CHAPTER 301 Posterior Thoracic Instrumentation
Anatomy and Biomechanics
The musculature overlying the dorsal surface of the thoracic spine contributes to the maintenance of sagittal and coronal balance and aids in controlling rotation. The dorsal musculature can be roughly divided into superficial and deep layers. Proper exposure for placement of thoracic instrumentation often requires visualization of the facets, transverse processes, and sometimes the ribs through extensive, careful muscle dissection. Using fascial planes and subperiosteal dissection reduces blood loss, postoperative pain, and wound complications. Rotation and transposition of muscle flaps can also be a useful adjunct to provide coverage for spinal hardware and promote wound healing in patients with poor healing potential, previous radiation, or revision surgery.1
It cannot be overstated that there is considerable individual variability with regard to the dimensions and angulation of the thoracic pedicles, making it generally advisable to assess pedicular anatomy with preoperative radiographic evaluation before attempting transpedicular fixation.2–4
In general, the pedicular dimensions are larger in men.5,6 The width of the pedicle decreases from T1 to T4 and then gradually increases to T12, whereas the pedicle height and length tend to increase from T1 to T12. The transverse angle of the pedicle, however, decreases from T1 to T12, such that the pedicles become less medially inclined the closer they are to the thoracolumbar junction. At T1 and T2, the pedicles have a medial projection of 30 to 40 degrees; at T3 to T11, this transverse angle is about 20 to 25 degrees; and at T12, the transverse angle is closer to 10 degrees (Fig. 301-1).3–8 Conversely, the pedicular sagittal angle remains consistent throughout the thoracic spine (downward projection of about 10 to 20 degrees) (see Fig. 301-1D). Most of the pedicle is composed of cancellous bone, which is encased in a shell of cortical bone, that is significantly thicker medially than laterally. This may account for the finding that most screw-related pedicle fractures occur laterally.9,10
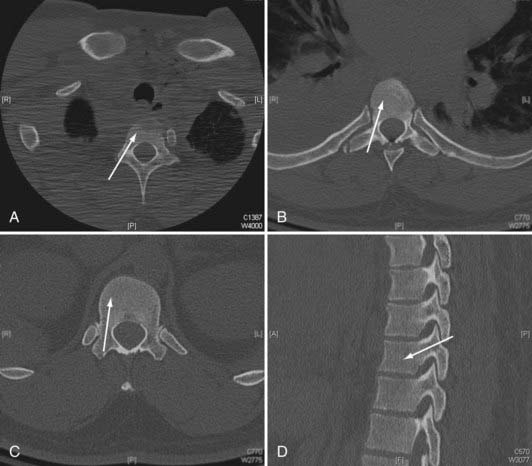
FIGURE 301-1 Axial plane pedicle angulation at T1 (A), T7 (B), and T12 (C). D, Representative sagittal pedicle angulation.
The thecal sac abuts the medial wall of the pedicle, and the nerve root exits under the inferior wall of the same numbered pedicle. The nerve roots tend to have a more cephalad angulation and a closer relationship to the pedicle in the upper thoracic spine and a more caudal angulation with a greater distance from the pedicle in the lower thoracic spine.5 The thoracic facets are oriented in a coronal plane throughout most of the thoracic spine (T1 to T10). This orientation provides stability against anteroposterior translation. In the lower thoracic spine, the facets take on a more sagittal orientation, providing more stability against rotation. The spinous processes project inferiorly in the upper and middle thoracic spine but have a more horizontal configuration in the lower thoracic spine.
The spinal ligaments and joint capsules preserve the articulated nature of the spine, allowing a requisite but restrained amount of movement. The anterior longitudinal ligament contains a relatively higher proportion of collagen and acts to prevent hyperextension and overdistraction.11 Testing has found that the strength of the anterior longitudinal ligament increases as it descends toward the thoracolumbar junction.12 The posterior longitudinal ligament functions to limit hyperflexion of the spine. Its tensile strength is about half that of the anterior longitudinal ligament. The integrity of the posterior longitudinal ligament is essential when attempting to perform indirect reductions of fractures through ligamentotaxis with posterior instrumentation.13
Biomechanics
Whitesides defined a stable spine as one that possesses sufficient integrity to protect the neural elements from initial or subsequent damage and prevent the development of incapacitation deformity and severe pain under physiologic loads.14 Proper instrumentation warrants a thorough understanding of the unique biomechanical considerations in the thoracic region. In general, the thoracic spine is a relatively less mobile segment positioned between two regions of relatively abrupt motion transition zones at the cervicothoracic and thoracolumbar junctions. This transition between relative mobility and immobility makes cervicothoracic and thoracolumbar junctions more susceptible to traumatic injury and destabilization. The thoracic spine has a normal kyphosis over its length (20% to 45%, increases with age) but is relatively neutral across the thoracolumbar junction.11,15 This kyphosis makes the thoracic spine more susceptible to sagittal imbalance and instability. This kyphosis is formed in utero and is maintained by the differences in the anterior and posterior vertebral bodies and disk dimensions. The height of the ventral surface of the thoracic vertebral body is about 1 to 2 mm less than that of the dorsal surface. This slight height difference is also seen in the thoracic intervertebral disks.16
Similar to other regions of the spine, the vertebral bodies support most of the axial loading forces, and the intervertebral disk complexes are major stabilizers of the thoracic spine. Additionally, the steep orientation of the facets limits sagittal translation. However, the rib cage and sternum provide significant inherent stability to the thoracic spine in flexion-extension, lateral bending, and axial rotation.17 Sternal fractures have been shown to destabilize the thorax and have a strong association with thoracic spine fractures.17–19 The rib head joints provide stabilization in the sagittal, coronal, and transverse planes. The combination of diskectomy and rib head resection introduces significant mobility to a thoracic spinal segment.20
There are several key biomechanical questions that need to be addressed when planning thoracic instrumentation. Very often the question pertains to number of instrumented levels. There has to be a balance between minimizing the number of involved segments (to reduce blood loss; case length; risk for neural, vascular, and visceral injury; lost motion segments; cost), and providing sufficient stabilization to allow healing. This decision must take into account the intended biomechanical function of the posterior instrumentation: maintain deformity correction and alignment until the anterior column heals, neutralize rotation-translation, or perhaps supplement an anterior reconstruction. The integrity or healing capacity of the anterior column, bone quality, surgical anatomy (adequate pedicular dimensions and structural integrity), and relationship to thoracolumbar or cervicothoracic junction must also considered. Posterior-only fusion for thoracolumbar fracture assumes eventual healing of the anterior column. Decisions about the length of the construct and the need for anterior reconstruction in thoracolumbar fractures have been addressed, for example, by the load-sharing classification of McCormack and colleagues.21
Techniques for Posterior Thoracic Internal Fixation
Spinal instrumentation has undergone rapid modification in the past 30 years. There has been a trend toward pedicle screw–based rigid construction in the treatment of a variety of spinal pathologies. This is certainly vastly different from the approaches of the past, including Harrington rod-hook constructs and wiring techniques. Eduardo Luque described a technique of rigid internal fixation using parallel paraspinal bars secured to the spine with multilevel bilateral sublaminar wires.22 The technique was modified to involve use of a rectangular device instead of two separate bars (Hartshill-Luque rectangle) as well as multistrand wires (Songer cables), which simplified placement and increased strength and stability.23–26 This technique was used initially to treat scoliosis and was subsequently applied to other spinal pathologies, including stabilization for degenerative disease, tumor resection, and trauma.27–35 The less rigid nature of the Hartshill-Luque system comes from the fact that the wires are not solidly attached to the rods. Therefore, distraction and compression forces cannot be applied. It is better at preventing sagittal flexion-extension as opposed to rotational forces,36,37 and some kyphosis correction is possible with this technique. Hook-based constructs, although not as rigid or as versatile for performing deformity correction, still are based on fixation of the hooks solidly to the rods. The use of laminar, transverse process, and pedicle hooks provides constructs that can better control axial loading in comparison to sublaminar wires. However, hooks require intact lamina, pedicles, or transverse process and have significantly less pullout strength than pedicle screws except in cases of osteoporosis. When treating patients with osteoporosis, hooks may provide rigidity comparable to that of pedicle screw constructs unless the screws are augmented with bone cement.38,39 First used in the correction of scoliotic deformities, laminar hooks can be placed around either the superior or inferior portion of the lamina, depending on the configuration needed. Distraction or compression can therefore be achieved to correct the deformity and provide stability. The insertion of laminar, transverse process, and pedicle hooks is technically less demanding and carries less of a risk for iatrogenic injury than does pedicle screw insertion, especially in the upper thoracic spine where the pedicles are relatively smaller. However, hooks do require placement of hardware in the spinal canal.
Variations in pedicle anatomy sometimes require the use of screws and hooks in combination, further increasing versatility. Hooks can serve as cephalad points of attachment of a construct that uses pedicle screws at its more caudal end (Fig. 301-2). Offset laminar hooks may also be used in conjunction with pedicle screws to augment the stiffness of the construct. Combination techniques can be especially useful at the cervicothoracic junction, where the pathology can be very challenging. Combining cervical lateral mass and possibly C7 pedicle screws with upper thoracic pedicle screws or hooks provides excellent versatility and stability at the cervicothoracic junction (Fig. 301-3).
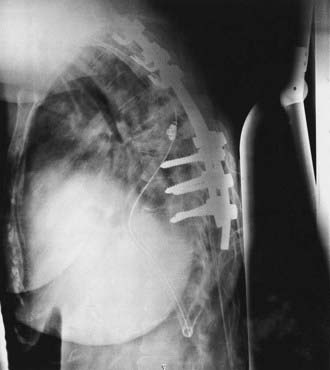
FIGURE 301-2 Lateral radiograph showing thoracic fusion with caudal pedicle screws and cephalad laminar-pedicle hook construct.
Despite the increased level of technical difficulty associated with placement, pedicle screws provide several distinct advantages over other fixation techniques. From a biomechanical standpoint, pedicle screw–based spinal instrumentation provides the most rigid constructs and also allows versatility with corrections, including the ability to apply distraction and compression and intraoperative manipulation for correction of deformity.40–42 Pedicle screw fusion also decreases the number of adjacent segments that need to be incorporated to obtain stability compared with wire and hook systems.7 Pedicle screws can be placed at levels where there have been wide laminectomies, lamina fractures, or even partial pedicle removal. Additionally, pedicle screws do not require placement of implants in the spinal canal, as is the case with hooks and sublaminar wires.
Numerous descriptions of the appropriate starting point and angles of insertion for thoracic pedicle screws exist.3,8,43–47 Individual surgical technique often represents modifications of these descriptions based on previous training and experience, resulting in an amalgam of numerous techniques. The spectrum varies from “freehand” techniques based entirely on external anatomic landmarks, to adjuncts including fluoroscopy, use of laminotomies, and direct palpation of the pedicles, to various types of intraoperative frameless navigation systems, including systems based on imaging intraoperatively that allow tomographic verification of screw placement. Literature support exists for all these possibilities alone or in combination.44,48–50 Ultimately, the decision concerning the method for screw insertion is based on previous experience and training, accessibility to radiographic adjuncts, and comfort level of the individual surgeons.
Proper pedicle screw insertion requires the surgeon to navigate the pedicle projections in three dimensions. Because of individual variability and pathology, locating starting points and trajectory angles based only on published techniques can lead to inaccuracies. They can be used as general guidelines but must be modified by radiographic examination and intraoperative observation.3,6,8,43,45,50–54
Complications associated with thoracic pedicle screw insertion include nerve root, spinal cord, visceral, and vascular injury. An improperly placed screw in the sagittal plane may injure a nerve root. Esses and coworkers’ series of 617 cases of pedicle screw fixation contained 169 complications, 29 of which were nerve root injury.55 Matsuzaki and colleagues series of 57 cases had 6 patients with postoperative radiculopathies.56 Even in the face of spinal deformity, thoracic transpedicular fixation may be used safely. In Suk and associates’ series of 462 patients with spinal deformity, only 1 patient suffered transient neurological paraparesis from a delayed epidural hematoma.47
Several methods have been used to increase the safety of pedicle screw placement, including fluoroscopy, image guidance, direct palpation of the pedicle through laminotomies, and electrophysiologic monitoring. Electrophysiologic monitoring has been used with some success to assess the placement of lumbar pedicle screws. A screw placed completely within the pedicle requires higher voltage stimulation to achieve an electromyographic limb response than does a screw placed with a breach in the pedicle wall. However, threshold values to identify a misplaced pedicle screw in the thoracic spine are less well defined.57,58
Image-guidance techniques are being used more frequently for the placement of pedicle screws to improve accuracy and safety. Newer techniques using intraoperative scan acquisition after positioning, in combination with image-guided navigation, allow reregistration if necessary and final CT scans to verify hardware placement. These techniques are especially useful in combination with minimally invasive techniques (Fig. 301-4). Although their full utility has yet to be realized, there are several potential drawbacks. These systems are not foolproof, and as with intracranial image guidance, they can provide misinformation. Also, if a surgeon’s experience involves placement only with image guidance, he or she may not recognize an aberrant trajectory. Finally, image guidance can potentially add additional time to the case.
Anterior Column Reconstruction Through A Posterior Approach
Although subsequent posterior instrumentation has been traditionally used to augment anterior reconstruction in complex spinal pathologies (tumor, infection, trauma, deformity), it is possible to simultaneously address both anterior and posterior decompression and stabilization through a one-stage posterior approach. These approaches generally involve some variation of an extracavitary or costotransversectomy approach with some form of anterior column reconstruction and posterior stabilization. Various methods of anterior reconstruction have been used, including polymethyl methacrylate (PMMA), fixed-length metal cages, and bone grafts. The development of expandable cages for reconstruction has greatly enriched the versatility of this technique. The distinct advantage of this technique is that it avoids the morbidity of an anterior transthoracic approach. Although the anterior transthoracic approach provides the most direct route for pathology in the vertebral body, allowing for minimal removal of uninvolved bone,59 these anterior approaches can be associated with significant morbidity. For example, anterior decompression and stabilization has been associated with complications in 21% to 46% of cases, including intraoperative organ and vessel injury, excessive hemorrhage, wound breakdown, cerebrospinal fluid leak, and postoperative respiratory failure.59,60 Additionally, the anterior approach for complete decompression and spinal reconstruction for upper thoracic tumors can be especially difficult.61–63 Alternatively, the posterolateral transpedicular approach avoids many of the cardiopulmonary complications seen in the anterior approach.27,64–66 Wang and associates reported a 14% complication rate for the posterolateral transpedicular approach with most being wound dehiscence or infection.67 In our experience, wound difficulties increase after radiation and incisions in the upper thoracic spine.
The anterior column can be reconstructed with a number of materials, including bone grafts, PMMA, struts with or without Steinmann pins, and metal cages. Titanium cages (fixed and expandable) have been used extensively as anterior fixation devices after corpectomy from anterior approaches. They have been shown to improve spinal alignment and decrease pain.66 Expandable cages are ideal for posterolateral placement. Their broad end plates provide solid anterior support to the adjacent vertebral end plates. In situ cephalocaudal cage expansion can also help reduce pathologic kyphosis.68 The exothermic PMMA setting process is avoided, eliminating the risk for thermal injury to surrounding structures, including the spinal cord. Insertion and positioning require few steps and can be accomplished quickly. Given the structural stability of the cage as an anterior fixation device, the number of levels included in posterior fixation can be reduced. This technique can be used to address a wide range of pathologies, including tumor, infection, and trauma (Figs. 301-5 and 301-6).
Surgical Technique
Generally, the patients are placed prone on a radiolucent table. A midline incision is made and the dissection carried out over the costotransverse junction to expose the ribs at the affected levels. Laminectomies are generally performed at the affected level. The pedicle screws are placed in a standard fashion, with placement of a unilateral temporary rod before beginning the anterior dissection. This is done so that if bleeding is excessive or the patient becomes hemodynamically unstable, the posterior fusion can be performed in an expedient fashion. Subsequently, a portion of the rib extending beyond the transverse process is removed at the involved levels, the pedicle is drilled down to the vertebral body, and the affected body is removed (Fig. 301-7). Particular attention is made to identify the superior and inferior end plates when possible because drilling into the end plate of an adjacent vertebral body can weaken the end plate and promote subsidence of the cage into that body.
The bone removal is most often performed bilaterally (often more extensively on the side of cage insertion) to minimize traction on the cord, maximize resection, and ensure that the cage is near the center of the vertebral body (see Fig. 301-7). A thoracic nerve root (from T2 to T12) is generally sacrificed by suture ligation on the side of the cage insertion, at the level of the corpectomy, to facilitate placement. This usually results in radicular numbness along the rib at the same level. Bilateral decompression can be accomplished by working around the nerve root on the side opposite cage insertion. This typically avoids bilateral takedown of radicular arteries. This is important, especially for multilevel corpectomies, to avoid potential cord ischemia.
Bilateral bone decompression facilitates kyphosis correction because the spine can be completely disarticulated. Significant kyphosis correction is accomplished before placing the cage by placing a rod on one side to maintain reduction. The cage is then inserted from a posterolateral approach and expanded to complete the anterior column reconstruction (see Fig. 301-7). Finally, the remaining posterior hardware is placed, and either autograft or allograft bone is placed around the cage and in the lateral gutters after decortication (see Fig. 301-7).
An HS, Singh K, Vaccaro AR, et al. Biomechanical evaluation of contemporary posterior spinal internal fixation configurations in an unstable burst-fracture calf spine model: special references of hook configurations and pedicle screws. Spine. 2004;29:257.
An HS, Vaccaro A, Cotler JM, et al. Spinal disorders at the cervicothoracic junction. Spine. 1994;19:2557.
Bilsky MH, Boland P, Lis E, et al. Single-stage posterolateral transpedicle approach for spondylectomy, epidural decompression, and circumferential fusion of spinal metastases. Spine. 2000;25:2240.
Ebraheim NA, Jabaly G, Xu R, et al. Anatomic relations of the thoracic pedicle to the adjacent neural structures. Spine. 1997;22:1553.
Ebraheim NA, Xu R, Ahmad M, et al. Projection of the thoracic pedicle and its morphometric analysis. Spine. 1997;22:233.
Esses SI, Sachs BL, Dreyzin V. Complications associated with the technique of pedicle screw fixation. A selected survey of ABS members. Spine. 1993;18:2231.
Gokaslan ZL, York JE, Walsh GL, et al. Transthoracic vertebrectomy for metastatic spinal tumors. J Neurosurg. 1998;89:599.
Hitchon PW, Brenton MD, Black AG, et al. In vitro biomechanical comparison of pedicle screws, sublaminar hooks, and sublaminar cables. J Neurosurg. 2003;99:104.
Hunt T, Shen FH, Arlet V. Expandable cage placement via a posterolateral approach in lumbar spine reconstructions. Technical note. J Neurosurg Spine. 2006;5:271.
Kim YJ, Lenke LG, Bridwell KH, et al. Free hand pedicle screw placement in the thoracic spine: is it safe? Spine. 2004;29:333.
McCormack T, Karaikovic E, Gaines RW. The load sharing classification of spine fractures. Spine. 1994;19:1741.
Schizas C, Theumann N, Kosmopoulos V. Inserting pedicle screws in the upper thoracic spine without the use of fluoroscopy or image guidance. Is it safe? Eur Spine J. 2007;16:625.
Shen FH, Marks I, Shaffrey C, et al. The use of an expandable cage for corpectomy reconstruction of vertebral body tumors through a posterior extracavitary approach: a multicenter consecutive case series of prospectively followed patients. Spine J. 2008;8:329.
Ugur HC, Attar A, Uz A, et al. Thoracic pedicle: surgical anatomic evaluation and relations. J Spinal Disord. 2001;14:39.
Vaccaro AR, Rizzolo SJ, Allardyce TJ, et al. Placement of pedicle screws in the thoracic spine. Part I: Morphometric analysis of the thoracic vertebrae. J Bone Joint Surg Am. 1995;77:1193.
Vaccaro AR, Rizzolo SJ, Balderston RA, et al. Placement of pedicle screws in the thoracic spine. Part II: An anatomical and radiographic assessment. J Bone Joint Surg Am. 1995;77:1200.
Whang PG, Vaccaro AR. Thoracolumbar fracture: posterior instrumentation using distraction and ligamentotaxis reduction. J Am Acad Orthop Surg. 2007;15:695.
White AA, Punjabe M. Clinical Biomechanics of the Spine, 3rd ed. Philadelphia: JB Lippincott; 1990.
Whitesides TEJr. Traumatic kyphosis of the thoracolumbar spine. Clin Orthop Relat Res. 1977;128:78-92.
Xu R, Ebraheim NA, Shepherd ME, et al. Thoracic pedicle screw placement guided by computed tomographic measurements. J Spinal Disord. 1999;12:222.
1 Vitaz TW, Oishi M, Welch WC, et al. Rotational and transpositional flaps for the treatment of spinal wound dehiscence and infections in patient populations with degenerative and oncological disease. J Neurosurg. 2004;100:46.
2 Ugur HC, Attar A, Uz A, et al. Thoracic pedicle: surgical anatomic evaluation and relations. J Spinal Disord. 2001;14:39.
3 Vaccaro AR, Rizzolo SJ, Allardyce TJ, et al. Placement of pedicle screws in the thoracic spine. Part I: Morphometric analysis of the thoracic vertebrae. J Bone Joint Surg Am. 1995;77:1193.
4 Vaccaro AR, Rizzolo SJ, Balderston RA, et al. Placement of pedicle screws in the thoracic spine. Part II: An anatomical and radiographic assessment. J Bone Joint Surg Am. 1995;77:1200.
5 Ebraheim NA, Jabaly G, Xu R, et al. Anatomic relations of the thoracic pedicle to the adjacent neural structures. Spine. 1997;22:1553.
6 Ebraheim NA, Xu R, Ahmad M, et al. Projection of the thoracic pedicle and its morphometric analysis. Spine. 1997;22:233.
7 An HS, Singh K, Vaccaro AR, et al. Biomechanical evaluation of contemporary posterior spinal internal fixation configurations in an unstable burst-fracture calf spine model: special references of hook configurations and pedicle screws. Spine. 2004;29:257.
8 Xu R, Ebraheim NA, Shepherd ME, et al. Thoracic pedicle screw placement guided by computed tomographic measurements. J Spinal Disord. 1999;12:222.
9 Kothe R, O’Holleran JD, Liu W, et al. Internal architecture of the thoracic pedicle. An anatomic study. Spine. 1996;21:264.
10 Panjabi MM, O’Holleran JD, Crisco JJ3rd, et al. Complexity of the thoracic spine pedicle anatomy. Eur Spine J. 1997;6:19.
11 White AA, Punjabe M. Clinical Biomechanics of the Spine, 3rd ed. Philadelphia: JB Lippincott; 1990.
12 Myklebust JB, Pintar F, Yoganandan N, et al. Tensile strength of spinal ligaments. Spine. 1988;13:526.
13 Whang PG, Vaccaro AR. Thoracolumbar fracture: posterior instrumentation using distraction and ligamentotaxis reduction. J Am Acad Orthop Surg. 2007;15:695.
14 Whitesides TEJr. Traumatic kyphosis of the thoracolumbar spine. Clin Orthop Relat Res. 1977;128:78-92.
15 Fon GT, Pitt MJ, Thies ACJr. Thoracic kyphosis: range in normal subjects. AJR Am J Roentgenol. 1980;134:979.
16 Maiman DJ, Pintar FA. Anatomy and clinical biomechanics of the thoracic spine. Clin Neurosurg. 1992;38:296.
17 Watkins RT, Watkins R3rd, Williams L, et al. Stability provided by the sternum and rib cage in the thoracic spine. Spine. 2005;30:1283.
18 Oda I, Abumi K, Cunningham BW, et al. An in vitro human cadaveric study investigating the biomechanical properties of the thoracic spine. Spine. 2002;27:E64.
19 Oda I, Abumi K, Lu D, et al. Biomechanical role of the posterior elements, costovertebral joints, and rib cage in the stability of the thoracic spine. Spine. 1996;21:1423.
20 Takeuchi T, Abumi K, Shono Y, et al. Biomechanical role of the intervertebral disc and costovertebral joint in stability of the thoracic spine. A canine model study. Spine. 1999;24:1414.
21 McCormack T, Karaikovic E, Gaines RW. The load sharing classification of spine fractures. Spine. 1994;19:1741.
22 Gau YL, Lonstein JE, Winter RB, et al. Luque-Galveston procedure for correction and stabilization of neuromuscular scoliosis and pelvic obliquity: a review of 68 patients. J Spinal Disord. 1991;4:399.
23 Calista F, Gualtieri I, Conti P, et al. The results of the surgical treatment of 64 patients with thoracic and lumbar fracture. Chir Organi Mov. 2002;87:109.
24 Christodoulou AG, Kapetanos G, Apostolou T, et al. Segmental spinal correction of idiopathic scoliosis. Luque rods and Hartshill rectangle in 30 patients followed for 2-6 years. Acta Orthop Scand Suppl. 1997;275:3.
25 Parsons JR, Chokshi BV, Lee CK, et al. The biomechanical analysis of sublaminar wires and cables using Luque segmental spinal instrumentation. Spine. 1997;22:267.
26 Songer MN, Spencer DL, Meyer PRJr, et al. The use of sublaminar cables to replace Luque wires. Spine. 1991;16:S418.
27 Akeyson EW, McCutcheon IE. Single-stage posterior vertebrectomy and replacement combined with posterior instrumentation for spinal metastasis. J Neurosurg. 1996;85:211.
28 Bridwell KH. Treatment of a markedly displaced hangman’s fracture with a Luque rectangle and a posterior fusion in a 71-year-old man. Case report. Spine. 1986;11:49.
29 Chang CJ, Huang JS, Wang YC, et al. Intraosseous schwannoma of the fourth lumbar vertebra: case report. Neurosurgery. 1998;43:1219.
30 Cybulski GR, Nijensohn E, Brody BA, et al. Spinal cord compression from a thoracic paraganglioma: case report. Neurosurgery. 1991;28:306.
31 MacKenzie AI, Uttley D, Marsh HT, et al. Craniocervical stabilization using Luque/Hartshill rectangles. Neurosurgery. 1990;26:32.
32 Maurer PK, Ellenbogen RG, Ecklund J, et al. Cervical spondylotic myelopathy: treatment with posterior decompression and Luque rectangle bone fusion. Neurosurgery. 1991;28:680.
33 Sutterlin CE3rd, Bianchi JR, Kunz DN, et al. Biomechanical evaluation of occipitocervical fixation devices. J Spinal Disord. 2001;14:185.
34 Sutterlin CE3rd, McAfee PC, Warden KE, et al. A biomechanical evaluation of cervical spinal stabilization methods in a bovine model. Static and cyclical loading. Spine. 1988;13:795.
35 Ward P, Harvey AR, Ramos J, et al. Hartshill rectangle: failure of spinal stabilisation in acute spinal cord injury. Eur Spine J. 2000;9:152.
36 Davies AG, McMaster MJ. The effect of Luque-rod instrumentation on the sagittal contour of the lumbosacral spine in adolescent idiopathic scoliosis and the preservation of a physiologic lumbar lordosis. Spine. 1992;17:112.
37 Gaines RWJr, Carson WL, Satterlee CC, et al. Experimental evaluation of seven different spinal fracture internal fixation devices using nonfailure stability testing. The load-sharing and unstable-mechanism concepts. Spine. 1991;16:902.
38 Coe JD, Warden KE, Herzig MA, et al. Influence of bone mineral density on the fixation of thoracolumbar implants. A comparative study of transpedicular screws, laminar hooks, and spinous process wires. Spine. 1990;15:902.
39 Burval DJ, McLain RF, Milks R, et al. Primary pedicle screw augmentation in osteoporotic lumbar vertebrae: biomechanical analysis of pedicle fixation strength. Spine. 2007;32:1077.
40 Gurr KR, McAfee PC, Shih CM. Biomechanical analysis of anterior and posterior instrumentation systems after corpectomy. A calf-spine model. J Bone Joint Surg Am. 1988;70:1182.
41 Gurr KR, McAfee PC, Shih CM. Biomechanical analysis of posterior instrumentation systems after decompressive laminectomy. An unstable calf-spine model. J Bone Joint Surg Am. 1988;70:680.
42 Hitchon PW, Brenton MD, Black AG, et al. In vitro biomechanical comparison of pedicle screws, sublaminar hooks, and sublaminar cables. J Neurosurg. 2003;99:104.
43 Esses SI, Bednar DA. The spinal pedicle screw: techniques and systems. Orthop Rev. 1989;18:676.
44 Karapinar L, Erel N, Ozturk H, et al. Pedicle screw placement with a free hand technique in thoracolumbar spine: is it safe? J Spinal Disord Tech. 2008;21:63.
45 Kim YJ, Lenke LG, Bridwell KH, et al. Free hand pedicle screw placement in the thoracic spine: is it safe? Spine. 2004;29:333.
46 Myles RT, Fong B, Esses SI, et al. Radiographic verification of pedicle screw pilot hole placement using Kirschner wires versus beaded wires. Spine. 1999;24:476.
47 Suk SI, Kim WJ, Lee SM, et al. Thoracic pedicle screw fixation in spinal deformities: are they really safe? Spine. 2001;26:2049.
48 Cho KJ, Suk SI, Park SR, et al. Short fusion versus long fusion for degenerative lumbar scoliosis. Eur Spine J. 2008;17:650.
49 Modi H, Suh SW, Song HR, et al. Accuracy of thoracic pedicle screw placement in scoliosis using the ideal pedicle entry point during the freehand technique. Int Orthop. 2009;33:469-475.
50 Schizas C, Theumann N, Kosmopoulos V. Inserting pedicle screws in the upper thoracic spine without the use of fluoroscopy or image guidance. Is it safe? Eur Spine J. 2007;16:625.
51 Daubs MD, Kim YJ, Lenke LG. Pedicle screw fixation (T1, T2, and T3). Instr Course Lect. 2007;56:247.
52 Kim YJ, Lenke LG. Thoracic pedicle screw placement: free-hand technique. Neurol India. 2005;53:512.
53 Roy-Camille R, Saillant G, Mazel C. Plating of thoracic, thoracolumbar, and lumbar injuries with pedicle screw plates. Orthop Clin North Am. 1986;17:147.
54 Suk SI, Lee CK, Min HJ, et al. Comparison of Cotrel-Dubousset pedicle screws and hooks in the treatment of idiopathic scoliosis. Int Orthop. 1994;18:341.
55 Esses SI, Sachs BL, Dreyzin V. Complications associated with the technique of pedicle screw fixation. A selected survey of ABS members. Spine. 1993;18:2231.
56 Matsuzaki H, Tokuhashi Y, Matsumoto F, et al. Problems and solutions of pedicle screw plate fixation of lumbar spine. Spine. 1990;15:1159.
57 Raynor BL, Lenke LG, Kim Y, et al. Can triggered electromyograph thresholds predict safe thoracic pedicle screw placement? Spine. 2002;27:2030.
58 Lewis SJ, Lenke LG, Raynor B, et al. Triggered electromyographic threshold for accuracy of thoracic pedicle screw placement in a porcine model. Spine. 2001;26:2485.
59 Gokaslan ZL, York JE, Walsh GL, et al. Transthoracic vertebrectomy for metastatic spinal tumors. J Neurosurg. 1998;89:599.
60 Lewandrowski KU, Hecht AC, DeLaney TF, et al. Anterior spinal arthrodesis with structural cortical allografts and instrumentation for spine tumor surgery. Spine. 2004;29:1150.
61 An HS, Vaccaro A, Cotler JM, et al. Spinal disorders at the cervicothoracic junction. Spine. 1994;19:2557.
62 Mazel C, Grunenwald D, Laudrin P, et al. Radical excision in the management of thoracic and cervicothoracic tumors involving the spine: results in a series of 36 cases. Spine. 2003;28:782.
63 Mazel C, Hoffmann E, Antonietti P, et al. Posterior cervicothoracic instrumentation in spine tumors. Spine. 2004;29:1246.
64 Bilsky MH, Boland P, Lis E, et al. Single-stage posterolateral transpedicle approach for spondylectomy, epidural decompression, and circumferential fusion of spinal metastases. Spine. 2000;25:2240.
65 Hunt T, Shen FH, Arlet V. Expandable cage placement via a posterolateral approach in lumbar spine reconstructions. Technical note. J Neurosurg Spine. 2006;5:271.
66 Shen FH, Marks I, Shaffrey C, et al. The use of an expandable cage for corpectomy reconstruction of vertebral body tumors through a posterior extracavitary approach: a multicenter consecutive case series of prospectively followed patients. Spine J. 2008;8:329.
67 Wang JC, Boland P, Mitra N, et al. Single-stage posterolateral transpedicular approach for resection of epidural metastatic spine tumors involving the vertebral body with circumferential reconstruction: results in 140 patients. Invited submission from the Joint Section Meeting on Disorders of the Spine and Peripheral Nerves, March 2004. J Neurosurg Spine. 2004;1:287.
68 Sciubba DM, Gallia GL, McGirt MJ, et al. Thoracic kyphotic deformity reduction with a distractible titanium cage via an entirely posterior approach. Neurosurgery. 2007;60:223.