed either intraoperatively or postoperatively and usually provide sufficient analgesia lasting approximately 6 to 12 hours (depending on amount and type of local anesthetic used) and may need to be repeated if additional analgesia is required. Local anesthetics may be administered as a single treatment under direct vision, before chest closure, as a single preoperative percutaneous injection, as multiple percutaneous serial injections, or via an indwelling intercostal catheter. Blockade of intercostal nerves interrupts C-fiber afferent transmission of impulses to the spinal cord. A single intercostal injection of a long-acting local anesthetic can provide pain relief and improve pulmonary function in patients after thoracic surgery for up to 6 hours. A continuous extrapleural intercostal nerve block technique may be used in which a catheter is placed percutaneously into an extrapleural pocket by the surgeon to achieve longer duration of analgesia. A continuous intercostal catheter allows frequent dosing or infusions of local anesthetic agents and avoids multiple needle injections. Various clinical studies have confirmed the analgesic efficacy of this technique, and the technique compares favorably with thoracic epidural analgesic techniques.34 A major concern associated with intercostal nerve block is the potentially high amount of local anesthetic systemic absorption, yet multiple clinical studies involving patients undergoing thoracic surgery have documented safe blood levels with standard techniques. Clinical investigations involving patients undergoing thoracic surgery indicate that intercostal nerve blockade by intermittent or continuous infusion of 0.5% bupivacaine with epinephrine is an effective method, as is continuous infusion of 0.25% bupivacaine through indwelling intercostal catheters for supplementing systemic intravenous opioid analgesia for postthoracotomy pain. The value of single preclosure injections remains doubtful.
Intrapleural administration of local anesthetics initiates analgesia via mechanisms that remain incompletely understood. However, the mechanism of action of extrapleural regional anesthesia seems to depend primarily on diffusion of the local anesthetic into the paravertebral region. Local anesthetic agents then affect not only the ventral nerve root but also afferent fibers of the posterior primary ramus. Posterior ligaments of the posterior primary ramus innervate posterior spinal muscles and skin and are traumatized during posterolateral thoracotomy. Intrapleural administration of local anesthetic agent to this region through a catheter inserted in the extrapleural space thus creates an anesthetic region in the skin. The depth and width of the anesthetic region depend on diffusion of the local anesthetic agent in the extrapleural space. With this technique, local anesthetics may be administered via an indwelling intrapleural catheter placed between the parietal and visceral pleura by intermittent or continuous infusion regimens. Concerns regarding systemic absorption of local anesthetic and toxicity are always a concern with this technique, yet have not been substantiated in clinical studies that assayed plasma levels. A handful of clinical investigations involving patients undergoing thoracic surgery via thoracotomy incision suggests that 0.25% to 0.5% bupivacaine may improve analgesia in patients after thoracic surgery, yet its true efficacy as a postoperative analgesic in this patient population remains somewhat controversial.37 The analgesic benefits are of short duration and there does not appear to be a significant overall opioid-sparing effect. Furthermore, the optimum concentration and duration regimen remains to be defined. However, a prospective, randomized, clinical study involving 50 patients undergoing minimally invasive direct CABG (via minithoracotomy) indicated that an intrapleural analgesic technique (with 0.25% bupivacaine) is safe, effective, and compares favorably (provided superior postoperative analgesia) with a conventional thoracic epidural technique.38 These investigators noted, however, that careful catheter positioning, chest tube clamping, and anchoring of the catheter are mandatory for postoperative intrapleural analgesia to be effective. A major factor implicated in lack of efficacy regarding intrapleural techniques is loss of local anesthetic solution through intercostal chest drainage tubes. Although clamping the chest tubes during the postoperative period will increase analgesic efficacy, it may not be safe to clamp chest tubes because they provide important drainage of hemorrhage and air and allow for enhanced lung patency and expansion. Apart from proper catheter positioning (insertion of catheter under direct vision and anchoring catheter to skin are essential), effective analgesia with this technique also appears to depend on whether lung surgery is performed or whether the pleural anatomy and physiology are relatively intact.
Thoracic paravertebral block involves injection of local anesthetic adjacent to the thoracic vertebrae close to where the spinal nerves emerge from the intervertebral foramina (Figure 38-2). Thoracic paravertebral block, compared with thoracic epidural analgesic techniques, appears to provide equivalent analgesia, is technically easier, and may harbor less risk. Several different techniques exist for successful thoracic paravertebral block and recently have been extensively reviewed.35 The classic technique, most commonly used, involves eliciting loss of resistance. Injection of local anesthetic results in ipsilateral somatic and sympathetic nerve blockade in multiple contiguous thoracic dermatomes above and below the site of injection (together with possible suppression of the neuroendocrine stress response to surgery). These blocks may be effective in alleviating acute and chronic pain of unilateral origin from the chest, abdomen, or both. Bilateral use of thoracic paravertebral block also has been described. Continuous thoracic paravertebral infusion of local anesthetic via a catheter placed under direct vision at thoracotomy is also a safe, simple, and effective method of providing analgesia after thoracotomy. It is usually used in conjunction with adjunct intravenous medications (opioid or other analgesics) to provide optimum relief after thoracotomy.
Although supplemental intravenous analgesics are usually required, opioid requirements are substantially reduced. Unilateral paravertebral block is useful for attaining post-thoracotomy analgesia because pain after lateral thoracotomy is essentially always unilateral. The role of bilateral thoracic paravertebral block remains to be defined. The benefits of unilateral paravertebral blockade are a lesser incidence of adverse events (hypotension, urinary retention) and a decreased risk for systemic local anesthetic toxicity because less local anesthetic is used. Few clinical investigations involve unilateral paravertebral block in patients undergoing thoracic surgery. Therefore, it is not possible to determine from the available literature whether the technique of paravertebral blockade (single injection) is truly useful in the postoperative analgesic management of patients after thoracotomy. However, continuous thoracic paravertebral block, as part of a balanced analgesic regimen, may provide effective pain relief with few adverse effects after thoracotomy and appears to be comparable with thoracic epidural analgesia.35
For a wide variety of reasons (increased use of small thoracic incisions by cardiac surgeons, etc.), the last decade has seen a resurgence of nerve blocks (usually catheter-based techniques) in patients undergoing cardiac surgery. Specifically, recent clinical studies using intercostal catheters,40 intrapleural catheters,41,42 and paravertebral blockade43,44 indicate that these techniques may have unique advantages, even when compared with traditional intrathecal/epidural techniques.45–47
Opioids
Beginning in the 1960s (and continuing for essentially 30 years), large doses of intravenous opioids (starting with morphine) have been administered to patients undergoing cardiac surgery48,49 (Box 38-5). Because even very large amounts of intravenous opioids do not initiate “complete anesthesia” (unconsciousness, muscle relaxation, suppression of reflex responses to noxious surgical stimuli), other intravenous/inhalation agents must be administered during the intraoperative period.50 Analgesia is the best known and most extensively investigated opioid effect, yet opioids also are involved in a diverse array of other physiologic functions, including control of pituitary and adrenal medulla hormone release and activity, control of cardiovascular and gastrointestinal function, and in the regulation of respiration, mood, appetite, thirst, cell growth, and the immune system.51 A number of well-known and potential side effects of opioids (nausea and vomiting, pruritus, urinary retention, respiratory depression) may limit postoperative recovery when they are used for postoperative analgesia.
Fentanyl is rapidly transferred across the blood–brain barrier, resulting in a rapid onset of action after intravenous injection. The relative potential for entering the CNS is approximately 150 times greater for fentanyl than for morphine. However, the large quantities of fentanyl taken up by adipose tissues may act as a reservoir (depending on dosage amounts) that slowly releases fentanyl back into the circulation when plasma concentrations decline to less than that in fat. This slow reentry may serve to maintain the plasma concentration and is one factor in the relatively long plasma terminal elimination half-life of fentanyl. Fentanyl is rapidly and extensively metabolized by the liver to inactive metabolites. After bolus intravenous injection, plasma fentanyl concentrations decrease rapidly because of distribution from the plasma to tissues, so that after moderate (10 μg/kg) doses, fentanyl has a short duration of action (see Chapter 9).
The popularity of fentanyl as an intraoperative analgesic agent relates directly to the cardiovascular stability it provides, even in critically ill patients. Also, its analgesic efficacy relative to the intensity of side effects has prompted much interest in its use as an analgesic after surgery and/or in critically ill patients.52 Fentanyl (as well as any opioid) can be administered intravenously for postoperative analgesia in many ways: using a loading dose with a continuous fixed or variable infusion, a fixed background infusion with PCA, or PCA alone. An intravenous bolus of 1 to 2 μg/kg usually is administered before initiating an infusion. If variable, the infusion rate is usually 1 to 2 μg/kg/hr and may be adjusted upward or downward as required by fluctuations in analgesic requirements or appearance of side effects. Before the infusion rate is increased, small intravenous bolus doses of fentanyl may be administered. Infusion rates of 1.5 to 2.5 μg/kg/hr usually provide good-to-excellent postoperative analgesia. At rest, the quality of analgesia remains stable; however, with movement, analgesia may not be sufficient, even with greater infusion rates.
The performance of a patient-demand, target-controlled alfentanil infusion system has compared favorably with traditional morphine PCA in patients after cardiac surgery.53 Checketts et al53 prospectively randomized 120 patients undergoing elective cardiac surgery to receive either morphine PCA or alfentanil PCA for postoperative analgesia (nonblinded). All patients received a similar standardized intraoperative anesthetic technique and were extubated during the immediate postoperative period. Overall median visual analog pain scores were significantly lower in patients receiving alfentanil, yet both alfentanil and morphine delivered high-quality postoperative analgesia (Figure 38-3). Although the clinical impression by these investigators was that alfentanil patients were less sedated in the immediate postoperative period, this clinical observation was not substantiated after statistical analysis of sedation scores. The two groups did not differ with respect to overall sedation scores, frequency of nausea and vomiting, hemodynamic instability, myocardial ischemia, or hypoxemia during the immediate postoperative period.
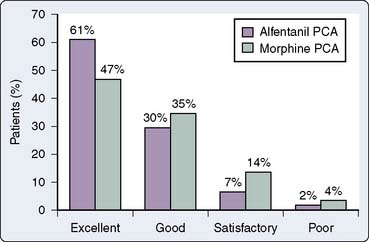
Figure 38-3 Overall patient satisfaction with postoperative analgesia.
(From Checketts MR, Gilhooly CJ, Kenny GNC: Patient-maintained analgesia with target-controlled alfentanil infusion after cardiac surgery: A comparison with morphine PCA. Br J Anaesth 80:748, 1998 ©The Board of Management and Trustees of the British Journal of Anaesthesia, by permission of Oxford University Press/British Journal of Anaesthesia.)
In 1996, Bowdle et al54 evaluated the use of a remifentanil infusion to provide postoperative analgesia during recovery from total intravenous anesthesia with remifentanil and propofol from a wide variety of noncardiac surgeries (abdominal, spine, joint replacement, thoracic surgery). This multi-institutional study involving 157 patients had a detailed protocol that specified doses and method of administration of all anesthetic drugs. In essence, total intraoperative intravenous anesthesia consisted of midazolam (premedication only), remifentanil, propofol, and vecuronium. Propofol was stopped immediately before intraoperative extubation, and the remifentanil infusion was continued for postoperative analgesia. During the immediate postoperative period, intravenous morphine was administered during tapering of remifentanil infusion. Adverse respiratory events (oxygen saturation via pulse oximetry < 90%, respiratory rate less than 12 per minute, apnea) affected 45 patients (29%, 2 required naloxone). Apnea occurred in 11 patients (7% treated with mask ventilation and downward titration of remifentanil infusion; 1 required naloxone). The administration of a bolus of remifentanil preceded the onset of adverse respiratory events in 19 of 45 cases and in 9 of 11 cases of apnea.
These data suggest that remifentanil boluses plus an infusion are particularly likely to produce clinically significant adverse respiratory events. The authors of this open, dose-ranging study concluded that although remifentanil certainly initiates analgesia, its use in the immediate postoperative period may pose dangers. Additional studies are needed to investigate the transition from remifentanil to longer-lasting analgesics and to refine strategies that minimize respiratory depression whereas optimizing pain control. The administration of a potent, rapidly acting opioid such as remifentanil by continuous infusion for postoperative analgesia must be performed with meticulous attention to detail and constant vigilance. Extreme caution should be exercised in the postoperative administration of bolus doses of remifentanil because substantial respiratory depression (including apnea) may develop. Furthermore, the remifentanil infusion should be inserted into the intravenous line as close as possible to the patient to minimize dead space, and the rate of the main intravenous infusion should be controlled at a rate that is high enough to continuously flush remifentanil from the tubing. A more dilute remifentanil solution that would run at greater rates (on a volume per time basis) would help to minimize the effect of variations in flow rate of the main intravenous tubing on delivery of remifentanil to the patient. Remifentanil also may possess detrimental cardiovascular effects via bradycardia and decreases in systemic vascular resistance, leading to decreased cardiac output and hypotension.55 Such changes may occur during clinically utilized doses for cardiac surgery (0.1 to 1.0 μg/kg/min), inducing significant cardiovascular disturbances that are potentially deleterious to patients with cardiac disease.55
Patient-controlled analgesia
When intravenous opioids are used for controlling postoperative pain (most commonly morphine and fentanyl), PCA technology generally is used. Essentials in the successful use of PCA technology include “loading” the patient with intravenous opioids to the point of patient comfort before initiating PCA, ensuring that the patient wants to control analgesic treatment, using an appropriate PCA dose and lockout interval, and considering the use of a basal rate infusion. Focused guidance of PCA dosing by a dedicated acute pain service, compared with surgeon-directed PCA, may result in more effective analgesia with fewer adverse effects. Patient-controlled epidural analgesic techniques, with opioids and/or local anesthetics, also have been proved reliable, effective, and safe.56,57
Although PCA is a well-established technique (used for more than two decades) and offers potential unique benefits (reliable analgesic effect, improved patient autonomy, flexible adjustment to individual needs, etc.), whether it truly offers significant clinical advantages (compared with traditional nurse-administrated analgesic techniques) to patients immediately after cardiac surgery remains to be determined.58–63 A clinical investigation by Gust et al59 indicated that PCA techniques provide a higher quality of postoperative analgesia, which may lead to a reduction in postoperative respiratory complications. In this prospective, randomized, clinical investigation involving 120 healthy patients after extubation after elective CABG, patients received either intravenous PCA piritramide, intravenous PCA piritramide plus rectal indomethacin, or conventional nurse-controlled analgesia with intravenous piritramide and/or rectal indomethacin for 3 days. Postoperative assessment included daily visual analog pain scoring and chest radiographs graded for the extent of atelectasis by a radiologist blinded to treatment. Perioperative management (surgical treatment, intraoperative anesthetic management) was standardized. Although chest radiography atelectasis scores and visual analog pain score values were similar among the three groups on the first and second postoperative days, on the third postoperative day, chest radiography atelectasis scores and visual analog pain score values were significantly better in the two PCA groups compared with the control (nurse-controlled analgesia) group.
At the end of the study, all patients retrospectively graded their postoperative pain management on average as good, but significantly more patients in the two PCA groups assessed their pain management as excellent compared with the control group. These investigators concluded that treatment with PCA may reduce respiratory complications in patients after CABG. However, no difference was observed regarding perioperative oxygenation values among the three groups during the entire study period, and not a single patient in any group met prospectively defined criteria for diagnosis of pneumonia. Other clinical investigations also have indicated that PCA techniques, compared with standard nurse-based pain therapy, may provide higher quality analgesia leading to reduced cardiopulmonary morbidity after cardiac surgery.60,63
Despite the popularity of PCA techniques and the results of the earlier quoted studies, other clinical investigations demonstrated no major benefits offered.58,61,62 Tsang and Brush58 prospectively evaluated 69 patients after cardiac surgery via median sternotomy. Thirty-nine were randomized to receive PCA morphine after surgery, whereas 30 were randomized to receive nurse-administered morphine after surgery. Perioperative care was standardized, visual analog pain scores were used for pain assessment, and pulmonary function tests were performed before surgery and every 6 hours after surgery until discharge from the ICU. These clinical investigators found no difference between the two groups regarding postoperative morphine consumption (Figure 38-4), postoperative visual analog pain scores, postoperative sedation scores, and postoperative pulmonary function (Figure 38-5). These investigators concluded that there are no significant advantages achieved when using PCA routinely in patients after cardiac surgery. Interestingly, in this study, opinions expressed by the nursing staff on the use of PCA were not as positive as expected (repetition of PCA instruction to patients was often required during the study period). Potential reasons for required repeated instructions on PCA include poor retention of preoperative learning because of anxiety after hospital admission, incomplete recovery of higher cognitive function after prolonged general anesthesia and CPB, and/or ICU-induced disorientation.
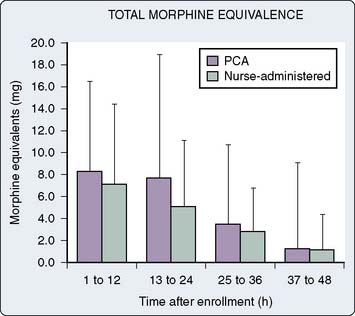
Figure 38-4 Total morphine equivalents.
(From Tsang J, Brush B: Patient-controlled analgesia in postoperative cardiac surgery. Anaesth Intensive Care 27:464, 1999.)
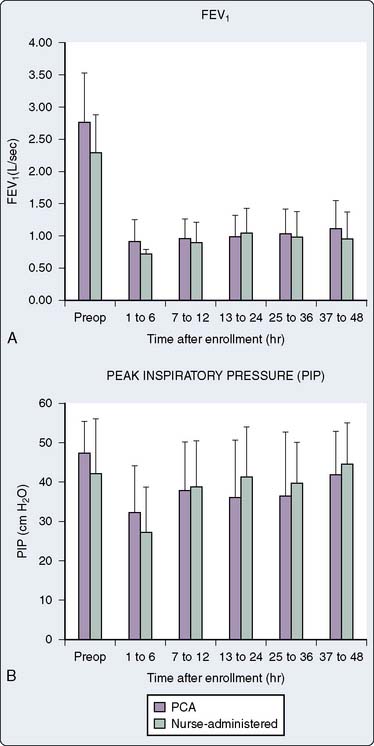
Figure 38-5 Postoperative pulmonary function.
(From Tsang J, Brush B: Patient-controlled analgesia in postoperative cardiac surgery. Anaesth Intensive Care 27:464, 1999.)
These results suggest that there may be additional patient limitations to the effective use of PCA immediately after cardiac surgery even though patients can obey simple commands and acknowledge discomfort. Munro and associates,61 when comparing intravenous PCA morphine and nurse-administered subcutaneous morphine, detailed similar findings. They prospectively randomized 92 patients undergoing elective cardiac surgery to receive either intravenous PCA morphine or nurse-administered subcutaneous morphine during the postoperative period. They found no differences between the two groups regarding many postoperative variables, including total postoperative morphine requirements, postoperative visual analog pain scores at rest and with movement, daily verbal pain relief scores, side effect profiles, and physiotherapist’s evaluation of effectiveness of analgesia for chest physiotherapy. Subcutaneous techniques are attractive because they have low equipment and disposable costs, eliminate the need for bulky pumps in ambulating patients, and may be more effective for the elderly or mildly confused postoperative patient.
Myles et al62 also were unable to find any specific clinical benefits during use of PCA techniques in patients after cardiac surgery. In their prospective clinical investigation, 72 patients undergoing elective cardiac surgery were randomized to receive either intravenous PCA morphine or intravenous nurse-titrated morphine during the immediate postoperative period. They found no differences between the two groups regarding many postoperative variables, including postoperative morphine consumption, postoperative pain scores, postoperative nausea scores, and postoperative serum cortisol levels (Figure 38-6). Much like Munro and associates, they noted that patients also had variable ability and understanding of the requirements of PCA, particularly in the early postoperative period when they were confused or too weak to operate the demand button. These investigators also noted that overall pain management in their patients was optimized by receiving experienced one-to-one nursing care (other studies evaluating PCA also have found that nurse-administered techniques may provide the highest quality analgesia). It could, therefore, be argued that these studies support increased staff education and involvement to optimize postoperative analgesia. The nurses in these clinical investigations all raised concerns regarding the time required for PCA setup and the inability of patients to cope with the demands of PCA in the early stages of their recovery, particularly if elderly, confused, or both. However, PCA was well received later in the recovery process and was found to be less demanding on nursing time.
Nonsteroidal anti-inflammatory agents
NSAIDs, in contrast with the opioids’ CNS mechanism of action, mainly exert their analgesic, antipyretic, and anti-inflammatory effects peripherally by interfering with prostaglandin synthesis after tissue injury64,65 (Box 38-6). NSAIDs inhibit COX, the enzyme responsible for the conversion of arachidonic acid to prostaglandin (Figure 38-7). Combining NSAIDs with traditional intravenous opioids may allow a patient to achieve an adequate level of analgesia with fewer side effects than if a similar level of analgesia was obtained with intravenous opioids alone. Numerous clinical investigations reveal the potential value (opioid-sparing effects) of NSAIDs when combined with traditional intravenous opioids during the postoperative period after noncardiac surgery. In fact, the administration of NSAIDs is one of the most common nonopioid analgesic techniques currently used for postoperative pain management. The efficacy of NSAIDs for postoperative pain has been demonstrated repeatedly in many analgesic clinical trials. Unlike opioids, which preferentially reduce spontaneous postoperative pain, NSAIDs have comparable efficacy for both spontaneous and movement-evoked pain, the latter of which may be more important in causing postoperative physiologic impairment. Certainly, NSAIDs reduce postoperative opioid consumption, accelerate postoperative recovery, and represent an integral component of balanced postoperative analgesic regimens after noncardiac surgery. However, little is known regarding NSAID use in the management of pain after cardiac surgery. It is likely that concerns regarding NSAID side effects, including alterations in the gastric mucosal barrier, renal tubular function, and inhibition of platelet aggregation, have made clinicians reluctant to use NSAIDs in patients undergoing cardiac surgery. Other rare adverse effects of NSAIDs (from COX inhibition) include hepatocellular injury, asthma exacerbation, anaphylactoid reactions, tinnitus, and urticaria. Despite these fears, a small number of clinical investigations seem to indicate that NSAIDs may provide analgesia in patients after cardiac surgery without untoward effects (gastrointestinal ulceration, renal dysfunction, excessive bleeding). Although NSAIDs have been associated with reports of increased postoperative blood loss, other studies have failed to corroborate this.
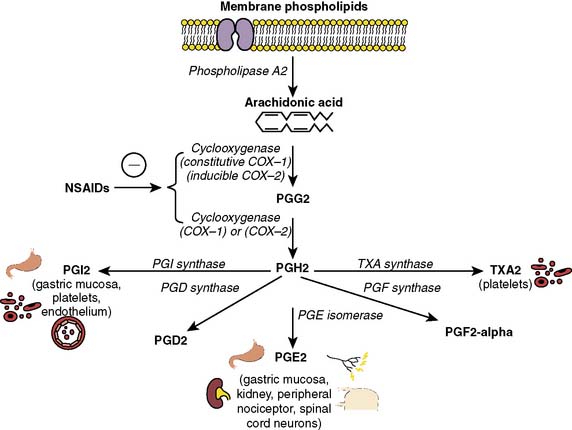
Figure 38-7 The role of cyclooxygenase in prostaglandin (PG) synthesis.
(From Gilron I, Milne B, Hong M: Cyclooxygenase-2 inhibitors in postoperative pain management: Current evidence and future directions [review article]. Anesthesiology 99:1198, 2003.)
NSAIDs are not a homogenous group and vary considerably in analgesic efficacy as a result of differences in pharmacodynamic and pharmacokinetic parameters. NSAIDs are nonspecific inhibitors of COX, which is the rate-limiting enzyme involved in the synthesis of prostaglandins. A major scientific discovery revealed that COX exists in multiple forms. Most important, a constitutive form is present in normal conditions in healthy cells (COX-1) and an inducible form (COX-2) exists, which is the major isozyme induced by and associated with inflammation. Simplistically, COX-1 is ubiquitously and constitutively expressed, and has a homeostatic role in platelet aggregation, gastrointestinal mucosal integrity, and renal function, whereas COX-2 is inducible and expressed mainly at sites of injury (and kidney and brain) and mediates pain and inflammation. NSAIDs are nonspecific inhibitors of both forms of COX, yet vary in their ratio of COX-1 to COX-2 inhibition. Recent molecular studies distinguishing between constitutive COX-1 and inflammation-inducible COX-2 enzymes have led to the exciting hypothesis that the therapeutic and adverse effects of NSAIDs could be uncoupled66–71 (Figure 38-8