Chapter 166 Posterior Lumbar Fusion by Open Technique
Indications and Techniques
The ultimate goal of a fusion is the elimination of pathologic segmental motion and its accompanying symptoms, achieved by the formation of osseous bridging across the previously mobile level.1 Successful fusion is known as arthrodesis; nonunion is referred to as pseudarthrosis. Surgical techniques have evolved dramatically over the past fifty years. However, despite these advances, there are three basic requirements for a successful fusion: immobilization, fusion bed, and bone graft.2 In the past, immobilization was accomplished with extensive bracing and casts; bracing is still used by some, but immobilization is often achieved by adjunctive instrumentation, mostly transpedicular instrumentation. Historically, the fusion bed and the bone graft were both accomplished through use of iliac crest bone graft; newer alternatives including (cadaveric) allograft bone, demineralized bone matrix, and bone morphogenetic protein (BMP) are now commonly used. This chapter focuses on the history of fusion, its indications, technique, perioperative management, and long-term sequelae.
History of Fusion
The history of modern spinal surgery, particularly fusion, began in the early twentieth century. The first fusion was described in 1911 by two independent surgeons, both of whom were treating patients with spinal tuberculosis, using different sources of bone graft: Albee used a tibial graft and Hibbs an iliac-crest graft (Table 166-1).3–4 The goal of the fusion was to provide stability, which was intended to limit deformity and the spread of tuberculosis. To obtain a successful fusion, these operations required a period of prolonged postoperative bed rest, as well as further immobilization by casts and braces.5 The first description of posterolateral lumbar fusion, involving fusion across the transverse processes, was in 1953 by Watkins.6
Date | Surgeon | Innovation |
---|---|---|
1911 | Albee | First use of tibial graft |
1911 | Hibbs | First use of Iliac crest graft |
1953 | Watkins | First posterolateral fusion (bilateral transverse process fusion) |
1950s | Harrington | Development of instrumentation (used to treat pediatric scoliosis from polio) |
2002 | FDA approval of recombinant human bone morphogenetic protein-2 |
Harrington is credited with being the first to popularize spinal instrumentation: During the 1950s, he treated many children with scoliosis, primarily due to polio, using a complex instrumentation system composed of rods secured to the spine at two ends with sublaminar hooks, which became known as Harrington rods.7 Many variations on these instrumentation systems were then developed. Hartshill rectangles involved stainless steel rectangles held in place by sublaminar wires, which provided the structural integrity of the system.8 Subsequently, plates and then later rods were used in conjunction with transpedicular screws; additionally, translaminar or facet screws have also been used to promote a fusion. The approval of human recombinant BMP-2 (rhBMP-2) in 2002 by the Food and Drug Administration (FDA) is the most recent advance in fusion (see Table 166-1).
Indications for a Fusion
In this chapter, we discuss each of these pathologic degenerative conditions briefly, evaluating some of the key recent, high-quality outcome studies published (Table 166-2). An extensive discussion of the presentation and natural history of these conditions is out of the scope of this chapter; likewise, rather than an exhaustive list of all of the studies that have been performed regarding degenerative conditions, we have highlighted key trials. We limit our discussion of indications to degenerative and infectious conditions. The reader is to keep in mind that fusions are also used for treatment of patients with deformity, spinal trauma, and oncologic conditions, which are discussed in other chapters.
Spondylolisthesis
Spondylolisthesis is the anterior slippage of one vertebral body relative to the adjacent one. It can be divided into five different types based on etiology, first described by Newman and Stone: congenital, spondylolytic, traumatic, degenerative, and pathologic.9 The degree of spondylolisthesis is defined as the percentage of slippage of the vertebral body relative to the adjacent one, with grade 1 indicating only a 0% to 25% slip (Fig. 166-1), grade 2 a 26% to 50% slip, grade 3 a 51% to 75% slip, grade 4 a 76% to 100% slip, and grade 5 greater than 100% slippage (also referred to as spondyloptosis).10 Grade 1 or 2 spondylolisthesis is low grade, and grade 3 or higher is high-grade (Table 166-3). Although displacement can occur posteriorly or laterally, spondylolisthesis is synonymous with anterior displacement of the vertebral body.11
Grade | Percent Slippage | Classification |
---|---|---|
Grade 1 | 0%-25% | Low grade |
Grade 2 | 26%-50% | Low grade |
Grade 3 | 51%-75% | High grade |
Grade 4 | 76%-100% | High grade |
Grade 5 | >100% | Spondyloptosis |
Degenerative low-grade spondylolisthesis is due to a combination of arthritic and degenerative changes in the disc and facet joints that leads to spinal stenosis and vertebral body displacement (Fig. 166-2).12 Degenerative spondylolisthesis is most often seen in patients older than 50 years and has an especially high incidence in women; the most commonly affected level is L4–L5 (the most mobile segmental level in the lumbar spine), and slippage is most commonly less than 30%. Patients typically present with neurogenic claudication, radiculopathy, low back pain, and/or vesicorectal dysfunction. Although listhesis can often be seen on lateral radiographs, further evaluation with computed tomography (CT) scans or magnetic resonance imaging (MRI) (or both) is often useful to exclude other concomitant sources of pain, such as herniated discs.11,13
The initial management of most patients with degenerative spondylolisthesis is nonsurgical. Conservative therapy should include a combination of physical therapy, nonsteroidal antiinflammatory drugs, and lifestyle modification, with most patients showing considerable pain relief. Surgical indications include persistent or recurrent back and leg pain with neurogenic claudication, substantially affecting one’s quality of life, and neurologic deficits, including bowel or bladder dysfunction. The goals of the operation are neural decompression to alleviate symptoms from the stenosis and stabilization to prevent further slippage and instability.11,13
The optimal treatment for patients with degenerative spondylolisthesis has been the subject of many recent studies (including prospective randomized, controlled trials), which provide some of the best evidence for lumbar spinal fusion: In appropriate candidates, surgical intervention is superior to nonoperative treatment.14–18 The highest quality data is provided by the Spine Patient Outcomes Research Trial (SPORT), comparing the effectiveness of surgical versus nonoperative treatment for patients with degenerative spondylolisthesis. Patients were selected from 13 centers in 11 states and were included if they had persistent symptoms (neurogenic claudication or radiculopathy) for greater than 12 weeks, as well as evidence of degenerative spondylolisthesis on lateral static radiographs. Enrollment was in either a randomized cohort or an observational cohort; treatment was decompression (with or without fusion) or standard nonoperative care. There was substantial crossover from patients who were randomized (as 66% of those randomized to receive surgery and 54% of those randomized to conservative treatment had an operation within 4 years), limiting the intention-to-treat analysis. However, the as-treated analysis, involving both the observational and randomized cohorts, showed a substantial and significant benefit to surgery in pain control, physical function, outcome measures [the Oswestry Disability Index (ODI)], patient satisfaction, and overall progress. These improvements were sustained 4 years after surgery.16–17 Although the significant crossover of patients negated the benefits of randomization, the outcomes reported from the SPORT trial likely represent the true clinical effectiveness of spinal fusion for patients with degenerative spondylolisthesis in the American population. The benefit of surgery over conservative treatment has been reported by other authors,18 advocated by experts,15 and included in guidelines for spine surgeons.14
The utility of spinal fusion to improve clinical outcomes in patients with degenerative spondylolisthesis has also been thoroughly investigated, showing that fusion is preferred.14 Although some authors have reported good outcomes in patients treated with decompression alone,19 laminectomy is likely to further destabilize an already unstable spinal segment, with potential worsening of the clinical and radiographic picture.20 Patients who undergo decompression alone have a significantly higher rate of reoperation than those who undergo decompression and fusion.21 A benefit to fusion has, in fact, been seen in all studies evaluating degenerative spondylolisthesis to date,12 with one exception—a retrospective trial comparing laminoplasty (rather than laminectomy) to posterolateral spinal fusion with instrumentation.18
In a landmark study comparing the outcome of decompression and fusion over decompression alone in patients with degenerative lumbar spondylolisthesis, Herkowitz and colleagues showed significant benefit in favor of fusion.22 Another prospective study found a significant improvement in the outcome measures (ODI and SF-36) in patients who had decompression with a posterolateral fusion compared to those receiving decompression alone.23 Several other cohorts of patients with degenerative spondylolisthesis treated with fusion have reported excellent outcomes,24–28 with substantial improvements in outcome measures as well as quality of life25,27; these benefits have persisted at least 5 years after surgery.28 A meta-analysis showed that fusion is significantly more likely to produce a satisfactory clinical outcome than decompression alone (relative risk, 1.40),12 and published guidelines recommend surgical decompression with fusion for appropriate candidates.14,20
Although a fusion is widely accepted for symptomatic patients, the need for instrumentation in achieving posterolateral fusion for degenerative spondylolisthesis has been debated. In the first major study evaluating the benefit of instrumentation in patients with this condition, Fischgrund and colleagues randomized subjects to posterolateral fusion with or without supplemental instrumentation. The use of instrumentation significantly improved fusion rates (82% in the instrumented versus 45%), but no differences were seen in clinical outcome.29 Another prospective randomized trial compared outcomes in patients with spondylolisthesis—both degenerative and spondylolytic—undergoing spinal fusionwith or without transpedicular instrumentation. Although a 5-year follow-up did not find any significant differences, patients with degenerative spondylolisthesis did significantly better with additional instrumentation. Patients with isthmic spondylolisthesis, however, did significantly worse with additional instrumentation. Reoperation rates were significantly higher in those treated with instrumentation, owing to misplacement or failure.30
Transpedicular instrumentation improved outcomes in patients who had a preoperative intervertebral angle difference of more than 11 degrees between flexion and extension in another study, but this benefit was not seen in patients with less motion.31 In yet another study, superior improvement in activities of daily living were seen in patients with transpedicular instrumentation, but there was no significant difference in overall outcome in those with transpedicular instrumentation.32 A meta-analysis confirmed an increased rate of solid fusion in patients with adjunctive instrumentation (relative risk 1.37), but it did not find an improvement in function.12 Interestingly, no difference in successful fusion rate between patients with unilateral versus bilateral one- or two-level fusions was reported in 2007, leading the authors to suggest that unilateral fusion decreases operative time and reduces complications.33
Kornblum and colleagues assessed the outcomes of patients who received a fusion without instrumentation who were treated in the trials of Herkowitz and colleagues and Fischgrund and colleagues. Average follow-up was almost 8 years. Interestingly, patients who had radiographic pseudarthrosis were significantly less likely to have a clinical outcome rated as excellent or good and performed significantly worse in symptom severity and physical function compared to those who had achieved successful radiographic arthrodesis.34
Many surgeons have used this study to advocate for the use of adjunct instrumentation in posterolateral fusion.15,35 The use of supplemental transpedicular instrumentation leads to higher rates of radiographic arthrodesis, a finding that has been associated with better clinical outcomes. Although there is only limited evidence that instrumentation directly improves outcomes in patients with degenerative spondylolisthesis,12 a survey of surgeon members of the North American Spine Society showed that the majority would recommend instrumented fusion,36 and the most recent guidelines suggest the addition of instrumentation to improve fusion rates.14,20,37
Interbody fusion has also shown good clinical outcomes in patients with degenerative spondylolisthesis, with successful union rates as high as 98%.38 Theoretically, the addition of an interbody graft increases the fusion surface area, potentially improving fusion rates.13 Studies that have directly compared posterolateral fusion with or without additional interbody fusion have not shown a clear benefit to one approach in comparison to the other. In one study, patients with preoperative segmental instability had significantly better outcomes with posterior lumbar interbody fusion (PLIF);39 another small study of 24 patients found a significant benefit to lumbar interbody fusion over posterolateral fusion alone.27 However, high rates of complications from interbody fusion for degenerative spondylolisthesis have also been reported. In one study, 8% of patients treated with interbody fusion had permanent neurologic deficits,40 leading the authors to conclude that complete facet takedown might limit morbidity associated with interbody graft placement. No clear data exist favoring the addition of interbody fusion to posterolateral fusion alone, and thus the decision is left up to the surgeon’s expertise and technical preference.
The use of an interspinous process distracter (whereby a spacer is inserted between the spinous processes for the treatment of spinal stenosis)41–42 in combination with nerve root decompression has been attempted. Although this approach has been shown to be superior to conservative management,43 high rates of further progression and surgical reintervention have been shown,44–45 indicating that this approach is likely inferior to fusion in patients with spinal stenosis and degenerative spondylolisthesis.
The treatment of adult patients with high-grade spondylolisthesis is challenging. Operative intervention should be considered in patients with severe pain, neurologic deficits, or progressive deformity.46 The degree of listhesis can cause technical difficulties in the operating room, and postoperative complication rates may be high. Posterior reduction and fusion without decompression has been reported with a low rate of pseudarthrosis (11.4%);47 others have used adjunctive fixation with iliac screws and/or transvertebral screws.48 The most commonly performed operation includes posterior instrumented fixation and fusion, an attempt at partial deformity reduction, and interbody structural support.46
Spondylolysis and Isthmic Spondylolisthesis
Isthmic spondylolisthesis is also common. The pars interarticularis, also referred to as the isthmus, is a thin bone of the posterior arch of the lumbar vertebrae where the lamina and the inferior articular process join the pedicle and superior articular process. A fracture in the pars interarticularis is referred to as spondylolysis, and can be found in 5% to 6% of the population. Isthmic spondylolisthesis results from elongation or traumatic fractures of the pars interarticularis, which lead to dissociation of the anterior and posterior vertebral arches. Anterior shear forces at the level of the listhesis eventually cause degeneration of the disc at the slipped level (Figs. 166-3 and 166-4). Patients are typically much younger than those in whom degenerative spondylolisthesis is seen; although there are a number of pediatric cases of isthmic spondylolisthesis, symptoms most commonly occur between the third and fifth decades of life. The same classification system for the degree of slippage is used in isthmic and degenerative spondylolisthesis, and whereas oblique radiographs can assess spondylolysis, CT is preferred. MRI is helpful for better views of the intervertebral discs and associated stenosis—centrally and foraminally.49–50
Similar to degenerative spondylolisthesis, patients with isthmic spondylolisthesis should have an initial trial of conservative management; precise indications for surgery have not been defined, but it is generally accepted that patients who have failed nonsurgical treatment for longer than 3 months, who have neurologic deficits, or who have significant disability are candidates for surgery.49
There is good evidence that patients with isthmic spondylolisthesis have better outcomes with surgery than with conservative management.51 Moller and Hedlund published a prospective randomized trial comparing patients (aged 18 to 55 years) with lumbar isthmic spondylolisthesis (of any grade), persistent symptoms for at least 1 year, and a “restricted functional ability.” These patients were randomized to posterolateral fusion or to an intensive exercise program; those randomized to posterolateral fusion were additionally randomized to include adjunct transpedicular instrumentation versus autologous iliac crest bone graft alone. At both 1 year and 2 years, those treated surgically had significantly improved pain scores and disability indices compared to those in the exercise group.51 In a long-term follow-up of the same patients (followed for 9 years after surgery), surgical patients had significantly better global assessment and self-satisfaction.52
Fusion is the preferred operation for patients with isthmic spondylolisthesis. Decompression alone is rarely used because it has been associated with poorer outcomes, up to a 27% slippage progression rate, and accelerated disc degeneration.49 Many other studies have reported excellent results in patients with isthmic spondylolisthesis who underwent a fusion.53–55 In one study, 75% of patients reported improvement after fusion.53 Another review of 132 patients followed for an average of almost 10 years found that improvements were seen in back and leg pain in 91.7% and 87.1% of patients, respectively; pseudarthrosis was only seen in 5%.54 Another study with an even longer follow-up (average 20.9 years) found 83% arthrodesis after posterolateral fusion, and only 7% of patients had moderate or severe disability.55 Patients who are working before surgery have been shown to have the best outcomes with fusion operations for isthmic spondylolisthesis; female patients and those who have not had an adequate trial of exercise might also have slightly poorer outcomes.53
The role of adjunct instrumentation to improve fusion in patients with isthmic spondylolisthesis remains debated. In Moller and Hedlund’s study, operative patients were further randomized to undergo stand-alone fusion versus transpedicular instrumented fusion; with a 2-year follow-up, there was no statistically significant difference in fusion rates or outcome in the two groups,56 and in the long-term follow-up of the same cohort, there was still no advantage to instrumentation.52 However, a 2005 meta-analysis showed a significant benefit to instrumentation: Fusion rates were higher (90% versus 77%), as was clinical success (85% versus 64%).57 Instrumentation can also aid in listhesis reduction, when attempted, which has also been argued to improve clinical outcomes in patients with isthmic spondylolisthesis.50 Thus, the use of supplemental instrumentation is preferred in patients with isthmic spondylolisthesis.
The addition of interbody fusion to posterolateral fusion is also debated. Good outcomes, including higher fusion rates, have been reported with PLIF.58 One prospective (but not randomized) study compared posterolateral fusion to PLIF, finding no difference in the two groups.59 Another retrospective study found no difference in clinical outcomes or fusion rate between PLIF and posterolateral fusion, although the PLIF group had better radiographic correction of subluxation.60 Superior clinical outcomes were seen in those treated with posterolateral fusion compared to PLIF in one retrospective study, although there was an insignificant trend toward higher fusion rates in the group treated with PLIF.61 On the other hand, a different prospective trial found better clinical outcomes at 6 months and 1 year after surgery in those treated with a combination of anterior lumbar interbody fusion (ALIF) and posterior instrumentation (although the benefit was not present at 2 years) compared to those treated with posterolateral fusion alone.62 Reviews have disagreed on the potential benefit of circumferential fusion,57,63 and thus, the addition of an interbody fusion is left to the surgeon’s experience.
Spinal Stenosis without Spondylolisthesis
Spinal stenosis without accompanying degenerative spondylolisthesis is distinct from degenerative spondylolisthesis with spinal stenosis. Spinal stenosis describes a narrowed spinal canal with neural compression. Typically occurring in middle-aged and older adults (although spinal stenosis can also be congenital), patients describe back and leg pain, numbness, occasionally weakness, and neurogenic claudication. Peripheral vascular disease, peripheral neuropathy, and degenerative conditions of the hip should be excluded; symptomatic patients should be evaluated by MRI, with or without additional CT scans.64–65
As with other degenerative conditions, the initial management should be nonsurgical. The presence of bowel or bladder dysfunction or neurologic deficits should merit prompt decompression; otherwise, surgery is reserved for those who have intractable pain or severely reduced quality of life and who have failed conservative therapy.65–66 The goals of surgery include pain relief, improved mobility, and prevention of neurologic deterioration. This is often accomplished by decompressive procedures, including laminectomies or laminotomies, partial facetectomies, foraminotomies, with or without discectomies. A fusion is added if there is concern for spinal instability or likely iatrogenic instability from the decompressive measures (Figs. 166-5 and 166-6).66–67
Two prospective randomized, controlled trials have evaluated the benefit to surgery in patients with spinal stenosis but without accompanying degenerative spondylolisthesis. The Finnish Lumbar Spine Research Group described 94 patients who were randomized to nonoperative treatment versus laminectomy of the stenotic segments, with or without instrumented fusion. At both 1 year and 2 years later, patients treated with surgery had greater improvement in leg pain, back pain, and overall disability; owing to the small size of the study, not all of the outcomes were statistically significant, but improvement in disability and back pain (but interestingly, not leg pain) were significantly improved in those treated with surgery.68
The SPORT group also included a trial of patients with lumbar spinal stenosis in the absence of spondylolisthesis who were randomized to decompression surgery without fusion or standard nonoperative care. In this trial, 289 patients were enrolled from 13 centers across the United States. The trial also had significant crossover between the randomized cohorts: the as-treated analysis, which negated the randomization but may be more indicative of outcomes in the American surgical candidates, showed a benefit to surgery in all primary outcomes that was sustained at 2 years.69 This group, moreover, found that surgical intervention was cost-effective compared to many other accepted surgical interventions, such as hip replacement.70
The Maine Lumbar Spine Study was another prospective cohort study comparing surgical treatment to conservative management in 148 patients. Those treated surgically had significantly better back or leg pain and overall satisfaction when followed up to 4 years, although the improvement seen with surgery was greatest initially.71 An 8- to 10-year follow-up of patients in the Maine Lumbar Spine Study found improvement in leg pain and greater back-related functional status seen in those treated surgically; however, by this time, improvements in low back pain and patient satisfaction were similar in those with and without surgical intervention.72 Predictors of good outcome after decompression may include greater walking capacity, milder symptoms, greater satisfaction with general health, and low cardiovascular risk profiles.73
The benefits of the addition of a fusion to a decompressive surgery in patients with spinal stenosis have been debated. Patients with preoperative instability or deformity likely benefit from the addition of a fusion. Extensive decompression can also cause iatrogenic instability, theoretically necessitating fusion (Figs. 166-5 and 166-7); however, supplemental fusion can increase operative times, blood loss, and complication rates as well as costs. Excellent results have been reported after decompression both with74–75 and without69,76 fusion in the treatment of patients with spinal stenosis.
One prospective study evaluating the benefit of laminectomy with or without arthrodesis in the treatment of lumbar spinal stenosis found that those with a fusion had a significant improvement in back pain at 2 months compared to those treated with decompression alone; however, in all other outcome measures there was no benefit to the addition of a fusion.77 Another study with longer follow-up found no significant benefit to the addition of either noninstrumented or instrumented fusion to decompression.78 A recent retrospective study comparing instrumented fusion to noninstrumented fusion found a significant benefit to surgery but no difference between those treated with adjunct transpedicular instrumentation versus iliac crest bone graft alone.75 Thus, the most recent guidelines on the management of patients with spinal stenosis without spondylolisthesis recommend decompression without additional fusion in patients without evidence of instability.65
The role of the addition of an interspinous spacer in addition to decompression in the treatment for spinal stenosis without spondylolisthesis has yet to be fully defined. One study did not find any difference in outcome measures in those in whom a supplemental interspinous spacer was placed.79
Herniated Lumbar Disc
Surgery is the optimal treatment for patients with persistent lumbar radiculopathy due to disc hernation. Both the Maine Lumbar Spine Study and the SPORT trial, which evaluated the treatment of radiculopathy, have shown a benefit to surgical intervention, which persists with long-term follow-up.80–84 The standard operation in these two trials was conventional open discectomy, which is discussed more extensively in the chapter covering lumbar discectomy.
The role of a fusion operation in patients with disc herniation is debated. Most commonly, patients are treated with discectomy alone, and good outcomes with a long period of follow-up have been described.85 However, fusion has been used as a treatment for patients both with primary and recurrent disc herniation. Disabling back pain can occur in up to 15% to 30% of patients who undergo a discectomy; 10% to 20% of patients who have had a discectomy have a subsequent lumbar spine operation.86 The intervertebral disc is the primary stabilizer of the functional spinal unit, decreasing biomechanical forces that are transmitted to the vertebral endplates. Thus, injury to the disc can theoretically lead to segmental spinal instability, and it may be the cause for chronic low back pain in these patients. Thus, some have advocated that a fusion also be performed in conjunction with discectomy for the primary treatment of patients with radiculopathy due to a herniated disc, although this is rarely performed today and is not our practice.87
Prospective trials that have compared discectomy to discectomy and posterolateral fusion in patients with radiculopathy (both primary and recurrent) have found no benefit to routine fusion in patients without instability, deformity, or chronic low back pain. One study evaluating 95 patients who had discectomy either with or without additional fusion found no benefit to fusion, with increased intraoperative blood loss, operative time, length of hospital stay, and total cost of the procedure for those who underwent a fusion.88 Excellent outcomes have also been reported for patients with recurrent disc herniation treated with discectomy alone,89 and one study reporting long-term (average follow-up of 89 months) outcomes in patients treated either by repeated discectomy or posterolateral fusion found no benefits to the addition of a fusion.90
However, fusion might have a role in patients who have post-discectomy chronic low back pain. One group reported that patients with post-discectomy back pain had a 32% relief of pain and a 20% improvement in disability when treated with posterolateral fusion.86 In patients who present with recurrent disc herniation and chronic low back pain, it is reasonable to perform both a discectomy and a fusion of the spinal segment; guidelines also state that fusion should be considered in patients presenting with primary radiculopathy in whom there is evidence of deformity or instability.87 Patients who have a large, centrally herniated disc, causing bilateral radiculopathy or bowel and bladder dysfunction might also require a fusion (Fig. 166-8).
Chronic Low Back Pain
Low back pain is extraordinarily common. The lifetime prevalence of low back pain has been reported to be between 70% and 85%; annually it may be as high as 45%.91 In the vast majority of patients with low back pain, their pain is alleviated within weeks. A much smaller minority of patients—but still an important segment of the population—have chronic debilitating pain, hindering employment and producing a very poor quality of life.
Low-back pain is a symptom, not a disease.92 Even degenerative changes of the lumbar spine is a very broad term, including defined pathologic entities as well as nonspecific changes. The data evaluating surgery, particularly fusion, for patients with chronic low back pain of an unknown etiology are reviewed here. The vast majority of these patients have no accompanying neurologic symptom or radiologic evidence of spondylolisthesis, spondylolysis, spinal canal stenosis, foraminal stenosis, or nerve root impingement by a herniated intervertebral disc. Instead, they present with chronic, debilitating, and unremitting low back pain, and imaging, if notable, is only remarkable for nonspecific degenerative changes.
Management of such patients can be frustrating. Analgesics might only provide moderate pain relief, their side effects not withstanding. There is some evidence that patients with chronic low back pain improve with rehabilitation including cognitive and behavioral components. Data on acupuncture is conflicting but potentially promising. There is far less evidence in favor of a plethora of other interventions, including botulinum toxin injections, local injections, intraspinal steroid injections, epidural injections, facet joint or intradisc injections, radiofrequency denervation, or spinal cord stimulation.92–93
Surgery can alleviate chronic low back pain. The intervertebral disc may be a pain generator, and patients with chronic low back pain can have “discogenic” pain. A review of the contentious debate about discogenic pain is beyond the scope of this chapter. There are no clear indications on history, physical examination, or imaging as to which patients with chronic low back pain have a degenerative intervertebral disc as the source of their pain, because many people with severe degenerative changes of the intervertebral disc on imaging are asymptomatic. Preoperative discography has been used to assess benefit from a fusion, but Resnick and colleagues in 2005 guidelines did not recommend this practice.94
The benefit of a fusion surgery for patients with chronic nonradicular low back pain has been the subject of three randomized, controlled trials (Table 166-4). The evidence, however, raises more questions than it answers.
Table 166-4 Comparison of the Three Major Trials Investigating Patients with Chronic Axial Low Back Pain without Neurologic Symptoms of Unknown Etiology
The first major randomized, controlled clinical trial evaluating the benefit of surgery in patients with chronic low back pain was by the Swedish Lumbar Spine Study Group, which enrolled 294 patients from 19 centers. Inclusion and exclusion criteria are listed in Table 166-4. Within the limited and generally very precisely defined population (although a surgeon’s interpretation of the anatomic location responsible for pain is quite vague), patients were randomized to nonoperative treatment—equivalent to being on a waiting list for surgery, with no precise intervention—versus one of three operative approaches. The results of the trial highly favored surgery. There was a statistically significant reduction in back pain in those treated operatively (although the clinical benefit was perhaps less impressive because only one third of patients improved, compared to 7% of the controls). Outcome measures and return-to-work rate also both significantly favored surgery.95 Not surprisingly, however, surgery was associated with significantly higher cost.91
The surgically treated patients in the Swedish Lumbar Spine Study were randomized to one of three operations: posterolateral fusion with or without instrumentation or posterolateral fusion with interbody fusion. No significant difference was seen among the three groups in outcome measures; patients treated with posterolateral fusion without instrumentation had significantly shorter operations, less requirement for blood transfusion, and shorter hospital stays than the patients treated with instrumentation or interbody fusion.96 With longer follow-up, patients treated with instrumentation or interbody fusion also had significantly higher rates of reoperation.97
Given the potential implications of this study, it is not surprising that it has been thoroughly evaluated.98–99 Although the trial did not compare surgery to any structured intervention,100 this might mimic clinical reality. The inclusion and exclusion criteria may be one of the main factors limiting how this study relates to the general population. Their definition of low back pain was, in fact, quite nonspecific;101 other criteria, however, were quite specific—such as the requirement that patients be out of work for at least 1 year—and might explain some of the differences seen compared with other trials.
Two other prospective randomized, controlled trials have investigated the benefit of surgery in the same population of patients, finding minimal or no benefit to surgery.102–103 The first trial was from Norway and enrolled 64 patients; inclusion and exclusion criteria are listed (and compared with the Swedish Lumbar Spine Study Group) in Table 166-4. Patients were randomized to either instrumented posterolateral lumbar fusion or an intensive rehabilitation program including cognitive behavioral interventions and exercise. Although there was a significant improvement in outcome measures postoperatively in the group treated with surgery compared with preoperative function, there was no difference between surgery and the rehabilitation group at 1-year follow-up.102
The MRC spine stabilization trial compared 349 patients who were randomized to fusion or an intensive rehabilitation program. The minimal inclusion criteria they used were age between 18 and 55 years and at least 1 year of chronic low back pain; exclusion criteria included spinal comorbidities, psychiatric disease, or previous surgical stabilization. There was a significant improvement in the primary functional outcome (ODI) in the patients treated with surgery compared to the rehabilitation group, but the improvement was of questionable clinical significance.103 Subgroup analysis found no difference in outcomes among those randomized to surgery who were treated with posterolateral fusion, interbody fusion, or flexible stabilization; however, posterolateral fusion had the lowest complication rate and cost.92,104
A meta-analysis combining these trials found a significant benefit to surgery, measured by an improvement in ODI; however, owing to the 16% pooled complication rate, surgery was not recommended by these authors.105 Recent reviews and guidelines also give conflicting recommendations, some favoring surgery106 and others saying there is insufficient evidence.100,107–108
Clearly, the best treatment for patients with nonradicular low back pain with nonspecific degenerative changes found on imaging has yet to be precisely defined. There are many possible reasons the data have been so contradictory. First, it is likely that in the absence of a clear pathologic etiology, such as spondylolisthesis, patients grouped into the category of “low back pain” probably have different foci responsible for their symptoms. If surgery is considered, patient selection is key, as well as understanding that the best scenario is likely only a moderate improvement in pain and function. Another reason the data are so divergent may be the wide variability in inclusion and exclusion criteria (see Table 166-4): The MRC spine stabilization trial included most patients who had back pain of unknown etiology for longer than 1 year; the criteria for the Swedish Lumbar Spine Study Group trial were more stringent. Because any benefit to surgery is likely to be only partial, some of their criteria—including disability preventing patients from working for at least 1 year, failure of conservative management, and persistent pain for 2 years—may in fact be quite critical to appropriate selection.
Osteomyelitis and Discitis
There has been a resurgence of spinal infections, in part owing to the increase in immunocompromised patients and the higher prevalence of antibiotic-resistant bacteria (including multidrug-resistant tuberculosis). Infections of the spine can involve the vertebral bodies, the intervertebral discs, the spinal canal, and the surrounding soft tissue (Fig. 166-9); pyogenic infections include bacterial and mycobacterial infections, and the nonpyogenic category encompasses fungi, yeast, and parasites. The presentation of spinal infection is variable but can include fever, focal back pain, and neurologic deficits (ranging from weakness to paralysis); risk factors include diabetes, urinary tract infections, steroid use, malignancy, alcoholism, and other immunocompromised states. Staphylococcus aureus is the most common organism (60% of cases); other important pathogens include Gram-negative rods such as Escherichia coli and Pseudomonas species, as well as Mycobacterium tuberculosis (with spinal tuberculosis seen in approximately 1% of patients with active disease). Fungal infections include coccidiomycosis, blastomycosis, histoplasmosis, cryptococcosis, and sporotrichosis (Table 166-5).109–110
Bacterial organisms |
Precise indications for operative intervention in patients with spinal infections have not been defined. Generally, medical management is initially preferred, except in cases presenting with neurologic deficits, significant kyphotic deformity, or pathologic fractures (Fig. 166-10). Abscesses may need to be drained surgically. Medical management includes antibiotics, with or without supplemental bracing to aid in immobilization. CT-guided biopsy may be necessary to direct antibiotic treatment. Intravenous antibiotic treatment for bacterial infections should be continued for at least 12 weeks; treatment for tuberculosis should include 12 months of antimicrobial therapy if rifampin and isoniazid are used, but longer treatment may be necessary with different drugs. Patients with prolonged refractory infections despite optimal medical management might also be candidates for surgical treatment. The goals of operative treatment include débridement of infected tissue, adequate blood flow for tissue healing, and maintenance or restoration of spinal stability.110
Owing to the importance of spinal stability in preventing neurologic deficits and kyphosis, fusion operations are preferred, especially in patients with tuberculous spondylitis. The best surgical approach is debatable. Owing to the frequent involvement of anterior structures in spinal infections, the intervertebral discs and the vertebral bodies, as well as concerns that posterior decompression might lead to further deformity, some advocate anterior approaches.110
However, concern for reabsorption of the anterior graft and progressive kyphosis has led some to advocate the addition of posterior instrumentation to anterior fusion (thus creating a circumferential fusion) in patients with spinal infection. One small retrospective study found a higher rate of graft dislodgment in patients treated with ALIF alone compared to patients treated with ALIF plus posterior instrumented fusion.111 Another retrospective study evaluating patients with spinal tuberculosis found an increase in kyphosis and graft slippage in patients treated with ALIF alone; these complications did not occur in patients treated with ALIF plus posterior instrumentation.112 This approach has been advocated by other groups as well.113–114
Anterior approaches, with or without supplemental posterior instrumentation, are commonly used for patients with spinal infections, but others have also reported the use of transpedicular decompression and posterior instrumentation for vertebral osteomyelitis.115–118 One group used this approach in patients who had spinal tuberculosis that manifested only with mild kyphosis or early bone destruction, reporting 100% fusion rates and substantial correction of kyphosis.115 Advantages of a single-stage posterior approach include easy access to the spinal canal for neural decompression, prevention of loss of corrected vertebral alignment, and facilitation of early mobilization.116
The use of instrumentation for spinal infections was initially controversial lest it serve as a nidus for persistent infection. However, instrumentation has been successfully used without additional complications in many cases,109 including both transpedicular instrumentation and interbody cages.119 Although infection is currently listed as a contraindication to rhBMP-2 use, it has been used with excellent fusion rates, good clinical outcomes, and no additional complications in patients with pyogenic vertebral osteomyelitis.120–121
Assessment Before a Fusion Operation
Preoperative medical clearance is essential. The most recently published data from national databases have shown that more than half of in-hospital perioperative complications are cardiac, pulmonary, or renal in nature, occurring in patients with chronic conditions.122 In addition to standard preoperative workup, nutritional status should be assessed prior to a fusion, because poor nutritional status places patients at higher risk for complications and pseudarthrosis. A serum albumin level less than 3.5 g/dl and a total lymphocyte count lower than 2000 cells/mm3 are indications that nutrition is suboptimal.123 Owing to the possibility of longer operative times and higher blood loss from instrumented fusion cases, some have advocated predonation of blood for allogeneic transfusion. A recent retrospective case-control study showed no benefit to this practice in patients with a normal coagulation profile.124
Smoking and Fusion
Many studies have shown a detrimental effect of smoking on the fusion rates and outcomes of patients undergoing lumbar fusion. The ability of cigarette smoke to hinder fusion has been seen in a number of animal models125 and may be related to alterations in gene expression or the antiinflammatory effects of smoking.126 Glassman and colleagues showed a significantly increased rate of pseudarthrosis in patients who continued to smoke after surgery (14.2% in nonsmokers versus 26.5% in smokers); lower return-to-work rates were also seen in that population. However, this difference was not present in patients who quit smoking for more than 6 months after their operation compared to nonsmokers.127 Another study showed poor fusion rates and lower patient satisfaction in smokers compared with nonsmokers after instrumented posterolateral fusion, with smoking cessation increasing fusion rates close to those seen in nonsmokers.128 Osteoinductive biological bone graft alternatives might partially overcome the inhibitory effects of smoking. A study evaluating the fusion rates using rhBMP-2 versus iliac crest bone graft in smokers and nonsmokers showed an increased rate of fusion in smokers treated with rhBMP-2 over iliac crest bone graft (95.2% versus 76.2% respectively), but smokers still had consistently poorer clinical outcomes, even if treated with rhBMP-2.129 Although these results are promising, smoking cessation is the best way to improve fusion rates.
Diabetes and Obesity
Diabetes and obesity are common comorbidities in America130 and are becoming increasingly prevalent throughout the world. Both have been shown to increase complications after fusion—especially instrumented spinal operations—and thus preoperative medical optimization of blood sugar and weight loss are crucial.
Type 1 diabetes mellitus (DM type I) and type 2 diabetes mellitus (DM type II) were both shown in a retrospective case-control series to have significantly increased complications after posterior fusion with instrumentation (56% in the T1DM patients versus 21% in controls). Rates of pseudarthrosis were also significantly higher in diabetics: 22% in T2DM subjects, 26% in T1DM subjects, and 5% in controls.131 Another study noted high rates of complications in diabetic patients undergoing instrumented posterolateral fusion (31%), but it also found 75% of patients rated their outcome as excellent or good and 95% of patients achieved radiographic fusion.132 Much of the increased risk of complications comes from an increased rate of surgical site infections (relative risk, 4.10).133 Other hospital complications reported from a large review of the nationwide inpatient sample (NIS) include pneumonia, need for transfusion, and nonroutine discharge from the hospital; diabetes was also significantly associated with higher total costs and longer length of hospital stay.134 Interestingly, subclinical elevations of hemoglobin A1c (above 6.1%) were also found to be significantly associated with increased cost and prolonged hospital stay in patients undergoing lumbar decompression and fusion, suggesting that routine screening of hemoglobin A1c may be beneficial.135
Obesity is also associated with perioperative complications. In a retrospective review of overweight and obese patients, complication rates were found to be directly correlated with body mass index (BMI), independent of hypertension or diabetes: 14% with a BMI of 25, 20% with a BMI of 30, and 36% with a BMI of 35. Some of the complications include pseudarthrosis, wound infection, cerebrospinal fluid leak, deep vein thromboses, cardiac events, pneumonia, urologic issues, and positioning-related nerve palsies.136 A study from the NIS database found higher BMI to be associated with increased transfusion requirements and increased likelihood of discharge to assisted living in patients undergoing fusion; morbidly obese patients also had an increased rate of wound complications and perioperative infections. Rates of thromboembolic events, a known complication of obese surgical patients, were not examined in that study. Perioperative mortality and length of stay were not different, however, suggesting that obese patients are safe surgical candidates.137 Surgical complications and length of stay may be decreased in obese patients undergoing minimally invasive lumbar spinal fusion surgery, suggesting this approach, when clinically indicated, may be considered in obese—especially morbidly obese—patients.138
End-Stage Renal Disease
The prevalence of patients with end-stage renal disease (ESRD) on hemodialysis is increasing, largely owing to the prevalence of hypertension and diabetes mellitus; the shortage of kidney donors places patients with ESRD on hemodialysis for extended periods. Both osteoarthritis and osteoporosis are common in patients with ESRD: osteoarthritis is seen in 23% of patients on hemodialysis; osteoporosis is due to secondary hyperparathyroidism, dynamic bone disease, and steroids. Han and colleagues showed that patients with ESRD have acceptable outcomes after spinal surgery that can improve quality of life; however, morbidity and mortality were higher and fusion rates were comparatively lower, pointing to the importance of preoperative clearance, medical management, and nephrology consultation.139
Owing to the high rates of hypertension, diabetes and cardiac disease, patients with ESRD need these comorbidities managed; echocardiography and possible coronary angiography should be considered. Medical management of osteoporosis should include maintaining calcium, vitamin D, and phosphate levels within normal (with a low-phosphorus diet and phosphate-binding agents if needed). Vitamin D and calcitriol should be used in patients with secondary hyperparathyroidism, and hormone replacement therapy should be considered; bisphosphonates should not be used in patients with ESRD owing to the drugs’ nephrotoxicity and the possibility of hypocalcemia. The goal hemoglobin preoperatively is 10.0 g/dl, and patients should receive appropriate transfusion or treatment with Epogen. Perioperative potassium level should be maintained below 5.0 mEq/L; patients should undergo routine dialysis between 18 and 24 hours before surgery (and sodium polystyrene [Kayexalate] should be administered as necessary) and then dialyzed again 18 to 24 hours after the operation. Postoperatively, ESRD patients should be admitted to the intensive care unit with frequent sodium and potassium checks, because up to half of patients have postoperative hyperkalemia despite these measures (Table 166-6). Other complications reported included spondylodiscitis, postoperative delirium, and an 8% mortality rate. Fusion rate was 57%.139
Table 166-6 Management of Patients Who Have End-Stage Renal Disease and are Undergoing Fusion
Comorbidity | Management |
---|---|
Vascular or cardiac disease |
Rheumatoid Arthritis
Cervical spine disease is well known in patients with RA;140 the lumbar spine is also commonly affected, manifested as degenerative scoliosis, degenerative spondylolisthesis, disc space narrowing, facet erosion, compression fractures, or spinal stenosis. Many patients with RA are treated with corticosteroids, predisposing them to osteopenia and osteoporosis. Nonsteroidal anti-inflammatory drugs (NSAIDs) are commonly used analgesics in patients with inflammatory arthritis; however, because chronic NSAID use has been associated with poorer rates of successful fusion, discontinuation of these agents should be considered.141–144 Disease-modifying drugs such as methotrexate and newer regimens that include anti–tumor necrosis factor-α (TNF-α) drugs are potent immunosuppressants. Crawford and colleagues published a case-control series that retrospectively evaluated RA patients at a major spine center. Although clinical outcomes and fusion rates were similar in patients with and without RA, complications were higher (37% versus 21%), including wound infections and implant complications (16%).145 The most recent rheumatologic guidelines suggest discontinuing any anti-TNF-α agents 2 to 4 weeks before surgery,146 a more prolonged cessation may be necessary in spine surgery to avoid postoperative complications such as discitis.147
Fusing the Aging Spine
As the generation of children born after World War II (known in the United States as the “baby boomers”) age, the percentage of the population that is older is increasing and will continue to do so both in America and elsewhere. The incidence of lumbar spine disease in older adults is very high, especially degenerative spondylolisthesis with spinal stenosis, which often requires a fusion.148 The impact of lumbar spine disease on quality of life can be severe. The recent finding that patients who have had lumbar spine surgery have a lower mortality than the general public has led some to suggest that treating degenerative spine disease is important in keeping patients ambulatory.149
Treating the aging spine, however, has many special considerations. The risk of complications increases with each comorbidity, and it is not uncommon to have older adults with more than three major comorbidities. Additionally, the prevalence of osteoporosis (defined as a t-score less than −2.5 by dual energy x-ray absorptiometry [DEXA] scan) is very high in older adults.150 Owing to decreased bone strength seen in osteoporotic spines, some have advocated non-instrumented fusion. A recent study showing a lack of increased complications with rhBMP-2 in older adults might lead to its wider use in the geriatric population.151 However, many studies have also shown that augmenting transpedicular instrumentation with agents such as polymethyl methacrylate (PMMA) is even more beneficial in osteoporotic patients.152–153
Given the high prevalence of multiple chronic medical conditions in the elderly, it is important to assess the safety and the effectiveness of lumbar spine surgery, especially fusion. One retrospective review of perioperative complications showed a 35% rate of major complications in patients in their ninth decade of life undergoing a fusion, leading the authors to question the safety of fusion in this population.154 Other studies, however, have found much lower rates of complications. Another study that analyzed patients older than 65 years at a single hospital only found a 3% risk of major complications, the most serious of which was an epidural hematoma. Within the subpopulation of patients older than 80 years, there was no statistically significant increase in the rate of complications.155 There was no significant difference between the rate of complications seen in patients older and those younger than 65 years in another study; the only difference they saw was a longer length of stay in the geriatric patients.156 Lumbar fusion surgery is safe in the geriatric population and associated with an acceptable risk profile; however, older adults should also be screened carefully and their medical comorbidities optimized to increase the likelihood of a safe operation.
Excellent outcomes after spinal fusion have been reported in older patients. Sustained improvements in functional outcome measures, including ODI and SF-36, have been described in an exclusively geriatric cohort of patients.157 Similarly, in a study of primarily older adults, high successful fusion rates were reported (82%) in the setting of improvements in the SF-36 that persisted 2 years after surgery.158
Most promising, however, is a study published from a group in Korea. The group assessed the impact of lumbar spine surgery on mortality. Using the Kaplan-Meier method, they compared the 10-year morality of more than 1000 patients to age- and sex- matched controls. They found that in comparison to the controls, standardized mortality ratios all favored surgery: 0.21 for patients aged 50 to 59 years, 0.53 for patients aged 60 to 69 years, and 0.45 for patients aged 70 to 85 years. Additionally, patients who had a fusion had a statistically significant lower mortality than those who had decompression alone, although this might relate to surgeons being less likely to offer high surgical risk patients a fusion procedure. The authors compare the benefit of lumbar spine fusion to studies that have shown that patients who have hip replacement also have a reduced mortality, hypothesizing that a key factor may be the improvement in ambulation allowing patients to participate in regular exercise.149 The ability to generalize data from the South Korean population to American patients, however, may be limited, because rates of diabetes and obesity are lower in South Korea and life expectancy is longer.
Adjuncts to improve fusion have been used in the geriatric population. RhBMP-2 has been shown to decrease complications and, surprisingly, cost, in comparison to iliac crest bone graft in one prospective randomized clinical trial.151 As described in more detail later, direct-current electrical stimulation (DCS) has also been studied in older adults and found to improve clinical outcomes, namely median walking distance, although fusion rates were not improved.159–160
In addition to greater risk of complications, older adults also have a much higher rate of osteoporosis. Another study from Korea showed that in the population at the authors’ institution, among patients older than 50 years, 14.5% of men and 51.3% of women had osteoporosis confirmed by DEXA scan.150 Patients with osteoporosis need comprehensive medical management before surgery. At least 1200 mg of calcium and 1200 IU of vitamin D should be taken daily. Raloxifene, a selective estrogen receptor modulator, has been shown to reduce the risk of vertebral fractures and should be considered in patients who do not have an underlying disposition to or history of venous thromboembolism. Bisphosphonates have also been shown to decrease the rates of vertebral fractures; alendronate 70 mg once weekly and risendronate 35 mg weekly are the most commonly used regimens. Other options that are less commonly used include nasal insufflation of calcitonin and daily subcutaneous injections of parathyroid hormone, both of which are best prescribed in conjunction with endocrinologists.161 Medical management should continue in the postoperative period; although there has been some concern that bisphosphonates might inhibit fusion, animal models do not suggest this to be the case.162
The preoperative planning of fusion cases requires special consideration in patients with osteoporosis. Some surgeons are reluctant to use instrumentation in this population owing to the risks of instrumentation failure (Fig. 166-11). However, successful use of transpedicular instrumentation has been described in the geriatric and osteoporotic population, sometimes using less-rigid rods.163–164 Strategies to supplement transpedicular instrumentation have also been used. Some have described the use of transpedicular cement injection, creating a vertebroplasty-like effect by strengthening the vertebral body and providing additional stability to the anchoring of the screws.165 Similarly, PMMA has also been shown to augment cannulated pedicle screws with successful arthrodesis demonstrated radiologically; the major additional risk of this procedure is a theoretical pulmonary cement embolism.153,166 Balloon kyphoplasty augmented screws have also been proposed, but cadaveric studies suggest PMMA augmentation may be superior.152,167–168
Perioperative Antibiotic Prophylaxis
Perioperative antibiotic prophylaxis was first found to be effective in spine surgery in 1975.169 Several retrospective reviews and a few randomized, controlled trials have established the efficacy of antibiotic prophylaxis in both non-instrumented and instrumented spinal surgery, but many questions remain, including the optimal type of antibiotic, its dosing, and its duration.170
Most prospective randomized, controlled trials examining antibiotic prophylaxis have included a range of procedures, including spinal. One trial compared ceftizoxime, a third-generation cephalosporin, to a combination of vancomycin and gentamicin. Surgical site infections were highest in spinal patients in this study, but there was no difference in infection rate between the two regimens, leading the authors to recommend ceftizoxime owing to its lower toxicity and superior penetration to the cerebrospinal fluid (CSF).171 Another large prospective study including 956 patients undergoing laminectomy, craniotomy, or other neurosurgical procedures assessed the efficacy of cefuroxime, a second-generation cephalosporin. Perioperative infection rates were found to be only 0.5% at 8 weeks, but no control group was included for comparison.172 The only large double-blind, placebo-controlled, randomized trial of antibiotic prophylaxis in spine patients examined 1.5 g of cefuroxime in non-instrumented cases. The trial had more than 600 patients in each arm and showed a statistically significant increase in deep infections (spondylodiscitis or epidural abscess) in patients without prophylaxis. Despite the large number of patients enrolled, the comparison of total infection in controls versus subjects only trended toward significance, owing to the low infection rate overall (2.8% in controls versus 1.3% in the treatment group).173
Rates of surgical site infections are known to be higher in instrumented spinal cases. An implanted prosthesis can act as a nidus of infection for bacteria that can produce glycocalyx—forming a barrier to the penetration of antibiotics.174 Whereas no trial has definitively established the role of antibiotic prophylaxis in instrumented spinal cases, one trial enrolled 233 patients comparing a single intravenous dose of cefazolin to 10 days of antibiotic prophylaxis (using IV cefazolin for 3 days followed by oral cephalexin). Infection rates were higher in the group treated with only one perioperative dose (4.3% versus 1.7%), but the trial was significantly underpowered.175 Furthermore, a retrospective study did not find any differences in the rate of surgical site infection between a group treated with 24 hours of prophylaxis with ampicillin compared to another group treated with a longer duration of antibiotics, and more resistant organisms were seen in the group taking prolonged prophylaxis. The authors concluded that longer duration does not provide more extensive coverage of surgical site infection but might select for resistance.176
Other studies have also supported the use of antibiotic prophylaxis in spinal fusion cases.177 One group in Italy reported wound infection rates less than 1% in their cohort of patients treated with perioperative ampicillin and sulbactam.178 A meta-analysis found that cumulative rates of infection were 2.2% in patients treated with a variety of regimens compared to 5.9% in those who received no prophylaxis; the statistically significant pooled odds ratio was 0.37 favoring antibiotics.179
Although the only antibiotic proved to have efficacy in preventing surgical site infections in spine patients is cefuroxime, cefazolin remains the most commonly used antibiotic for prophylaxis in spinal operations. Advantages of cefazolin include its coverage of the most common organisms that cause surgical site infections—including Staphylococcus spp, Streptococcus spp, Escherichia coli, and Proteus spp—with minimal coverage of additional pathogens, good penetration into subcutaneous tissue as well as bone, the longest half-life of the cephalospohins in serum and bone, fewer side effects, and lower cost. For patients with a true cephalosphorin allergy, clindamycin or vancomycin are valid alternatives; given the low degree of cross-reactivity between penicillin and cephalosphorin allergies, a penicillin allergy is not an absolute contraindication to cefazolin administration.174 The major concern with cefazolin in spinal procedures is its limited penetration into the intervertebral disc space.180
The increasing prevalence of methicillin-resistant Staphylococcus aureus (MRSA) has caused some to advocate vancomycin for surgical prophylaxis. Known risk factors for MRSA wound infection in spinal surgery include lymphopenia, history of chronic infections, alcohol abuse, and recent hospitalizations.181 A recent study of patients showed that those found to be colonized with MRSA had a surgical site infection rate of 23.5% compared to 4.1% in those who were not colonized.182 It is reasonable to screen patients for MRSA at the preoperative evaluation for elective cases in areas with high prevalence, administering vancomycin if the patient is colonized.183
The timing and redosing of antibiotic prophylaxis is critical. The first dose should be given within 30 minutes of skin incision; inappropriate timing is a significant risk factor for deep surgical site infection after spinal fusion operations.184 Cefazolin should be redosed after 1500 ml of blood loss or when the surgery exceeds 4 hours.185–186 Interestingly, a fraction of inspired oxygen less than 50% intraoperatively is associated with higher rates of infection;187 ultraclean air using vertical laminar flow has been shown to decrease the rate of infection in instrumented fusion cases.188
Many surgeons place a closed-wound suction drainage system deep to the lumbodorsal fascia before closure. The rationale is that when blood collects in a surgical wound, it creates an environment where bacteria can multiply; additionally, many believe drains reduce the risk of epidural hematoma or seroma formation. There are no data to support closed-wound suction drains, but they have not been sufficiently evaluated. Two prospective randomized trials examined closed-wound drains, one in patients undergoing laminectomy189 and another in patients treated with “more extensive lumbar spine surgery.”190 Both trials did not see a statistically significant difference in complications, but they were significantly underpowered. Another small retrospective study did not find a higher rate of complications in patients in whom no drain was placed.191 Drains can theoretically be a port of entry for infection; therefore, some surgeons continue antibiotic prophylaxis until they are removed. The decision to use a closed suction wound drain should be left to the surgeon until higher-quality data are available.
Techniques
After successful endotrachael intubation, the patient is positioned prone on a Jackson frame table to maintain lumbar lordosis (Fig. 166-12). The surgeon should verify with the anesthesiologist that antibiotics have been administered. All pressure points are covered with padding. The posterior lumbosacral area is subsequently shaved and prepped with povodine-iodine (Betadine), alcohol, and iodine 0.7%/isopropyl alcohol 74% weight per weight (DuraPrep, 3M, St. Paul, MN). The area is draped after the skin has dried. The exposed skin is covered with antimicrobial drapes (such as Ioban, 3M, St. Paul, MN) to minimize skin pathogens.
A midline lumbar incision is carried out. The lumbosacral fascia is identified and incised in the midline. Muscle is stripped off the spinous processes and the laminae in a subperiosteal fashion (Fig. 166-13). Special care is taken not to disrupt the facet joint and its overlying capsule. At the inferior and lateral border of the lamina lies the inferior articulating process, which meets with the adjacent superior articulating process, comprising the facet joint. At the superior and lateral border of the lamina lies the pars interarticularis. Immediately superior to the pars interarticularis and farther lateral lies the ventrally situated transverse process. The pedicle can be found at the junction of the pars interarticularis, transverse process, and superior articulating process (Fig. 166-14).
Dissection should be carried out at the superior and lateral edge of the lamina to expose the transverse processes. We prefer to cannulate the pedicles at this stage of the procedure; others prefer to perform a decompression before cannulating the pedicles. The landmarks used for cannulating the pedicles are the meeting point of the pars interarticularis, the superior articulating process, and the transverse process. There is a small ridge of bone at that level called the mammillary process. A high-speed drill is used to decorticate that point, followed by the use of a pedicle finder to cannulate the pedicle. There are two geometric planes at this point: mediolateral and rostrocaudal. In the rostrocaudal plane, when one is at L4, one should be almost 90 degrees perpendicular to the floor. At L5 and S1, the probe will be pointing inferiorly toward the caudal end with respect to the floor; at L1 to L3, the probe is pointing superiorly to the rostral end with respect to the floor. An intraoperative x-ray can aid in assessing the orientation of the various pedicles to the floor. In the mediolateral plane, going from L1 to S1, the pedicle increases in its medial configuration; thus, there should be between 5 and 10 degrees of medial angulation at L1 and a 25-degree medial angulation at S1. No force should be exerted during the cannulation of the pedicle, because the probe should be inside cancellous bone. Any resistance to the insertion of the probe should alert the operator that the probe is close to a cortical surface (medially, laterally, superiorly, or inferiorly). In that case, the surgeon should change the probe’s angle accordingly, looking for the area of least resistance. This process can only take place if the probe is inserted gently, so as to be able to rely on tactile feedback in order to cannulate the pedicle without cortical breaches. This portion of the operation can be done under fluoroscopic guidance.
Once the pedicles are cannulated, a feeler probe is inserted and used to feel all five walls of the pedicles, including the ventral, medial, lateral, inferior, and superior surfaces. An x-ray is obtained at this time, with markers placed inside the cannulated pedicles, to confirm positioning. Laminectomies and medial facetectomies are performed with a high-speed diamond-bit drill. The ligamentum flavum is removed with a nerve hook, blade, and multiple sized Kerrison rongeurs. The thecal sac and its dural covering are identified. We prefer to use free-running electromyography (EMG) to ensure gentle manipulations of the thecal sac and nerve roots. The bony decompression is carried out laterally until the medial border of the pedicle is palpated with a Woodson dissector. The segment of the nerve root medial to the pedicle is known as the lateral recess; the segment of the root inferior to the pedicle is the foraminal component. Nerve root decompression should encompass both the nerve root in its lateral recess (medial to the pedicle) and in its foramen (inferior to the pedicle). At this point, a nerve hook can be placed under the nerve root, and dissection is carried out superiorly until the disc is palpated and visualized. Lumbar discs lie closer to the inferior pedicle than the superior pedicle. Thus, a posterolateral disc herniation affects the traversing nerve root (lower) and not the exiting nerve root (upper). A foraminal or far-lateral disc herniation causes exiting root compression.
Once the central and root decompression is completed, attention is turned to the fusion. The transverse processes and the facet joints are decorticated with a high-speed drill (Fig. 166-15). The cannulated pedicle holes are pretapped with a 5-mm tap, followed by placement of 6- or 6.5-mm diameter screws (7-mm bicortical screws are used in the sacrum). The screws can then be stimulated for up to a threshold of 10 mA. One can confirm a lack of breach with a Woodson dissector placed medial and inferior to the pedicle. An x-ray is obtained at this point to ensure proper placement of screws. Two lordotic rods are fashioned, secured to the polyaxial screwheads, and tightened with caps accordingly (Fig. 166-16). If reduction is necessary, for example in the case of an L4–L5 spondylolisthesis, the L4 screws lie ventral to the dorsally placed L5 screws; the rod is initially secured to the L5 screws and kept dorsal to the L4 screws. Reduction instruments are then placed on top of the L4 screw head bilaterally and serve to pull the screw up to the dorsally placed rods. The reduction should be done under free-running EMG to prevent nerve root compression.
Following the reduction and instrumentation placement, copious irrigation with antibiotics is carried out with a pulse lavage. The retractors are removed and adequate hemostasis of the muscle laterally is obtained. Two Cobb elevators are used to expose the lateral gutters (the area lateral to the joint where the transverse processes lie). The bone graft is then directly placed on top of the previously arthrodesed transverse processes and joints (graft can be either allograft or autograft, in addition to demineralized bone matrix or BMP, see Fig. 166-16). We have found that removing the retractors before placing the graft prevents dislodgement of the graft into the exposed central canal during that process. Inspection of the central canal is recommended after the Cobb elevators are removed, to ensure no medial spillage of the graft. A subfacial closed-suction wound drainage system is left in place deep to the lumbodorsal fascia, and the wound is closed in multiple layers.
Intraoperative Electrophysiologic Monitoring and Lumbar Fusion
The use of intraoperative electrophysiologic monitoring, in particular EMG, has become routine during lumbar spine operations. Neurologic complications can arise from direct injury to the spinal nerve roots, especially during transpedicular instrumentation placement; vascular complications from ischemia secondary to hypotension can lead to neurologic dysfunction.192 The goal of monitoring is to detect these complications and make appropriate changes intraoperatively to prevent long-term neurologic deficits. Although a postoperative neurologic deficit can often be detected by intraoperative changes in monitoring, there is also the risk that false-positive changes might cause surgeons to make unnecessary adjustments, possibly leading to suboptimal placement of instrumentation.193 In complex spinal cases, external anal and urethral
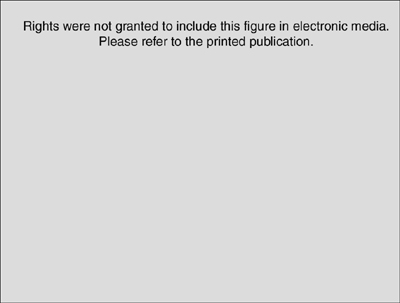
FIGURE 166-17 Illustration showing the dissection, decortication, instrumentation, and bone graft packing.
sphincter monitoring may be used, but this is often not needed in posterolateral instrumented fusion.194
When a change in intraoperative electrophysiologic monitoring occurs, the goal is to determine what might have caused this change. For example, a recently placed pedicle screw may be the presumed culprit; anatomic or radiographic assessment of the location can indicate if the screw should be removed. When there is no clear reason for the change, a series of steps should be taken: The electrodes checked to see that they have not become displaced; any inhalational anesthetics stopped; the mean blood pressure kept above 90 mm Hg to increase perfusion to the spinal cord; the fraction of inspired oxygen increased; the wound irrigated with warm saline; and the location of any instrumentation reassessed for possible empiric removal and replacement.192
Electromyography provides information on the integrity of the motor roots. EMGs can be classified into two categories: Electrically elicited EMGs are useful during the static phases of surgery, and mechanically elicited EMGs (based on action potentials) are useful during dynamic phases of surgery.192 Bose and colleagues published a study showing that EMGs were useful in detecting breaches of the pedicle cortex during transpedicular instrumentation; abnormal signals were found in six patients, but owing to intraoperative changes in the screw placement, normal signals were recovered and no long-term neurologic injuries occurred.195 Other studies have also found a correlation between EMG thresholds and lumbar pedicle screw malposition. Raynor and colleagues in an analysis of 4857 screws showed that the probability of a breach of the medial pedicle wall was correlated with the amplitude of the stimulation threshold: 0.31% for greater than 8.0 mA, 17.4% for 4.0 to 8.0 mA, 54.2% for less than 4.0 mA, and 100% for less than 2.8 mA. From their data, they calculated the sensitivity and specificity of a medial pedicle wall breach at 8.0 mA to be 94% and 86%, respectively.196 Despite the benefit of EMGs to determine the accuracy of pedicle screw placement, especially when used in conjunction with intraoperative fluoroscopy, misplacement occurs; urgent CT should be considered to confirm proper screw placement if the patient complains postoperatively of new neurologic deficit or radiculopathy.197
Adjuvant Osteoinductive Biological Agents: Bone Morphogenetic Protein
In 1965, Urist described a protein with the potential of inducing new bone formation. This protein became known as BMP.198 The protein was purified, and the genes were isolated and subsequently cloned. Now it is known that there is in fact a whole family of growth factors, BMPs, that are related to the transforming growth factor-β family. BMPs are homologous proteins that are secreted as homodimers or heterodimers and have autocrine and paracrine effects. BMPs are believed to be important in bone remodeling and in the regulation of osteoblastic and osteoclastic activity.
Although iliac crest bone graft was, and remains, the gold standard for lumbar fusion, its use is not ideal. Chronic bone graft site pain, discussed later, can be substantial and debilitating. The addition of bone graft harvesting to an operation increases operative time and blood loss. Allograft bone has also been used, but high cost, risk of disease transmission, and lack of osteoinductive capacity are limiting factors. Thus, the spine community has been eager for alternatives to autologous bone graft. BMP has proved to be one such alternative.199
Early studies of human recombinant BMP in animal models were promising, but initial clinical trials, some of which were not published, were disappointing. These trials likely failed owing to suboptimal dosing and the lack of an appropriate carrier matrix to deliver BMP.200 In 2000, a pilot study was published showing the efficacy of rhBMP-2 to induce bone formation with anterior interbody cages.201 As a result, in 2002, the FDA approved the use of InFUSE (rhBMP-2 applied to a collagen sponge) in a lumbar tapered fusion device cage for ALIF. Since then, there has been significant off-label use of rhBMP-2.
The initial study that demonstrated the ability of rhBMP-2 to improve fusion rates in posterolateral fusion was published in 2002. Twenty-five patients were randomized to autograft and supplemental instrumentation versus rhBMP-2 with or without supplemental instrumentation. All patients treated with rhBMP-2 achieved radiographic fusion; only 40% in the group treated with autograft and supplemental instrumentation had arthrodesis. Additionally, statistically significantly greater and faster improvements were seen in those treated with rhBMP-2.200
Several larger studies with longer follow-up have confirmed the efficacy of rhBMP-2 to produce superior fusion rates and clinical outcomes in posterolateral fusion. One study published in 2005 investigated 74 patients randomized to iliac crest bone graft versus rhBMP-2; patients treated with BMP had a more-rapid development of fusion mass.202 Subsequently, a study using a higher dose of BMP (20 mg per side, compared to 6 mg per side initially used) randomized patients to iliac crest bone graft or rhBMP-2 with a compression-resistant matrix. Fusion rates were higher, complication rates (blood loss and surgical time) were lower, and clinical improvements were superior in the group treated with rhBMP-2.203 Not only did patients treated with rhBMP-2 on an absorbable collagen sponge have larger and more consistent posterolateral fusion masses compared to iliac crest in another study, but also 92% of these patients referred to their outcomes as excellent, compared with 27% in the control (iliac crest) group.204 The follow-up in many of these trials was short, but a longer-term follow-up study (with an average follow-up of 34 months) found that clinical and radiographic improvements were sustained.205 Other studies have also reported very high radiographic fusion rates (greater than 93%) in the absence of supplemental instrumentation in posterolateral approaches.206–207 High fusion rates have also been reported using other approaches, including interbody fusion;208–210 however, early graft lucency and subsidence have been described in patients treated with interbody fusion, and patients should be assessed if this approach is used.208
A 2008 meta-analysis thoroughly evaluated the effectiveness of BMP to promote fusion. Compared to iliac crest bone graft, BMP-2 was found to have a higher rate of arthrodesis (relative risk, 0.42, p < 0.00001). Patients treated with BMP had shorter hospital stays than those treated conventionally. Interestingly, BMP might also be more effective in promoting fusion in instrumented posterolateral fusions than non-instrumented approaches.211 Other studies, reviewed later, have also found low complication rates in patients with lumbar pathology undergoing fusion, not significantly different from those treated with autologous bone.212 rhBMP-2 might represent a valid alternative to autologous bone graft for spinal fusion, and it might have the best outcomes when combined with transpedicular instrumentation.
The high cost of BMPs has thwarted its use; the cost in 2003 for BMP-2 was $3380.213 However, because of faster return-to-work, shorter operative times, and a lower rates of repeat operations seen in patients treated with BMP, models in both the United States and in Europe have suggested that BMP may be cost-effective.214–215
Adjunct External Bone Stimulation
External bone stimulation through electrical coupling has been used to improve fusion rates and surgical outcomes. It has been known for decades that bone produces electrical potentials under stress. This led to the hypothesis that electricity could accelerate bone formation. The first reported use in spine was in 1974 by Dwyer and colleagues;216 subsequently there has been great interest in the development and use of these devices.217 Three forms of electrical stimulation are available: DCS, pulsed electromagnetic field stimulation (PEMFS), and capacitate coupling stimulation (CCS).
DCS involves the implantation of a device during surgery at the location where fusion is to occur, and it is often removed after successful arthrodesis, commonly 1 year after the initial operation. PEMFS is based on an electromagnetic field inducing electrical currents at the site of desired fusion; the device is placed in a brace that patients wear for several hours each day. CCS is also noninvasive: Two electrodes are placed on the skin over the operative site, and they are connected to an external battery-powered device. Many studies were published in the 1990s showing the potential of these devices—particularly DCS—to improve fusion rates, especially in patients at high risk for pseudarthrosis. However, these devices remain underused. This is partly because of their high cost (the cost of DCS in the United States was $5075 in 2006), and partly because DCS requires the implantation of a foreign body and a separate procedure for removal. PEMFS and CC can have variable outcomes in practice owing to patient adherence. Two papers published in 2009 showing that DCS improves outcomes in patients older than 65 years,159–160 along with economic models speculating that DCS is cost effective, might lead to increased use in the future.
Direct-Current Electrical Stimulation
DCS is an implantable device. The cathode is placed over decorticated transverse processes in posterolateral fusions and the anode is placed in nearby tissue. A hermetically sealed generator provides constant current. Variable levels of currents are used, most commonly between 20 and 40 μA; levels greater than 100 μA should be avoided because of potential osteonecrosis and surrounding tissue death.218 These devices are typically removed after 6 months to 1 year after their initial implantation, although criteria have not been published. The rates of infection and malfunction are unknown, as well.219
The mechanism of action of DCS remains unknown. Studies suggest that DCS might modify electrophysiologic changes that are known to occur during bone healing, including attraction of growth factors, movement of osteoblasts and endothelial cells to the site of fusion, and polarization of cell membranes.220–221 The electrochemical reactions at the cathode of DCS create a number of byproducts that have local effects: An increase in the pH promotes osteoblastic activity and decreases osteoclastic activity; the consumption of oxygen also augments osteoblasts; and the secretion of hydrogen peroxide causes macrophages to release vascular endothelial growth factor (VEGF), which promotes bone healing and maturation of blood vessels.218 DCS has been shown in mouse models to elevate the expression of mRNA of several growth factors important in bone maturation, including BMP-2, BMP-6, and BMP-7, which may be responsible for the bone formation seen with DCS.222
Animal model studies have shown that DCS promotes spinal fusion. Studies in a rabbit model found the response to be dose-dependent, with better results seen at 60 μA current than at 20 μA.223 The ability of DCS to improve arthrodesis has been seen in sheep and nonhuman primate models of posterolateral fusion and interbody fusion.224–225
Several studies have evaluated the efficacy of DCS to achieve arthrodesis, especially in patients at risk for pseudarthrosis. One prospective study evaluated 94 patients who underwent fusion with instrumentation: they found 96% of patients with DCS and 85% of patients without DCS achieved radiographic fusion; the benefits of DCS extended to their high-risk group, which included smokers, patients with multiple prior surgeries, and patients with multiple level fusions.226 A different retrospective study also found a benefit to DCS: Of 130 patients undergoing instrumented fusion, half had adjunct implantable electrical stimulation. Fusion rates and clinical success (measured by a rating scale) were statistically significantly higher in the cohort with DCS (95.6% fusion); there was also a large improvement in a subgroup who were workers’ compensation patients.227 The benefits of DCS were not seen in another study, however, where patients were prospectively assigned to DCS, PEMFS, or no adjuvant external bone stimulator. No statistically significant differences were seen among the three groups in fusion or outcomes, but the small sample size of the groups (n = 17–22) caused the study to be too underpowered to detect differences.228–229
Andersen and colleagues published two articles in 2009 describing a randomized, controlled multicenter trial evaluating the efficacy of DCS in 107 patients older than 60 years treated primarily for spinal stenosis with decompression and non-instrumented fusion. The randomized patients received adjunct DCS, and the control patients had no additional intervention. At 1 year, patients treated with DCS had significantly better outcomes on three of four categories in the Dallas Pain Questionnaire and, perhaps most strikingly, better median walking distance.159 Fusion rates (assessed by CT scans) were not significantly different between the two groups and were quite low: 35% versus 36%. Successful arthrodesis was associated with better functional outcome and pain scores; fusion rates were also much higher in smokers randomized to DCS.160 That superior functional status was seen in the absence of differences in fusion rates led the authors to question whether some bias was introduced into the surgery itself (as the trial was not double-blinded), whereby the surgeons might have performed a more meticulous decompression in the patients receiving the DCS, thus explaining the superior walking distance. That is more plausible than attributing the differences to DCS.
Despite the high cost of the device and the procedure for removal, some have argued that the addition of DCS to instrumented or non-instrumented posterolateral fusion may be cost-effective. One study showed that patients with DCS had lower costs and shorter stays.230
Pulsed Electromagnetic Field Stimulation
PEMFS is based on the ability of a magnetic field to induce a secondary electric field at the location of desired fusion. The device involves current-carrying coils driven by a generator that a patient wears in a brace for 3 to 8 hours each day. Patient compliance is an important variable.229
Few studies have evaluated PEMFS. An initial study that was randomized and blinded showed that patients who wore the brace for more than 8 hours a day had higher fusion rates than those who had a brace with nonfunctional coils or those who used their brace for less than 4 hours per day.231 Other retrospective reviews have also found significantly higher fusion rates, as high as 98%.232–233 However, another study of patients who wore the PEMFS device for at least 2 hours a day did not show any improvement in fusion rates.234 Although there are limited data in support of PEMFS as an adjuvant therapy, given that it is noninvasive and there are no known side effects, its use might become more widespread after further research.
Capacitative Coupling Stimulation
CCS is another noninvasive device consisting of electrodes connected to an alternating-current signal generator. patients are encouraged to wear the device 24 hours per day, making patient adherence key.229 CCS was studied in one large double-blinded trial of 179 patients randomized to CCS or a placebo device. Both fusion rates (84.7% with CCS versus 64.9% with the placebo) and clinical outcomes were significantly better in patients treated with CCS after posterolateral fusion. Smokers also had much higher rates of fusion if treated by CCS.235 Unfortunately, there have been no clinical studies examining CCS since this study was published in 2006.218
Complications After Fusion Surgery
The rate of complications and perioperative mortality after open posterior lumbar fusion are fairly low. Perioperative mortality rates have been found to be 0.15% to 0.29%.122,236 The most common preventable cause of death after lumbar fusion may be analgesia overuse—either accidental overdose or intentional suicide.236 Surgical site infections, both superficial and deep, are among the most common short-term complications. In addition to standard postoperative complications, incidental durotomy causing a cerebrospinal fluid leak, spinal epidural hematoma, and other postoperative causes of cauda equina syndrome, neurologic injury, rhabdomyolysis, and sudden vision loss are also known to occur. Long-term complications include adjacent level disease, pseudarthrosis, and chronic pain from the donor allograft site.
Mortality
Mortality is the ultimate adverse outcome and is fortunately quite low after lumbar fusion. Data from the NIS database from 1993 to 2002 on 66,601 postoperative hospitalizations across America where degenerative spondylolisthesis was the primary admitting diagnosis showed in-hospital mortality as 0.15%. Complication rates were also low, the most common being hematoma or seroma formation (5.4%). More than half of the complications were cardiac, pulmonary, or renal, stressing the importance of preoperative medical optimization and clearance before surgery. Rates of neurologic and infectious complications each occurred in less than 1% of patients, although because of the reliance of the NIS on discharge coding and complications that occurred during the initial hospitalization, the rates of infection may be underrepreresented.122 A study of Washington State workers’ compensation claims from 1994 to 2001 showed that the 90-day perioperative mortality was also quite low, 0.29%, and the 3-year cumulative mortality rate was 1.93%. The most common cause of death within this period was analgesia overuse, primarily from opiates; most of these were accidental, but some were suicidal.236 Posterior lumbar fusion is quite safe; however, patients whose symptoms are not fully alleviated are at risk for analgesia-associated mortality, and consultation with chronic pain specialists is highly recommended.
Perioperative Complications
The most devastating perioperative complication after lumbar spine fusion, with the exception of mortality, is neurologic injury. As discussed earlier, utmost care is taken intraoperatively to prevent injury to the cauda equina and nerve roots, and intraoperative neuromonitoring (free-running EMGs) can aid the surgeon in making modifications to his or her technique. Cauda equina syndrome is rare after spinal fusion (with a reported incidence of 0.38%)237 and can result from a number of intraoperative or postoperative conditions, including direct trauma secondary to zealous manipulation, instrumentation misplacement, and postoperative epidural hematoma. If there is concern for cauda equina syndrome, emergent MRI and/or operative exploration should be considered.2 Additionally, epidural spinal cord compression from inadvertent extravasation of hemostatic gelatin matrix has also been described, showing that judicious use of gelatin matrix should be considered.238
Incidental durotomies can occur in any spinal procedure. If durotomy is detected during surgery, primary closure should be attempted with nonabsorbable sutures; fibrin glue may be used to supplement the primary closure. The most serious potential sequelae of dural violation is a CSF leak causing a pseudomeningocele (Fig. 166-18) or a CSF fistula; the latter can lead to meningitis. Additional concerns are that the CSF leak can collapse the thecal sac and lead to an increase in blood loss from epidural bleeding. The durotomy itself may be associated with injury to nerve roots. Vigilant monitoring for any signs of a CSF leak is essential, because cerebellar hemorrhage causing herniation has been reported after spinal fusion when a CSF leak went undetected.239 A lymphatic fluid fistula, initially diagnosed as a CSF leak, has been reported, and was hypothesized to be due to injury caused during lumbar transpedicular screw fixation.240
Surgical site infection is known to be higher in spinal fusion cases than other spinal procedures. Longer operative time, higher blood loss, and instrumentation implantation are all known risk factors for postoperative infections, placing fusion procedures—especially with instrumentation—at higher risk. The rate of postoperative infection (including superficial and deep infections) after spinal fusion ranges between 0.65% and 5% in most papers,237,241–247 although rates up to 12% have been reported.123,248 Known risk factors for postoperative wound infection in spinal fusion cases are obesity (especially morbid obesity),136,187,241,243,245 diabetes,133,243,245–246 history of prior surgical site infection,243,245 alcohol abuse,245 greater than 1 L of estimated (operative) blood loss,243 longer operation times,187,243,249 instrumentation use,187,246 postoperative incontinence,241,248 procedure for tumor removal,241 and postoperative CSF leak.242 Because greater blood loss and longer operations are associated with more complications (including infection), some surgeons stage longer operations; however, one study showed that malnourishment, hospital stay, and infection rates were all higher in patients with staged procedures.250 Length of hospital stay has been shown to be significantly longer in patients with a postoperative infection,241, 246 and the management of these complications is complex, especially in instrumented fusions.
Surgical site infections manifest with wound swelling, drainage, erythema, and dehiscence. The most common organism to cause postoperative spinal infections is S. aureus (49% of cases), followed by Staphylococcus epidermidis (28%); gram-negative organisms and mixed gram-positive and gram-negative infections each constitute 8% of surgical site infections in spine.123 Any discharge should be cultured to direct antibiotic therapy. Fever, elevated erythrocyte sedimentation rate, and elevated C-reactive protein are also nonspecific indications of a potential wound infection. CT and MRI might not be helpful because it is rarely possible to distinguish between a sterile seroma and a postoperative abscess. Broad-spectrum antibiotics should be started immediately (with coverage of both gram-positive and gram-negative organisms), nutrition supplemented, and the wound débrided and irrigated in the operating room, including the deep subfascial layer (except when there is a clearly delineated superficial infection).123,245 Aerobic, anaerobic, and fungal cultures of the wound should be obtained in the operating room. Consultation with plastic surgery may be warranted in severe cases, because paraspinous muscle flaps have been described with good control of infection.251
In cases where instrumentation has been used, the decision to remove hardware should be left up to the surgeon, because there are no clear guidelines in the literature. If intraoperatively it is clear that a successful arthrodesis has already occurred, then often the instrumentation can be removed; additionally, in cases requiring multiple débridements, removal of instrumentation should be considered. Closed-suction continuous irrigation systems have also been placed during débridement, with excellent results reported247,252; likewise, the successful treatment of infections using vacuum-assisted wound closure has also been described after instrumented spinal fusion.253 Many cases have been successfully treated with the hardware remaining in place.244,247–248,251 Intravenous antibiotics are generally continued as dictated by any culture results and in conjunction with consultation with infectious disease specialists. Superficial infections in cases without instrumentation might require no more than 2 weeks of antibiotics, but more-severe cases can require 12 weeks of intravenous antibiotic therapy; some surgeons continue lifetime oral antibiotic prophylaxis in instrumented cases where there has been a surgical site infection.
Venous thromboembolic events are common surgical complications; spine surgeons might hesitate to use prophylactic anticoagulation owing to the risk of hematoma formation. There are no evidence-based guidelines for deep vein thrombosis prophylaxis after spine surgery. There have been few reports on the rates of thromboembolic complications after fusion, but one study looking at fusion for degenerative spondylolisthesis from the NIS database found the risk to be 0.6%, although this might also be an underestimation owing to the reliance on discharge coding.122 A case of a deep venous thrombosis due to compression of the left common iliac vein from anterior migration of a fragment of bone graft has been reported, requiring a second operation for removal of the migrated bone graft.254
Loss of vision after spine surgery is a devastating complication that has received significant attention.255–262 The most common cause for perioperative vision loss after spine surgery is ischemic optic neuropathy,262 although central retinal artery occlusion and cortical blindness have also been described.259 Preoperative risk factors include peripheral vascular disease, hypertension, diabetes, and smoking; perioperative risk factors include prone positioning, long operative times, hypotension, anemia, and blood transfusion.263 However, vision loss can also occur in the absence of these risk factors.260 Although it is a devastating complication, a recent publication evaluating 4.7 million spinal procedures in the United States between 1993 and 2002 from the NIS database found the incidence to only be 0.094% (1:10,000). Risk factors identified in this national database included age younger than 18 years, age older than 84 years, peripheral vascular disease, hypertension, transfusion, intraoperative hypotension, and anemia. Recommendations to minimize the risk of sudden vision loss after spinal surgery include avoiding direct pressure on the globes, perioperative hypotension, and anemia; considering 10 degrees of reverse Trendelenburg during prone surgery; considering transfusing to keep the hematocrit above 30% in high risk patients; keeping mean arterial pressures close to the patient’s baseline; and performing an early postoperative vision exam.263
Rhabdomyolysis has been described after posterior spinal fusion surgery, manifesting with hypotension and acute renal failure.264–266 Despite the use of a Jackson frame, these cases occurred in obese patients, hypothesized to be secondary to pressure on muscles and the abdominal wall from positioning. Thus, when placing a patient prone before spinal surgery—especially an obese patient or before a long procedure—it is important to avoid excessive muscle pressure.265 Transverse myelitis has also been described after spinal fusion surgery, possibly resulting from a vascular etiology or transient spinal cord ischemia, and should be treated with high-dose corticosteroids.267
Sacral fractures in patients with osteoporosis who had a prior lumbar fusion have been reported in the literature.268–274 Although only 30 patients have been described, it is likely that this is a much more common entity that is often misdiagnosed.268 Patients have typically been female, elderly, undergoing multilevel fusions, and with a history of osteoporosis.268 Presenting as early as 4 weeks and as late as 8 months postoperatively, patients have described continued low back and gluteal pain, which is easy to confuse with a lack of improvement from the operation. Diagnosis has been made by plain x-ray.269 Although many patients have been successfully treated with bracing and medical management of osteoporosis, some required surgical stabilization.270
Long-Term Complications from Fusion Operations
Adjacent Segment Disease
The fact that intervertebral discs rostral and caudal to the fusion segment are at increased risk for subsequent herniation and/or degeneration has been known for many years (Fig. 166-19).275 Radiographic degenerative disc disease, including herniation, is more common than clinical radiculopathy and low back pain.276 In one study, 62.5% of patients had radiographic adjacent segment disease, but no significant differences in clinic outcomes were seen.277 There is a wide range in the published rates of adjacent segment disease, and another study found only 1.3% of patients followed for 8 years had clinically significant adjacent segment disease.278 However, with operation rates for symptomatic disc herniation ranging from 7.7% to 36.1%, it is clear that adjacent segment disease is not an isolated radiologic phenomenon.279–280 Other studies have shown that patients with adjacent segment disease have significantly worse outcomes.281–282 Multiple level fusions have a higher rate of adjacent segment disease than do single level fusions;280, 283 however, each level has a differential propensity for adjacent segment disease.275,281 Age is another risk factor.282 A greater degree of concomitant decompression, in addition to fusion, especially a simultaneous laminectomy, also predisposes patients to adjacent disc disease.284–285 Symptomatic disc herniation can occur as soon as months after and as late as 29 years after the initial operation.275
Two excellent studies merit closer evaluation. A prospective randomized, controlled trial was recently published examining the natural history of adjacent segment disease. In this study, 111 patients with isthmic spondylolisthesis were randomized to exercise, posterolateral fusion with instrumentation, or fusion without instrumentation. The average follow-up time was 12.6 years. No changes were seen in the adjacent discs of patients randomized to exercise; 38% of patients who underwent surgical interventions had evidence of adjacent segment disease by radiography. Patients who also had a laminectomy with the fusion had a significantly higher rate of adjacent level disease. There was no statistically significant difference in clinical outcomes between patients who had adjacent segment disease from those who did not.284 Another study examined the rates of reoperation for adjacent segment disease in the lumbar spine: 5 years and 10 years after the index operation, 16.5% and 36.1% of patients (respectively) had additional surgery. The rates of adjacent segment disease varied substantially by level, from a prevalence of 8.2% at L2–3, 14.7% at L3–4, 21.8% at L4–5, and 7.2% at L5–S1.279
Some surgeons have studied if modifications to posterolateral fusion can decrease the risk of adjacent level disease; however, preliminary studies show that there is unlikely to be any difference in adjacent level disease between posterolateral fusion and dynamic stabilization with Dyneys.286
Pseudarthrosis
The correlation between clinical outcomes and rates of successful arthrodesis is, surprisingly, quite low. This has led some to conclude that pseudarthrosis is, in fact, not a complication if successful postoperative clinical outcomes are achieved. However, there is ample evidence that patients with pseudarthrosis have significantly poorer clinical outcomes compared to patients in whom successful union is seen radiologically.34 Thus, it is important to regard pseudarthrosis as a nondesirable complication of operations, and preoperative planning should be achieved to optimize the likelihood of a successful fusion. Numerous trials have shown that fusion rates are higher in patients in whom transpedicular instrumentation is placed during their fusion operations.12,29 The evidence that transpedicular instrumentation directly improves outcomes is limited, but until superior methods are found to achieve high rates of arthrodesis, fusion operations should, when possible and clinically indicated, be supplemented with instrumentation.15,35
Chronic Donor Site Pain
Much has been written about chronic donor pain site from iliac crest bone graft harvesting.287–293 Pain alleviation is a goal of many lumbar spinal fusions, and thus surgeons are understandably hesitant to perform a procedure that will cause additional pain. The reluctance to use iliac crest bone graft, although it remains the gold standard for bone graft in spinal fusion, has been a major impetus behind the development of alternative agents, including BMP. Some have suggested that the degree of donor site pain is overestimated because the majority of patients who undergo fusion have chronic low back pain, and thus may be unable to distinguish between this pain and donor site pain, but the same authors reported that 14.3% of trauma patients had donor site pain—certainly not an inconsequential percentage.291 Another prospective study found that 12 months after the initial surgery, 16.5% of patients reported severe pain and 29.1% reported numbness at the donor site; 15.2% said this pain limited ambulation, and 5.2% reported that it limited employment.292 The impact of donor site pain can be substantial.
The use of local anesthetics to alleviate donor site pain has been described. Sacroiliac blocks with lidocaine have been successful.287 A prospective double-blinded, randomized, controlled trial evaluating a continuous-infusion catheter placed at the site of iliac crest bone graft harvesting delivering bupivacaine (Marcaine) (versus saline in the placebo group) show that patients in the treatment group had a statistically significant better pain score and satisfaction with the procedure; additionally, no patient in the treatment group reported chronic pain from the donor site.289 Larger cohorts are needed before this device becomes more commonly used, because the placement of an infusion catheter and a continuous administration of local anesthetic are not without additional risks.
Sexual Dysfunction
Autonomic nerve dysfunction causing retrograde ejaculation is a well-known complication of anterior spinal approaches, especially ALIF, and it is important to discuss this with patients because it can ultimately impair fertility.2 However, overall sexual function has not been thoroughly studied after fusion procedures. Berg and colleagues published a study showing that postoperatively, both men and women describe improvement in their sex lives, which is strongly correlated with relief of low back pain; however, there was a significantly increased rate of inability to achieve orgasm in men treated with posterolateral fusion or PLIF (26%) as compared to men treated with total disc replacement (4%).294
Heterotopic Ossification from Bone Morphogenetic Proteins
Pilot studies found BMP to be quite safe.151 However, BMP has been used much more extensively recently, and thus a better understanding of potential complications has arisen. About 320,000 patients from the NIS undergoing fusion procedures with adjunct BMP were examined and a higher rate of complications was seen in the cervical spine, primarily related to voice and swallowing212 (which have been described previously by other authors).295 However, no significant difference in the rate of complications was seen in the lumbar spine.212
The primary concern with the use of BMP is heterotopic ossification. The extent of undesired spread of BMP can be partially limited by the use of fibrin glue.296–297 However, cases of heterotopic ossification have been reported, including a patient with extensive ossification of his psoas and iliacus muscles, causing significant pain. Ossification was detectable by 3 months postoperatively and was largely complete by 6 months.298 Potentially more concerning is epidural heterotopic ossification. One study of 33 patients undergoing PLIF or TLIF with or without supplemental rhBMP-2 showed that epidural bone formation occurred in 20.8% of patients treated with BMP as compared to 8.3% in those who were not.299 Although there were no clinically detectable complications from the epidural ossification in this study, neurologic deficits have been documented by others, and revision surgery was difficult.300
Vertebral osetolysis has also been reported with the use of BMP-2 after PLIF, which resolved spontaneously with conservative management; however, this has not been reported after posterolateral fusion and might relate to violation of the end plate.301 Immune responses are known to occur in patients against a range of recombinant proteins, including rhBMP-2. Complications from antibody formation have not been reported, and there is no difference in outcomes in patients who mount an immune response, but further research will determine if there are any long-term complications.302
Postoperative Bracing
Postoperative lumbar bracing as an adjunct after fusion surgery is common, although controversy exists over its best use.303 Goals of adjunct bracing therapy include limiting mobility, aiding in the relief of pain postoperatively, and improving fusion rates and functional outcomes. One initial study suggested that fusion rates were higher in patients treated with a brace for 5 months.304 However, there is no other evidence that orthoses improve fusion rates or outcomes. One prospective study randomized patients to instrumented posterolateral fusion with or without orthosis for 8 weeks full time versus no intervention, and no difference was seen in fusion rates, outcomes, or reoperation rates at a number of time points (followed to 2 years).305
Despite the lack of evidence, a questionnaire study of surgeons attending a spine conference in January 2008 showed that 49% of surgeons routinely use bracing after lumbar spine operations. Bracing was typically used for 3 to 8 weeks, and the most common reason surgeons gave for using them was “restriction of patient activity.”306 The decision to use postoperative bracing should be left to the discretion of the surgeon.
Radiologic Assessment of Successful Fusion
Radiologic assessment of arthrodesis is not perfect. It is a common primary or secondary endpoint in clinical trials, and it is used clinically to assess successful fusion, but even fine-cut axial CT scans have limitations. Studies that examine the ability of imaging to assess fusion should use surgical exploration as the gold standard.307 One initial study showed that correlation rates between x-ray and surgical exploration were 64% to 69%; there is also relatively low inter-reader reliability (κ = 0.4–0.7).308 Another study found the sensitivity and specificity of x-ray to detect fusion to be 85% and 62%, respectively; the positive predictive value and negative predictive value were 76% and 54%, respectively.309 Obtaining routine radiographs postoperatively might not change the management of patients, although many surgeons, including the authors of this chapter, do so.310
Flexion-extension radiography may be a good initial study, especially if CT is not available. The correlation between flexion-extension x-rays and surgical re-exploration to detecting arthrodesis has been shown to be 62%, the sensitivity is 37%, specificity is 96%, positive predictive value is 70%, and negative predictive value is 86%.307–308 Although 5 degrees of angular motion between vertebrae is the consensus for the maximum degree after successful fusion, this may be excessively limited: Recent data suggest that up to 6.0 degrees of angular motion may be consistent with arthrodesis after posterolateral fusion procedures, and up to 6.9 degrees might not be inconsistent with fusion after PLIF.311
CT scanning is the best technique to assess fusion. Only one study has compared preoperative CT scans to surgical exploration in patients undergoing reoperation. The rate of inter-reader reliability was moderate (κ = 0.42–0.62). The accuracy of CT to detect posterolateral fusion was 89%, and when fusion involved both the facets and the posterolateral gutters, then the accuracy increased even more to 96%.312
Any rod, plate, or screw fracture or dislodgement should be diligently assessed on radiographs or CT scan, because this is highly predictive of a true pseudarthrosis.313 The presence of radiolucent zones around pedicle screw fixation, known colloquially as “clear zones” or the “halo sign,” should also be evaluated (Fig. 166-20). Although the positive predictive value of a pseudarthrosis if a clear zone is seen at 6 months is only 50%, the presence of persistent clear zone more than 2 years after the initial operation is highly suggestive of a pseudarthrosis.314
Determining accurate placement of instrumentation is distinct from assessing fusion, and it may be crucial in the immediate postoperative period. One study compared the results of radiographs and CT to anatomic dissection in assessing the correct placement of transpedicular screws in cadavers. The sensitivity of radiographs, CT, and combined was 93%, 94%, and 99%, respectively; the specificity was 13%, 37%, and 41%, respectively; the positive predictive value was 83%, 88%, and 90%, respectively; the negative predictive value was 31%, 56%, and 85% respectively; the accuracy was 79%, 84%, and 90% respectively.315 Yet another study evaluated the ability of imaging modalities to detect correct transpedicular screw placement in cadavers, finding the sensitivity and specificity of x-rays to be 70% and 83%, respectively and for CT 85% and 90%, respectively. Risk factors for inaccurate assessment included level (with L1 being significantly more likely to be incorrect) and stainless steel screws, because titanium screws produce less artifact.316 These studies suggest that routine x-rays may be a good initial study to assess the integrity of screw placement, with CT scan reserved for indeterminate cases or cases where the clinical and radiographic assessments do not agree. CT, however, may be substantially better at diagnosing the accurate placement of interbody cages than radiographs.317
Other imaging modalities have been assessed for their ability to detect arthrodesis. Scintigraphy using the tracer Tc-99m has been investigated; however, because of the low sensitivity and positive predictive value, it is not recommended for routine assessment of fusion.318 Despite the use of newer sequences such as spin-echo to reduce artifact from titanium screws, MRI is not recommended for assessment of screw placement or fusion.8 MRI might have a role in the assessment of spinal instability, because lumbar facet fluid seen on MRI has a positive predictive value greater than 80% for instability, especially at L4–L5.319
Economics of Lumbar Spinal Fusion
Fusion rates have been increasing rapidly in the United States since the 1970s.320–321 Between 1979 and 1987, the rates of lumbar fusion increased by 200%,320 and this trend has been continuing. Data from the NIS database showed that between 1990 and 2001 there was also a 220% increase in the rates of fusion, although that increase was only 113% between 1996 and 2001.322 During this time there has been a slight increase in total spine surgeries, and rates of inpatient spinal surgeries have remained stable (as 26% of discectomies performed in 2000 were outpatient cases, which was uncommon in 1990), indicating that the percentage of spinal operations that involve a fusion has increased substantially.323
The most rapid increases in fusion rates in the 1990s were seen in patients aged 60 year and older.322 In fact, among Medicare enrollees, rates of fusion have increased from 0.3 per 1000 in 1992 to 1.1 per 1000 in 2003, and spending for lumbar fusion increased by 500% during this time period.324 The average hospital bill for a spinal fusion procedure, excluding professional fees, was $34,000 in 2004;98 more than 300,000 spinal fusions were performed that year, and the total cost was more than $16 billion in hospital charges.99
Wide geographic variations also have been reported in spinal operations, especially fusion.237,324–326 The United States has the highest rate of spinal operations, which is five times greater than in England and Scotland and double that of many other countries including Australia, Canada, and many Scandinavian countries. Rates within the United States also vary substantially by state, from 1.8 per 1000 Medicare enrollees in Hawaii to 9.2 per 1000 Medicare enrollees in Wyoming in 2001.325 Geographic variations in fusion rates are even higher, with a 20-fold range among Medicare enrollees in 2002 and 2003, which is the largest coefficient of variation seen with any surgical procedure in this population.324 There might also be a minor variation in complications and costs of spinal fusion procedures within the United States, because a significantly higher rate of cauda equina syndrome, but shorter hospital length of stay and higher charges, have been reported in the South and the West as compared to the Northeast and the Midwest.237
Along with the increase seen in fusion procedures, there has been a concomitant increase in the use of spinal instrumentation and BMP. The market for spinal implants was estimated in 2004 to be $2 billion per year, with an annual growth rate of 18% to 20%.98 Similarly, data from the NIS database showed that the rate of BMP use has increased from 0.69% of fusions in 2002 to 24.89% of fusions in 2006, and variations also exist within BMP use, with higher use in women, European Americans, and Medicare patients. The use of BMP is associated with an increased hospital cost that varies by procedure type, from 11% to 41%.212
However, some have argued that the increase in fusion is partially driven by differences in physician reimbursement, because reimbursement is higher for fusion procedures. A recently published economic model suggests the opposite: Fusion procedures were found to be associated with a greater number of postoperative visits, a higher mean total clinical time, and lower mean dollars per minute.327
Future Directions In Lumbar Spinal Fusion
Animal models were first used to show the ability of BMP to promote spinal fusion, and now, more than a decade after these initial studies, BMP is being used in one quarter of spinal fusions in the United States.212 Current research using animal models gives insight into potential future directions in spinal fusion.
The use of gene therapy has been investigated in animal models of fusion. Implantation of bone marrow cells transfected with a lentivirus containing BMP-2 has been shown to augment posterolateral fusion in a rat model.328 Although these results are promising, there are many barriers to use in humans, including the best delivery vector, the effective dose, transduction time, and gene transfer method.329
The ability of adipose-derived stem cells to promote osteogenesis is well known, but only recently have they been shown to increase rates of fusion in a rat model (compared to fusion rates with a scaffold).330 Using a combination of both approaches, another group showed the ability of adipose-derived tissue stem cells nucleofected with recombinant BMP-6 to induce fusion in rats.331
Conclusions
Fusion of the lumbar spine has made significant advances since Albee and Hibbs in 1911. Now it is understood that there are a number of distinct pathologic types of degenerative conditions, including degenerative spondylolisthesis with spinal stenosis, spondylolysis, spinal stenosis without spondylolisthesis, herniated lumbar discs, and chronic axial low back pain of an unknown etiology. Each of these conditions should be evaluated separately, because their indications for surgery differ (see Table 166-2).
Andersen T., Christensen F.B., C CE, et al. The effect of electrical stimulation on lumbar spinal fusion in older patients: A randomized, controlled, multi-center trial: Part 1: functional outcome. Spine. 2009;34:2241-2247.
Atlas S.J., Keller R.B., Robson D., et al. Surgical and nonsurgical management of lumbar spinal stenosis: Four-year outcomes from the Maine Lumbar Spine Study. Spine. 2000;25:556-562.
Brox J.I., Soerensen R., Friis A., et al. Randomized clinical trial of lumbar instrumented fusion and cognitive intervention and exercises in patients with chronic low back pain and disc degeneration. Spine. 2003;28:1913-1921.
Cahill K.S., Chi J.H., Day A., et al. Prevalence, complications and hospital charges associated with use of bone-morphogenetic proteins in spinal fusion procedures. JAMA. 2009;302:58-66.
Carreon L.Y., Dijurasovic M., Glassman S.D., et al. Diagnostic accuracy and reliability of fine-cut CT scans with reconstructions to determine the status of an instrumented posterolateral fusion with surgical exploration as reference standard. Spine. 2007;32:892-895.
Ekman P., Moller H., Shalabi A., et al. A prospective randomized study on the long-term effect of lumbar fusion on adjacent disc degeneration. Eur Spine J. 2009;18:1175-1186.
Fairbank J., Frost H., Wilson-MacDonald J., et al. Randomised controlled trial to compare surgical stabilization of the lumbar spine with an intensive rehabilitation programme for patients with chronic low back pain: The MRC spine stabilization trial. BMJ. 2005;330:1233.
Fritzell P., Hagg O., Wessberg P., et al. 2001 Volvo Award Winner in Clinical Studies: Lumbar fusion versus nonsurgical treatment for chronic low back pain: A multicenter randomized controlled trial from the Swedish Lumbar Spine Study Group. Spine. 2001;26:2521-2534.
Ghiselli G., Wang J.C., Bhatia N.N., et al. Adjacent segment degeneration in the lumbar spine. J Bone Joint Surg Am. 2004;86:1497-1503.
Glassman S.D., Anagnost S.C., Parker A., et al. The effect of cigarette smoking and smoking cessation on spinal fusion. Spine. 2000;15:2608-2615.
Ibrahim T., Tleyjeh I.M., Gabbar O. Surgical versus non-surgical treatment of chronic low back pain: A meta-analysis of randomized trials. Int Orthop. 2008;32:107-113.
Kalanithi P.S., Patil C.G., Boakye M. National complication rates and disposition after posterior lumbar fusion for acquired spondylolisthesis. Spine. 2009;34:1963-1969.
Kim H.J., Lee H.W., Kim H.S., et al. Life expectancy after lumbar spine surgery: One- to eleven-year follow-up of 1015 patients. Spine. 2008;33:2116-2121.
Kornblum M.B., Fischgrund J.S., Herkowitz H.N., et al. Degenerative lumbar spondylolisthesis with spinal stenosis: A prospective long-term study comparing fusion and pseudoarthrosis. Spine. 2004;29:726-734.
Malmivaara A., Slatis P., Heliovaara M., et al. Surgical or non-operative treatment for lumbar spinal stenosis? A randomized controlled trial. Spine. 2007;32:1-8.
Moller H., Hedlund R. Surgery versus conservative management in adult isthmic spondylolisthesis. Spine. 2000;25:1711-1715.
Papakostidis C., Kontakis G., Bhandari M., et al. Efficacy of autologous iliac crest bone graft and bone morphogenetic protein for posterolateral fusion of lumbar spine: A meta-analysis of the results. Spine. 2008;33:E680-E692.
Quinones-Hinojosa A., Jun P., Jacobs R., et al. General principles in the medical and surgical management of spinal infections: A multidisciplinary approach. Neurosurg Focus. 2004;17:E1.
Watters W.C., Baisden J., Bono C.M. Antibiotic prophylaxis in spine surgery: An evidence-based clinical guideline for the use of prophylactic antibiotics in spine surgery. Spine J. 2009;9:142-146.
Watters W.C., Baisden J., Gilbert T.J., et al. Degenerative lumbar spinal stenosis: An evidence-based clinical guideline for the diagnosis and treatment of degenerative lumbar spinal stenosis. Spine J. 2008;8:305-310.
Watters W.C., Bono C.M., Gilbert T.J., et al. An evidence-based clinical guideline for the diagnosis and treatment of degenerative lumbar spondylolisthesis. Spine J. 2009;9:609-614.
Weinstein J.N., Lurie J.D., Tosteson T.D., et al. Surgical versus nonsurgical treatment for lumbar degenerative spondylolisthesis. N Engl J Med. 2007;356:2257-2270.
Weinstein J.N., Tosteson T.D., Lurie J.D., et al. Surgical versus nonsurgical therapy for lumbar spinal stenosis. N Engl J Med. 2008;358:794-810.
Weinstein J.N., Tosteson T.D., Lurie J.D., et al. Surgical vs non-operative treatment for lumbar disk herniation: The Spine Patient Outcomes Research Trial (SPORT): A randomized trial. JAMA. 2006;296:2441-2450.
Yee A.J., Yoo J.U., Marsolais E.B., et al. Use of postoperative lumbar corset after lumbar spinal arthrodesis for degenerative conditions of the spine: A prospective randomized trial. J Bone Joint Surg Am. 2008;90:2062-2068.
1. Wang J.C., Mummaneni P.V., Haid R.W. Current treatment strategies for the painful lumbar motion segment: posterolateral fusion versus interbody fusion. Spine. 2005;30:S33-S43.
2. Ogilvie J.W. Complications in spondylolisthesis surgery. Spine. 2005;30:S97-S101.
3. Hibbs R.A. An operation for progressive spinal deformities. N Y Med. 1911;121:1013.
4. Albee F.H. Transplantation of a portion of the tibia into the spine for Pott’s disease. JAMA. 1911;57:855.
5. Lipson S.J. Spinal-fusion surgery—advances and concerns. N Engl J Med. 2004;350:643-644.
6. Watkins M.B. Posterolateral fusion of the lumbar and lumbosacral spine. J Bone Joint Surg Am. 1953;35:1014-1018.
7. Harrington P.R. Treatment of scoliosis. Correction and internal fixation by spine instrumentation. J Bone Joint Surg Am. 1962;44:591-610.
8. Rutherford E.E., Tarplett T.L., Davies E.M., et al. Lumbar spine fusion and stabilization: hardware, techniques, and imaging appearances. Radiographics. 2007;27:1737-1749.
9. Newman P.H., Stone K.H. The etiology of spondylolisthesis. J Bone Joint Surg Br. 1963;45:39-59.
10. Meyerding H.W. Spondylolisthesis: surgical treament and results. Surg Gynecol Obstet. 1932;54:371-377.
11. Metz L.N., Deviren V. Low-grade spondylolisthesis. Neurosurg Clin N Am. 2007;18:237-248.
12. Martin C.M., Gruszcynski A.T., Braunsfurth H.A., et al. The surgical management of degenerative lumbar spondylolisthesis: a systematic review. Spine. 2007;32:1791-1798.
13. Majid K., Fischgrund J.S. Degenerative lumbar spondylolisthesis: trends in management. J Am Acad Orthop Surg. 2008;16:208-215.
14. Watters W.C., Bono C.M., Gilbert T.J., et al. An evidence-based clinical guideline for the diagnosis and treatment of degenerative lumbar spondylolisthesis. Spine J. 2009;9:609-614.
15. Herkowitz H.N. Degenerative lumbar spondylolisthesis: evolution of surgical management. Spine J. 2009;9:605-606.
16. Weinstein J.N., Lurie J.D., Tosteson T.D., et al. Surgical versus nonsurgical treatment for lumbar degenerative spondylolisthesis. N Engl J Med. 2007;356:2257-2270.
17. Weinstein J.N., Lurie J.D., Tosteson T.D., et al. Surgical compared with non-operative treatment for lumbar degenerative spondylolisthesis. J Bone Joint Surg. 2009;91:1295-1304.
18. Matsudaira K., Yamazaki T., Seichi A., et al. Spinal stenosis in grade I degenerative lumbar spondylolisthesis: a comparative study of outcomes following laminoplasty and laminectomy with instrumented spinal fusion. J Orthop Sci. 2005;10:270-276.
19. Kristof R.A., Aliashkevich A.F., Schuster M., et al. Degenerative lumbar spondylolisthesis–induced radicular compression: nonfusion-related decompression in selected patients without hypermobility on flexion-extension radiographs. J Neurosurg. 2002;97:281-286.
20. Resnick D.K., Choudhri T.F., Dailey A.T., et al. Guidelines for the performance of fusion procedures for degenerative disease of the lumbar spine. Part 9: fusion in patients with stenosis and spondylolisthesis. J Neurosurg Spine. 2005;2:679-685.
21. Martin B.I., Mirza S.K., Comstock B.A., et al. Reoperation rates following lumbar spine surgery and the influence of spinal fusion procedures. Spine. 2007;32:382-387.
22. Herkowitz H.N., Kurz L.T. Degenerative lumbar spondylolisthesis with spinal stenosis. A prospective study comparing decompression with decompression and intertransverse process arthrodesis. J Bone Joint Surg Am. 1991;73:802-808.
23. Ghogawal Z., Benzel E.C., Amin-Hanjani S., et al. Prospective outcomes evaluation after decompression with or without instrumented fusion for lumbar stenosis and degenerative grade I spondylolisthesis. J Neurosurg Spine. 2004;3:267-272.
24. Bednar D.A. Surgical management of lumbar degenerative spinal stenosis with spondylolisthesis via posterior reduction with minimal laminectomy. J Spinal Disord Tech. 2002;15:105-109.
25. Nork S.E., Hu S.S., Workman K.L., et al. Patient outcomes after decompression and instrumented posterior spinal fusion for degenerative spondylolisthesis. Spine. 1999;24:561-569.
26. Park Y.K., Kim J.H., Oh J.I., et al. Facet fusion in the lumbosacral spine: a 2-year follow-up study. Neurosurgery. 2002;51:88-96.
27. Rousseau M.A., Lazennec J.Y., Bass E.C., et al. Predictors of outcomes after posterior decompression and fusion in degenerative spondylolisthesis. Eur Spine J. 2005;14:55-60.
28. Zagra A., Giudici F., Minoia L., et al. Long-term results of pediculo-body fixation and posterolateral fusion for lumbar spondylolisthesis. Eur Spine J. 2009;18:S151-S155.
29. Fischgrund J.S., Mackay M., Herkowitz H.N., et al. Degenerative lumbar spondylolisthesis with spinal stenosis: a prospective, randomized study comparing decompressive laminectomy and arthrodesis with and without spinal instrumentation. Spine. 1997;22:2807-2812.
30. Christensen F.B., Hansen E.S., Laursen M., et al. Long-term functional outcome of pedicle screw instrumentation as a support for posterolateral spinal fusion: randomized clinical study with a 5-year follow-up. Spine. 2002;27:1269-1277.
31. Mochida J., Suzuki K., Chiba M. How to stabilize a single level lesion of degenerative lumbar spondylolisthesis. Clin Orthop Relat Res. 1999;368:126-134.
32. Thomsen K., Christensen F.B., Eiskjaer S.P., et al. The effect of pedicle screw instrumentation on functional outcome and fusion rates in posterolateral lumbar spinal fusion: a prospective, randomized clinical study. Spine. 1997;22:2813-2822.
33. Fernandez-Fairen M., Sala P., Ramirez H., et al. A prospective randomized study of unilateral versus bilateral instrumented posterolateral lumbar fusion in degenerative spondylolisthesis. Spine. 2007;32:395-401.
34. Kornblum M.B., Fischgrund J.S., Herkowitz H.N., et al. Degenerative lumbar spondylolisthesis with spinal stenosis: a prospective long-term study comparing fusion and pseudoarthrosis. Spine. 2004;29:726-734.
35. Fischgrund J.S. The argument for instrumented decompressive posterolateral fusion for patients with degenerative spondylolisthesis and spinal stenosis. Spine. 2004;29:173-174.
36. Phillips F.M. The argument for noninstrumented posterolateral fusion for patients with spinal stenosis and degenerative spondylolisthesis. Spine. 2004;29:170-172.
37. Resnick D.K., Choudhri T.F., Dailey A.T., et al. Guidelines for the performance of fusion procedures for degenerative disease of the lumbar spine. Part 12: pedicle screw fixation as an adjunct to posterolateral fusion for low back pain. J Neurosurg Spine. 2005;2:700-706.
38. McAfee P.C., DeVine J.G., Chaput C.D., et al. The indications for interbody fusion cages in the treatment of spondylolisthesis: analysis of 120 cases. Spine. 2005;30:S60-S65.
39. Ha K.Y., Na K.H., Shin J.H., et al. Comparison of posterolateral fusion with and without additional posterior lumbar interbody fusion for degenerative lumbar spondylolisthesis. J Spinal Disord Tech. 2008;21:229-234.
40. Hosono N., Namekata M., Makino T., et al. Perioperative complications of primary posterior lumbar interbody fusion for nonisthmic spondylolishtesis: analysis of risk factors. J Neurosurg Spine. 2008;9:403-407.
41. Lauryssen C. Appropriate selection of patients with lumbar spinal stenosis for interspinous process decompression with the X STOP device. Neurosurg Focus. 2007;22:E5.
42. Bono C.M., Vaccaro A.R. Interspinous process devices in the lumbar spine. J Spinal Disord Tech. 2007;20:255-261.
43. Anderson P.A., Tribus C.B., Kitchel S.H. Treatment of neurogenic claudication by interspinous decompression: application of the X-Stop device in patients with lumbar degenerative spondylolisthesis. J Neurosurg Spine. 2006;4:464-471.
44. Schaeren S., Broger I., Jeanneret B. Minimum four-year follow-up of spinal stenosis with degenerative spondylolisthesis treated with decompression and dynamic stabilization. Spine. 2008;33:E636-E642.
45. Verhoof O.J., Bron J.L., Wapstra F.H., et al. High Failure rate of the interspinous distraction device (X-Stop) for the treatment of lumbar spinal stenosis caused by degenerative spondylolisthesis. Eur Spine J. 2008;17:188-192.
46. Acosta F., Ames C., Chou D. Operative management of adult high-grade lumbosacral spondylolisthesis. Neurosurg Clin N Am. 2007;18:249-254.
47. Sailhan F., Gollogly S., Roussouly P. The radiographic results and neurologic complications of instrumented reduction and fusion of high-grade spondylolisthesis without decompression of the neural elements: a retrospective review of 44 patients. Spine. 2006;31:161-169.
48. DeWald C.J., Vartabedian J.E., Rodts M.F., et al. Evaluation and management of high-grade spondylolisthesis in adults. Spine. 2005;30:S49-S59.
49. Jones T.R., Rao R.D. Adult isthmic spondylolisthesis. J Am Acad Orthop Surg. 2009;17:609-617.
50. Floman Y., Milgram M.A., Ashkenazi E., et al. Instrumented slip reduction and fusion for painful unstable isthmic spondylolisthesis in adults. J Spinal Disord Tech. 2008;21:477-483.
51. Moller H., Hedlund R. Surgery versus conservative management in adult isthmic spondylolisthesis. Spine. 2000;25:1711-1715.
52. Ekman P., Moller H., Hedlund R. The long-term effect of posterolateral fusion in adult isthmic spondylolisthesis: a randomized, controlled study. Spine J. 2005;5:36-44.
53. Ekman P., Moller H., Hedlund R. Predictive factors for the outcome of fusion in adult isthmic spondylolisthesis. Spine. 2009;34:1204-1210.
54. Wenger M., Sapio N., Markwalder T.M. Long-term outcome in 132 consecutive patients after posterior internal fixation and fusion for grade I and II isthmic spondylolisthesis. J Neurosurg Spine. 2005;2:289-297.
55. Lamberg T.S., Remes V.M., Helenius I.J., et al. Long-term clinical, functional and radiological outcome 21 years after posterior or posterolateral fusion in childhood and adolescence isthmic spondylolisthesis. Eur Spine J. 2005;14:639-644.
56. Moller H., Hedlund R. Instrumented and noninstrumented posterolateral fusion in adult spondylolisthesis: a prospective randomized study: part 2. Spine. 2000;25:1716-1721.
57. Kwon B.K., Hilibrand A.S., Malloy K., et al. A critical analysis of the literature regarding surgical approach and outcome for adult low-grade isthmic spondylolisthesis. J Spinal Disord Tech. 2005;18:S30-S40.
58. Suh K.T., Park W.W., Kim S.J., et al. Posterior lumbar interbody fusion for adult isthmic spondylolisthesis. J Bone Joint Surg Br. 2008;90:1352-1356.
59. Ekman P., Moller H., Tulberg T., et al. Posterior lumbar interbody fusion versus posterolateral fusion in adult isthmic spondylolisthesis. Spine. 2007;32:2178-2183.
60. Rosa G.L., Conti A., Cacciola F., et al. Pedicle screw fixation for isthmic spondylolisthesis: does posterior lumbar interbody fusion improve outcome over posterolateral fusion? J Neurosurg. 2003;99:143-150.
61. Madan S., Boeree N.R. Outcome of posterior lumbar interbody fusion versus posterolateral fusion for spondylolytic spondylolisthesis. Spine. 2002;27:1536-1542.
62. Swan J., Hurwitz E., Malek F., et al. Surgical treatment for unstable low-grade isthmic spondylolisthesis in adults: a prospective controlled study of posterior instrumented fusion compared with combined anterior-posterior fusion. Spine J. 2006;6:606-614.
63. Jacobs W.C., Vreeling A., Kleuver M.D. Fusion for low-grade adult isthmic spondylolisthesis: a systematic review of the literature. Eur Spine J. 2006;15:391-402.
64. Zak P.J. Surgical management of spinal stenosis. Phys Med Rehabil Clin N Am. 2003;14:143-155.
65. Watters W.C., Baisden J., Gilbert T.J., et al. Degenerative lumbar spinal stenosis: an evidence-based clinical guideline for the diagnosis and treatment of degenerative lumbar spinal stenosis. Spine J. 2008;8:305-310.
66. Sengupta D.K., Herkowitz H.N. Lumbar spinal stenosis: treatment strategies and indications for surgery. Orthop Clin North Am. 2003;34:281-295.
67. Resnick D.K., Choudhri T.F., Dailey A.T., et al. Guidelines for the performance of fusion procedures for degenerative disease of the lumbar spine. Part 10: fusion following decompression in patients with stenosis without spondylolisthesis. J Neurosurg Spine. 2005;2:686-691.
68. Malmivaara A., Slatis P., Heliovaara M., et al. Surgical or non-operative treatment for lumbar spinal stenosis? A randomized controlled trial. Spine. 2007;32:1-8.
69. Weinstein J.N., Tosteson T.D., Lurie J.D., et al. Surgical versus nonsurgical therapy for lumbar spinal stenosis. N Engl J Med. 2008;358:794-810.
70. Tosteson A.N., Lurie J.D., Tosteson T.D., et al. Surgical treatment of spinal stenosis with and without degenerative spondylolisthesis: cost-effectiveness after 2 years. Ann Intern Med. 2008;149:845-853.
71. Atlas S.J., Keller R.B., Robson D., et al. Surgical and nonsurgical management of lumbar spinal stenosis: four-year outcomes from the Maine Lumbar Spine Study. Spine. 2000;25:556-562.
72. Atlas S.J., Keller R.B., Wu Y.A., et al. Long-term outcomes of surgical and nonsurgical management of lumbar spinal stenosis: 8 to 10 year results from the Maine Lumbar Spine Study. Spine. 2005;30:936-943.
73. Katz J.N., Stucki G., Lipson S.J., et al. Predictors of surgical outcome in degenerative lumbar spinal stenosis. Spine. 1999;24:2229-2233.
74. Panagiotis Z.E., Athanasios K., Panagiotis D., et al. Functional outcome of surgical treatment for multi-level lumbar spinal stenosis. Acta Orthop Scand. 2006;77:670-676.
75. Gu Y., Chen L., Yang H.L., et al. Efficacy of surgery and type of fusion in patients with degenerative lumbar spinal stenosis. J Clin Neurosci. 2009;16:1291-1295.
76. Jolles B.M., Porchet F., Theumann N. Surgical treament of lumbar spinal stenosis: five-year follow-up. J Bone Joint Surg Br. 2001;83:949-953.
77. Katz J.N., Lipson S.J., Lew R.A., et al. Lumbar laminectomy alone or with instrumented or noninstrumented arthrodesis in degenerative lumbar spinal stenosis: patient selection, costs, and surgical outcomes. Spine. 1997;22:1123-1131.
78. Cornefjord M., Byrod G., Brisby H., et al. A long-term (4- to 12-year) follow-up study of surgical treatment of lumbar spinal stenosis. Eur Spine J. 2000;9:563-570.
79. Kim K.A., McDonald M., Pik J.H., et al. Dynamic intraspinous spacer technology for posterior stabilization: case-control study on the safety, sagittal angulation, and pain outcome at 1-year follow-up evaluation. Neurosurg Focus. 2007;22:E7.
80. Atlas S.J., Keller R.B., Wu Y.A., et al. Long-term outcomes of surgical and nonsurgical management of sciatica secondary to a lumbar disc herniation: 10 year results from the Maine Lumbar Spine Study. Spine. 2005;30:927-935.
81. Atlas S.J., Keller R.B., Chang Y., et al. Surgical and nonsurgical management of sciatica secondary to a lumbar disc herniation: five-year outcomes from the Maine Lumbar Spine Study. Spine. 2001;26:1179-1187.
82. Weinstein J.N., Tosteson T.D., Lurie J.D., et al. Surgical vs non-operative treatment for lumbar disk herniation: the Spine Patient Outcomes Research Trial (SPORT): a randomized trial. JAMA. 2006;296:2441-2450.
83. Weinstein J.N., Lurie J.D., Tosteson T.D., et al. Surgical vs non-operative treatment for lumbar disk herniation: the Spine Patient Outcomes Research Trial (SPORT) observational cohort. JAMA. 2006;296:2451-2459.
84. Weinstein J.N., Lurie J.D., Tosteson T.D., et al. Surgical versus non-operative treatment for lumbar disc herniation: four-year results for the Spine Patient Outcomes Research Trial (SPORT). Spine. 2008;33:2789-2800.
85. Padua R., Padua S., Romanini E., et al. Ten- to 15-year outcome of surgery for lumbar disc herniation: radiographic instability and clinical findings. Eur Spine J. 1999;8:70-74.
86. Gepstein R., Shabat S., Reichel M., et al. Treatment of postdiscectomy low back pain by percutaneous posterior lumbar interbody fusion versus open posterior lumbar fusion with pedicle screws. Spine J. 2008;8:741-746.
87. Resnick D.K., Choudhri T.F., Dailey A.T., et al. Guidelines for the performance of fusion procedures for degenerative disease of the lumbar spine. Part 8: lumbar fusion for disc herniation and radiculopathy. J Neurosurg Spine. 2005;2:673-678.
88. Takeshima T., Kambara K., Miyata S., et al. Clinical and radiographic evaluation of disc excision for lumbar disc herniation with and without posterolateral fusion. Spine. 2000;25:450-456.
89. Suk K.Y., Lee H.M., Moon S.H., et al. Recurrent lumbar disc herniation: results of operative management. Spine. 2001;26:672-676.
90. Fu T.S., Lai P.L., Tsai T.T., et al. Long-term results of disc excision for recurrent lumbar disc herniation with or without posterolateral fusion. Spine. 2005;30:2830-2834.
91. Fritzell P., Hagg O., Jonsson D., et al. Cost-effectiveness of lumbar fusion and nonsurgical treatment for chronic low back pain in the Swedish Lumbar Spine Study: a multicenter, randomized, controlled trial from the Swedish Lumbar Spine Study Group. Spine. 2004;29:421-423.
92. Allen R.T., Rihn J.A., Glassman S.D., et al. An evidence-based approach to spine surgery. Am J Med Qual. 2009;24:S15.
93. Ostelo R.W., van Tulder M.W., Vlaeyen J.W., et al. Behavioural treatment for chonic low back pain (review). Cochrane Database Syst Rev. 2009;25(1):CD002014.
94. Resnick D.K., Choudhri T.F., Dailey A.T., et al. Guidelines for the performance of fusion procedures for degenerative disease of the lumbar spine. Part 6: magnetic resonance imaging and discography for patient selection for lumbar fusion. J Neurosurg Spine. 2005;2:662-669.
95. Fritzell P., Hagg O., Wessberg P., et al. 2001 Volvo Award Winner in Clinical Studies: lumbar fusion versus nonsurgical treatment for chronic low back pain: a multicenter randomized controlled trial from the Swedish Lumbar Spine Study Group. Spine. 2001;26:2521-2534.
96. Fritzell P., Hagg O., Wessberg P., et al. Chronic low back pain and fusion: a comparison of three surgical techniques: a prospective multicenter randomized study from the Swedish Lumbar Spine Study Group. Spine. 2002;27:1131-1141.
97. Fritzell P., Hagg O., Nordwall A. Complications in lumbar fusion surgery for chronic low back pain: comparison of three surgical techniques used in a prospective randomized study. A report from the Swedish Lumbar Spine Study Group. Eur Spine J. 2003;12:178-189.
98. Deyo R.A., Nachemson A., Mirza S.K. Spinal-fusion surgery—the case for restraint. N Engl J Med. 2004;350:722-726.
99. Deyo R.A. Back surgery—who needs it? N Engl J Med. 2007;356:2239-2243.
100. Mirza S.K., Deyo R.A. Systematic review of randomized trials comparing lumbar fusion surgery to non-operative care for treatment of chronic back pain. Spine. 2007;32:816-823.
101. Kwon B., Katz J.N., Kim D.H., et al. A Review of the 2001 Volvo Award Winner in Clinical Studies: lumbar fusion versus nonsurgical treatment for chronic low back pain: a multicenter randomized controlled trial from the Swedish Lumbar Spine Study Group. Spine. 2006;31:245-249.
102. Brox J.I., Soerensen R., Friis A., et al. Randomized clinical trial of lumbar instrumented fusion and cognitive intervention and exercises in patients with chronic low back pain and disc degeneration. Spine. 2003;28:1913-1921.
103. Fairbank J., Frost H., Wilson-MacDonald J., et al. Randomised controlled trial to compare surgical stabilization of the lumbar spine with an intensive rehabilitation programme for patients with chronic low back pain: the MRC spine stabilization trial. BMJ. 2005;330:1233.
104. Wilson-MacDonald J., Fairbank J., Frost H., et al. The MRC Spine Stabilization Trial: surgical methods, outcomes, costs, and complications of surgical stabilization. Spine. 2008;33:2334-2340.
105. Ibrahim T., Tleyjeh I.M., Gabbar O. Surgical versus non-surgical treatment of chronic low back pain: a meta-analysis of randomized trials. Int Orthop. 2008;32:107-113.
106. Resnick D.K., Choudhri T.F., Dailey A.T., et al. Guidelines for the performance of fusion procedures for degenerative disease of the lumbar spine. Part 7: intractable low back pain without stenosis or spondylolisthesis. J Neurosurg Spine. 2005;2:670-672.
107. Chou R., Baisden J., Carragee E.J., et al. Surgery for low back pain: a Review of the evidence for an american pain society clinical practice guideline. Spine. 2009;34:1094-1109.
108. Kwon B.K., Vaccaro A.R., Grauer J.N., et al. Indications, techniques, and outcomes of posterior surgery for chronic low back pain. Orthop Clin North Am. 2003;34:297-308.
109. Lee M.C., Wang M.Y., Fessler R.G., et al. Instrumentation in patients with spinal infection. Neurosurg Focus. 2004;17:E7.
110. Quinones-Hinojosa A., Jun P., Jacobs R., et al. General principles in the medical and surgical management of spinal infections: a multidisciplinary approach. Neurosurg Focus. 2004;17:E1.
111. Ha K.Y., Shin J.H., Kim K.W., et al. The fate of anterior autogenous bone graft after anterior radical surgery with or without posterior instrumentation in the treatment of pyogenic lumbar spondylodiscitis. Spine. 2007;32:1856-1864.
112. Talu U., Gogus A., Ozturk C., et al. The role of posterior instrumentation and fusion after anterior radical débridement and fusion in the surgical treatment of spinal tuberculosis: experience of 127 cases. J Spinal Disord Tech. 2006;19:554-559.
113. Pee Y.H., Park J.D., Choi Y.G., et al. Anterior débridement and fusion followed by posterior pedicle screw fixation in pyogenic spondylodiscitis: autologous iliac bone strut versus cage. J Neurosurg Spine. 2008;8:405-412.
114. Karaeminoguillari O., Aydinli U., Ozerdemoglu R., et al. Tuberculosis of the lumbar spine: outcomes after combined treatment of two-drug therapy and surgery. Orthopedics. 2007;30:55-59.
115. Lee S.H., Sung J.K., Park Y.M. Single-stage transpedicular decompression and posterior instrumentation in treatment of thoracic and thoracolumbar spinal tuberculosis: a retrospective case series. J Spinal Disord Tech. 2006;19:595-602.
116. Guzey F.K., Emel E., Bas N.S., et al. Thoracic and lumbar tuberculous spondylitis treated by posterior débridement, graft placement, and instrumentation: a retrospective analysis in 19 cases. J Neurosurg Spine. 2005;3:450-458.
117. Bezer M., Kucukdurmaz F., Aydin N., et al. Tuberculous spondylitis of the lumbosacral region: long-term follow-up of patients treated by chemotherapy, transpedicular drainage, posterior instrumentation, and fusion. J Spinal Disord Tech. 2005;18:425-429.
118. Katonis P., Tzermiadianos M., Gikas A., et al. Surgical treatment of spinal brucellosis. Clin Orthop Relat Res. 2006;44:66-72.
119. Lu D.C., Wang V., Chou D. The use of allograft or autograft and expandable titanium cages for the treatment of vertebral osteomyelitis. Neurosurgery. 2009;64:122-130.
120. Allen R.T., Lee Y.P., Stimson E., et al. Bone morphogenetic protein-2 (BMP-2) in the treatment of pyogenic vertebral osteomyelitis. Spine. 2007;32:2996-3006.
121. O’Shaughnessy B.A., Kuklo R., Ondra S.L. Surgical treatment of vertebral osteomyelitis with recombinant human bone morphogenetic protein-2. Spine. 2008;33:E132-E139.
122. Kalanithi P.S., Patil C.G., Boakye M. National complication rates and disposition after posterior lumbar fusion for acquired spondylolisthesis. Spine. 2009;34:1963-1969.
123. Beiner J.M., Grauer J., Kwon B.K., et al. Postoperative wound infections of the spine. Neurosurg Focus. 2003;15:A14.
124. Brookfield K.F., Brown M.D., Henriques S.M., et al. Allogeneic transfusion after predonation of blood for elective spine surgery. Clin Orthop Rel Res. 2008;466:1949-1953.
125. Lee T.C., Ueng S.W., Chen H.H., et al. The effect of acute smoking on spinal fusion: an experimental study among rabbits. J Trauma. 2005;59:402-408.
126. Theiss S.M., Boden S.D., Hair G., et al. The effect of nicotine on gene expression during spine fusion. Spine. 2000;20:2588-2594.
127. Glassman S.D., Anagnost S.C., Parker A., et al. The effect of cigarette smoking and smoking cessation on spinal fusion. Spine. 2000;15:2608-2615.
128. Andersen T., Christensen F.B., Laursen M., et al. Smoking as a predictor of negative outcome in lumbar spinal fusion. Spine. 2001;26:2623-2628.
129. Glassman S.D., Dimar J.R., Burkus K., et al. The efficacy of rhBMP-2 for posetolateral lumbar fusion in smokers. Spine. 2007;32:1693-1698.
130. Ogden C.L., Carroll M.D., Curtin L.R., et al. Prevalence of overweight and obesity in the United States, 1999-2004. JAMA. 2006;295:1549-1555.
131. Glassman S.D., Alegre G., Carreon L., et al. Perioperative complications of lumbar instrumentation and fusion in patients with diabetes mellitus. Spine J. 2003;3:496-501.
132. Bendo J.A., Spivak J., Moskovich R., et al. Instrumented posterior arthrodesis of the lumbar spine in patients with diabetes mellitus. Am J Orthop. 2000;29:617-620.
133. Chen S., Anderson M.V., Cheng W.K., et al. Diabetes associated with increased surgical site infections in spinal arthrodesis. Clin Orthop Relat Res. 2009;467:1670-1673.
134. Browne J.A., Cook C., Pietrobon R., et al. Diabetes and early postoperative outcomes following lumbar fusion. Spine. 2007;32:2214-2219.
135. Walid M.S., Newman B.F., Yelverton J.C., et al. Prevalence of previously unknown elevation of glycosylated hemoglobin in spine surgery patients and impact on length of stay and total cost. J Hosp Med. 2010;5(1):E10-E14.
136. Patel N., Bagan B., Vadera S., et al. Obesity and spine surgery: relation to perioperative complications. J Neurosurg Spine. 2007;6:291-297.
137. Shamji M.F., Parker S., Cook C., et al. Impact of body habitus on perioperative morbidity associated with fusion of the thoracolumbar and lumbar spine. Neurosurgery. 2009;65:490-498.
138. Rosen D.S., Ferguson S.D., Ogden A.T., et al. Obesity and self-reported outcome after minimially invasive lumbar spinal fusion surgery. Neurosurgery. 2008;63:956-960.
139. Han I.H., Kim K.S., Park H.C., et al. Spinal surgery in patients with end-stage renal disease undergoing hemodialysis therapy. Spine. 2009;34:1990-1994.
140. Kim D.S., Hilibrand A.S. Rheumatoid arthritis in the cervical spine. J Am Acad Orthop Surg. 2005;13:463-474.
141. Thaller J., Walker M., Kline A.J., et al. The effect of nonsteroidal anti-inflammatory agents on spinal fusion. Orthopedics. 2005;28:299-303.
142. Dumont A.S., Verma S., Dumont R.J., et al. Nonsteroidal anti-inflammatory drugs and bone metabolism in spinal fusion surgery: a pharmacological quandary. J Pharmacol Toxicol Methods. 2000;43:31-39.
143. Glassman S.D., Rose S.M., Dimar J.R., et al. The effect of postoperative nonsteroidal anti-inflammatory drug administration on spinal fusion. Spine. 1998;23:834-838.
144. Dimar J.R., Ante W.A., Zhang Y.P., et al. The effects of nonsteroidal anti-inflammatory drugs on posterior spinal fusions in the rat. Spine. 1996;21:1870-1876.
145. Crawford C.H., Carreon L.Y., Djurasovic M., et al. Lumbar fusion outcomes in patients with rheumatoid arthritis. Eur Spine J. 2008;17:822-825.
146. Koike R., Takeuchi T., Eguchi K., et al. Update on the Japanese guidelines for the use of infliximab and etanercept in rheumatoid arthritis. Mod Rheumatol. 2007;17:451-458.
147. Mori S., Tomita Y., Horikawa T., et al. Delayed spinal infection after laminectomy in a patient with rheumatoid arthritis interruptedly exposed to anti–tumor necrosis factor alpha agents. Clin Rheumatol. 2008;27:937-939.
148. Szpalski M., Gunzburg R. Lumbar spinal stenosis in the elderly: an overview. Eur Spine J. 2003;12:S170-S175.
149. Kim H.J., Lee H.W., Kim H.S., et al. Life expectancy after lumbar spine surgery: one- to eleven-year follow-up of 1015 patients. Spine. 2008;33:2116-2121.
150. Chin D.K., Park J.Y., Yoon Y.S., et al. Prevalence of osteoporosis in patients requiring spine surgery: incidence and significance of osteoporosis in spine disease. Osteoporos Int. 2007;18:1219-1224.
151. Carreon L.Y., Glassman S.D., Djurasovic M., et al. RhBMP-2 versus iliac crest bone graft for lumbar spine fusion in patients over 60 years of age. Spine. 2009;34:238-243.
152. Becker S., Chavanne A., Spitaler R., et al. Assessment of different screw augmentation techniques and screw designs in osteoporotic spines. Eur Spine J. 2008;17:1462-1469.
153. Moon B.J., Cho B.Y., Choi E.Y., et al. Polymethylmethacrylate-augmented screw fixation for stabilization of the osteoporotic spine: a three-year follow-up of 37 patients. J Korean Neurosurg Soc. 2009;46:305-311.
154. Raffo C.S., Lauerman W.C. Predicting morbidity and mortality of lumbar spine arthrodesis in patients in their ninth decade. Spine. 2006;31:99-103.
155. Cassinelli E.H., Eubanks J., Vogt M., et al. Risk factors for the development of perioperative complications in elderly patients undergoing lumbar decompression and arthrodesis for spinal stenosis: an analysis of 166 patients. Spine. 2007;32:230-235.
156. Kilincer C., Steinmetz M.P., Sohn M.J., et al. Effects of age on the perioperative characteristics and short-term outcome of posterior lumbar fusion surgery. J Neurosurg Spine. 2005;3:34-39.
157. Glassman S.D., Carreon L.Y., Dimar J.R., et al. Clinical outcomes in older patients after posterolateral lumbar fusion. Spine J. 2007;7:547-551.
158. Epstein N.E. Fusion rates and SF-36 outcomes after multilevel laminectomy and noninstrumented lumbar fusions in a predominately geriatric population. J Spinal Disord Tech. 2008;21:159-164.
159. Andersen T., Christensen F.B., Ernst C., et al. The effect of electrical stimulation on lumbar spinal fusion in older patients: a randomized, controlled, multi-center trial: part 1: functional Outcome. Spine. 2009;34:2241-2247.
160. Andersen T., Christensen F.B., Egund N., et al. The effect of electrical stimulation on lumbar spinal fusion in older patients: a randomized, controlled, multi-center trial: part 2: fusion rates. Spine. 2009;34:2248-2253.
161. Kanis J.A., Burlet N., Cooper C., et al. European guidance for the diagnosis and management of osteoporosis in postmenopausal women. Osteoporos Int. 2008;19:399-428.
162. Xue Q., Li H., Zou X., et al. The influence of alendronate treatment and bone graft volume on posterior lateral spine fusion in a porcine model. Spine. 2005;30:1116-1121.
163. Tokuhashi Y., Ajiro Y., Umezawa N. Outcomes of posterior fusion using pedicle screw fixation in patients ≥ 70 years with lumbar spinal canal stenosis. Orthopedics. 2008;31:1096.
164. Cavagna R., Tournier C., Aunoble S., et al. Lumbar decompression and fusion in elderly osteoporotic patients: a prospective study using less rigid titanium rod fixation. J Spinal Disord Tech. 2008;21:86-91.
165. Fransen P. Increasing pedicle screw anchoring in the osteoporotic spine by cement injection through the implant: technical note and report of three cases. J Neurosurg Spine. 2007;7:366-369.
166. Aydogan M., Ozturk C., Karatoprak O., et al. The pedicle screw fixation with vertebroplasty augmentation in the surgical treatment of the severe osteoporotic spine. J Spinal Disord Tech. 2009;22:444-447.
167. Tan J.S., Sing S., Zhu Q.A., et al. The effect of cement augmentation and extension of posterior instrumentation on stabilization and adjacent level effects in the elderly spine. Spine. 2008;33:2728-2740.
168. Burval D.J., McLain R.F., Milks R., et al. Primary pedicle screw augmentation in osteoporotic lumbar vertebrae: BIOMECHANICAL analysis of pedicle fixation strength. Spine. 2007;32:1077-1083.
169. Horowitz N.H., Curtin J.A. Prophylactic antibiotics and wound infections following laminectomy for lumber disc herniation. J Neurosurg. 1975;43:727-731.
170. Watters W.C., Baisden J., Bono C.M. Antibiotic prophylaxis in spine surgery: an evidence-based clinical guideline for the use of prophylactic antibiotics in spine surgery. Spine J. 2009;9:142-146.
171. Pons V.G., Denlinger S.L., Guglielmo B.J., et al. Ceftizxime versus vancomycin and gentamicin in neurosurgical prophylaxis: a randomized, prospective, blinded clinical study. Neurosurgery. 1993;33:416-423.
172. Holloway K.L., Smith K.W., Wilberger J.E., et al. Antibiotic prophylaxis during clean neurosurgery: a large, multicenter study using cefuroxime. Clin Ther. 1996;18:84-94.
173. Petignat C., Francioli P., Harbarth S., et al. Cefuroxime prophylaxis is effective in noninstrumented spine surgery. Spine. 2008;33:1919-1924.
174. Dimick J.B., Lipsett P.A., Kostuik J.P. Spine update: antimicrobial prophylaxis in spine surgery: basic principles and recent advances. Spine. 2000;25:2544-2548.
175. Hellbusch L.C., Helzer-Julin M., Doran S.E., et al. Single-dose vs multiple-dose antibiotic prophylaxis in instrumented lumbar fusion—a prospective study. Surg Neurol. 2008;70:622-627.
176. Kanayama M., Hashimoto T., Shigenobu K., et al. Effective prevention of surgical site infection using a Centers for Disease Control and Prevention guideline-based antimicrobial prophylaxis in lumbar spine surgery. J Neurosurg Spine. 2007;6:327-329.
177. Takahashi H., Wada A., Iida Y., et al. Antimicrobial prophylaxis for spinal surgery. J Orthop Sci. 2009;14:40-44.
178. Mastronardi L., Tatta C. Intraoperative antibiotic prophylaxis in clean spinal surgery: a retrospective analysis in a consecutive series of 973 cases. Surg Neurol. 2004;61:129-135.
179. Barker F.G. Efficacy of prophylactic antibiotic therapy in spinal surgery: a meta-analysis. Neurosurgery. 2002;51:391-401.
180. Walters R., Moore R., Fraser R. Penetration of cephazolin in human lumbar intervertebral disc. Spine. 2006;31:567-570.
181. Klekamp J., Spengler D.M., McNamara M.J., et al. Risk factors associated with methicilin-resistant staphylococcal wound infection after spinal surgery. J Spin Disord. 1999;12:187-191.
182. Akins P.T., Belko J., Banerjee A., et al. Perioperative management of neurosurgical patients with methicillin-resistant Staphylococcus aureus. J Neurosurg. 2010;112(2):354-361.
183. Prokuski L. Prophylactic antibiotics in orthopaedic surgery. J Am Acad Orthop Surg. 2008;16:283-293.
184. Milstone A.M., Maragakis L.L., Townsend T., et al. Timing of preoperative antibiotic prophylaxis: a modifiable risk factor for deep surgical site infections after pediatric spinal fusion. Pediatr Infect Dis J. 2008;27:704-708.
185. Swoboda S.M., Merz C., Kostuik J., et al. Does intraoperative blood loss affect antibiotic serum and tissue concentration. Arch Surg. 1996;131:1165-1172.
186. Polly D.W., Meter J.J., Brueckner R., et al. The effect of intraoperative blood loss on serum cefazolin level in patients undergoing instrumented spinal fusion: a prospective, controlled study. Spine. 1996;19:2363-2367.
187. Maragakis L.L., Cosgrove S.E., Martinez E.A., et al. Intraoperative fraction of inspired oxygen is a modifiable risk factor for surgical site infection after spinal surgery. Anesthesiology. 2009;110:556-562.
188. Gruenberg M.F., Campaner G.L., Sola C.A., et al. Ultraclean air for prevention of postoperative infection after posterior spinal fusion with instrumentation: a comparison between surgeries performed with and without a vertical exponential filtered air-flow system. Spine. 2004;29:2330-2334.
189. Payne D.H., Fischgrund J.S., Herkowitz H.N., et al. Efficacy of closed wound suction drainage after single-level lumbar laminectomy. J Spin Disord. 1996;9:401-403.
190. Brown M.D., Brookfield K.F.W. A randomized study of closed wound suction drainage for extensive lumbar spine surgery. Spine. 2004;29:1066-1068.
191. Scuderi G.T., Brusovanik G.V., Fitzhenry L.N., et al. Is wound drainage necessary after lumbar spine fusion surgery? Med Sci Monit. 2005;11:CR64-CR66.
192. Devlin V.J., Schwartz D.M. Intraoperative neurophysiologic monitoring during spinal surgery. J Am Acad Orthop Surg. 2007;15:549-560.
193. Resnick D.K., Choudhri T.F., Dailey A.T., et al. Guidelines for the performance of fusion procedures for degenerative disease of the lumbar spine. Part 15: electrophysiological monitoring and lumbar fusion. J Neurosurg Spine. 2005;2:725-732.
194. Krassioukov A.V., Sarjeant R., Arkia H., et al. Multimodality intraoperative monitoring during complex lumbosacral procedures: indications, techniques, and long-term follow-up review of 61 consecutive cases. J Neurosurg Spine. 2004;3:243-253.
195. Bose B., Wierzbowski L.R., Sestokas A.K. Neurophysiologic monitoring of spinal nerve root function during instrumented posterior lumbar spine surgery. Spine. 2002;27:1444-1450.
196. Raynor B.L., Lenke L.G., Bridwell K.H., et al. Correlations between low triggered electromyographic thresholds and lumbar pedicle screw malposition. Spine. 2007;32:2673-2678.
197. Alemo S., Sayadipour A. Role of intraoperative neuropsyiologic monitoring in lumbosacral spine fusion and instrumentation: a retrospective study. Surg Neurol. 2010;73(1):72-76.
198. Urist M.R. Bone: formation by autoinduction. Science. 1965;150:893-899.
199. Resnick D.K., Choudhri T.F., Dailey A.T., et al. Guidelines for the performance of fusion procedures for degenerative disease of the lumbar spine. Part 16: bone graft extenders and substitutes. J Neurosurg Spine. 2005;2:733-736.
200. Boden S.D., Kang J., Sandhu H., et al. Use of recombinant human bone morphogenetic protein-2 to achieve posterolateral lumbar spine fusion in humans: a prospective, randomized clinical pilot trial. Spine. 2002;27:2662-2673.
201. Boden S.D., Zdeblick T.A., Sandhu H.S., et al. The use of rhBMP-2 in interbody fusion cages: definitive evidence of osteoinduction in humans: a preliminary report. Spine. 2000;25:376-381.
202. Glassman S.D., Dimar J.R., Carreon L.Y., et al. Initial fusion rates with recombinant human bone morphogenetic protein-2/compression resistant matrix and a hydroxyapatite and tricalcium phosphate/collagen carrier in posterolateral spinal fusion. Spine. 2005;30:1694-1698.
203. Dimar J.R., Glassman S.D., Burkus K.J., et al. Clinical outcomes and fusion success at 2 years of single-level instrumented posterolateral fusions with recombinant human bone morphogenetic protein-2/compression resistant matrix versus iliac crest bone graft. Spine. 2006;31:2534-2539.
204. Singh K., Smucker J.D., Boden S.D. Use of recombinant human bone morphogenetic protein-2 as an adjunct in posterolateral lumbar spine fusion: a prospective CT-scan analysis at one and two years. J Spinal Disord Tech. 2006;19:416-423.
205. Hamilton D.K., Jones-Quaidoo S.M., Sansur C., et al. Outcomes of bone morphogenetic protein-2 in mature adults: posterolateral non-instrument assisted lumbar decompression and fusion. Surg Neurol. 2008;69:457-462.
206. Acosta F.L., Cloyd J.M., Aryan H.E., et al. Patient satisfaction and radiographic outcomes after lumbar spinal fusion without iliac crest bone graft or transverse process fusion. J Clin Neurosci. 2009;16:1184-1187.
207. Glassman S.D., Carreon L., Djurasovic M., et al. Posterolateral lumbar spine fusion with INFUSE bone graft. Spine J. 2007;7:44-49.
208. Vaidya R., Weir R., Sethi A., et al. Interbody fusion with allograft and rhBMP-2 leads to consistent fusion but early subsidence. J Bone Joint Surg Br. 2007;89:342-345.
209. Mummaneni P.V., Pan J., Haid R.W., et al. Contribution of recombinant human bone morphogenetic protein-2 to the rapid creation of interbody fusion when used in transforaminal lumbar interbody fusion: a preliminary report. J Neurosurg Spine. 2004;1:19-23.
210. Geibel P.T., Boyd D.L., Slabisak V. The use of recombinant human bone morphogenic protein in posterior interbody fusions of the lumbar spine: a clinical series. J Spinal Disord Tech. 2009;22:315-320.
211. Papakostidis C., Kontakis G., Bhandari M., et al. Efficacy of autologous iliac crest bone graft and bone morphogenetic protein for posterolateral fusion of lumbar spine: a meta-analysis of the results. Spine. 2008;33:E680-E692.
212. Cahill K.S., Chi J.H., Day A., et al. Prevalence, complications and hospital charges associated with use of bone-morphogenetic proteins in spinal fusion procedures. JAMA. 2009;302:58-66.
213. Polly D.W., Ackerman S.J., Shaffrey C.L., et al. A cost analysis of bone morphogenetic protein versus autogenous iliac crest bone graft in single-level anterior lumbar fusion. Orthopedics. 2003;26:1027-1037.
214. Ackerman S.J., Mafilios M.S., Polly D.W.Jr. Economic evaluation of bone morphogenetic protein versus autogenous iliac crest bone in single-level anterior lumbar fusion. Spine. 2002;27:S94-S99.
215. Alt V., Chhabra A., Franke J., et al. An economic analysis of using rhBMP-2 for lumbar fusion in Germany, France and UK from a societal perspective. Eur Spine J. 2009;18:800-806.
216. Dwyer A.F., Yau A.C., Jeffcoat K.W. Use of direct current in spine fusion. J Bone Joint Surg Am. 1974;30:302-312.
217. Akai M., Kawashima N., Kimura T., et al. Electrical stimulation as an adjunct to spinal fusion: a meta-analysis of controlled clinical trials. Bioelectromagnetics. 2002;23:496-504.
218. Gan J.C., Glazer P.A. Electrical stimulation therapies for spinal fusions: current concepts. Eur Spine J. 2006;15:1301-1311.
219. Hodges S.D., Eck J.C., Humphreys S.C. Use of electrical bone stimulation in spinal fusion. J Am Acad Orthop Surg. 2003;11:81-88.
220. Morone M.A., Feuer H. The use of electrical stimulation to enhance spinal fusion. Neurosurg Focus. 2002;13:A5.
221. Oishi M., Onesti S.T. Electrical bone graft stimulation for spinal fusion: a review. Neurosurgery. 2000;47:1041-1056.
222. Fredericks D.C., Smucker J., Petersen E.B., et al. Effects of direct current electrical stimulation on gene expression of osteopromotive factors in a posterolateral spinal fusion model. Spine. 2007;32:174-181.
223. France J.C., Norman T.L., Santrock R.D., et al. The efficacy of direct current stimulation for lumbar intertransverse process fusions in an animal model. Spine. 2001;26:1002-1008.
224. Toth J.M., Seim H.B., Schwardt J.D., et al. Direct current electrical stimulation increases the fusion rate of spinal fusion cages. Spine. 2000;25:2580-2587.
225. Cook S.D., Patron L.P., Christakis P.M., et al. Direct current stimulation of titanium interbody fusion devices in primates. Spine J. 2004;4:300-311.
226. Rogozinski A., Rogozinski C. Efficacy of implanted bone graft stimulation in instrumented lumbosacral spinal fusion. Spine. 1996;21:2479-2483.
227. Kucharzyk D.W. A controlled prospective outcome study of implantable electrical stimulation with spinal instrumentation in a high-risk spinal fusion population. Spine. 1999;24:465-468.
228. Jenis L.G., Ah H.S., Stein R., et al. Prospective comparison of the effect of direct current electrical stimulation and pulsed electromagnetic fields on instrumented posterolateral lumbar arthrodesis. J Spinal Disord. 2000;13:290-296.
229. Resnick D.K., Choudhri T.F., Dailey A.T., et al. Guidelines for the performance of fusion procedures for degenerative disease of the lumbar spine. Part 17: bone growth stimulators and lumbar fusion. J Neurosurg Spine. 2005;2:737-740.
230. Kahanovitz N., Pashos C. The role of implantable direct current electrical stimulation in the critical pathway for lumbar spinal fusion. J Care Manage. 1996;6:2-8.
231. Mooney V.A. randomized double-blind prospective study of the efficacy of pulsed electromagnetic fields for interbody lumbar fusions. Spine. 1990;15:708-712.
232. Bose B. Outcomes after posterolateral lumbar fusion with instrumentation in patients treated with adjunctive pulsed electromagnetic field stimulation. Adv Ther. 2001;18:12-20.
233. Marks R.A. Spine fusion for discogenic low back pain: outcomes in patients treated with or without pulsed electromagnetic field stimulation. Adv Ther. 2000;17:57-67.
234. Simmons J.W., Mooney V I.T. Pseudoarthrosis after lumbar spine fusion: non-operative salvage with pulsed electromagnetic fields. Am J Orthop. 2004;33:27-30.
235. Goodwin C.B., Brighton C.T., Guyer R.D., et al. A double-blind study of capacitively coupled electrical stimulation as an adjunct to lumbar spinal fusions. Spine. 1999;24:1349.
236. Juratli S.M., Franklin G.M., Mirza S.K., et al. Lumbar fusion outcomes in Washington State workers’ compensation. Spine. 2006;31:2715-2723.
237. Cook C., Santos G.C., Lima R., et al. Geographic variation in lumbar fusion for degenerative disorders: 1990 to 2000. Spine J. 2007;7:552-557.
238. Buchowski J.M., Bridwell K.H., Lenke L.G., et al. Spinal cord compression with neurologic deficit associated with intrapedicular application of hemostatic gelatin matrix during pedicle screw insertion. Spine. 2009;34:E473-E477.
239. Khong P., DAy M.J. Spontaneous cerebellar haemorrhage following lumbar fusion. J Clin Neurosci. 2009;16:1673-1675.
240. Raco A., Russo N., Landi A., et al. Lymphatic fluid fistula: an extremely rare complication of posterior lumbar transpedicular screw fixation. J Neurosurg Spine. 2006;4:421-423.
241. Olsen M.A., Mayfield J., Lauryssen C., et al. Risk factors for surgical site infection in spinal surgery. J Neurosurg Spine. 2003;2:149-155.
242. Lietard C., Thebaud V., Besson G., et al. Risk factors for neurosurgical site infections: an 18-month prospective survey. J Neurosurg. 2008;109:729-734.
243. Gunne A.F., Cohen D.B. Incidence, prevalence, and analysis of risk factors for surgical site infection following adult spinal surgery. Spine. 2009;34:1422-1428.
244. Weinstein M.A., McCabe J.P., Cammisa F.P.Jr. Postoperative spinal wound infection: a review of 2,391 consecutive index procedures. J Spinal Disord. 2000;13:422-426.
245. Fang A., Hu S.S., Endres N., et al. Risk factors for infection after spinal surgery. Spine. 2005;30:1460-1465.
246. Kanafani Z.A., Dakdouki G.K., El-Dbouni O. Surgical site infections following spinal surgery at a tertiary care center in Lebanon: incidence, microbiology, and risk factors. Scan J Infect Dis. 2006;38:589-592.
247. Levi A.D., Dickman C.A., Sonntag V.K. Management of postoperative infections after spinal instrumentation. J Neurosurg. 1997;86:975-980.
248. Perry J.W., Montgomerie J.Z., Swank S., et al. Wound infections following spinal fusion with posterior segmental spinal instrumentation. Clin Infect Dis. 1997;24:558-561.
249. Boston K.M., Baraniuk S., O’Heron S., et al. Risk factors for spinal surgical site infection, Houston. Texas. Infect Control Hosp Epidemiol. 2009;30:884-889.
250. Dick J., Boachie-Adjei O., Wilson M. One-stage versus two-stage anterior and posterior spinal reconstruction in adults. Comparison of outcomes including nutritional status, complication rates, hospital costs, and other factors. Spine. 1992;17:S310-S316.
251. Hultman C.S., Jones G.E., Losken A., et al. Salvage of infected spinal hardware with paraspinous muscle flaps: anatomic considerations with clinical correlation. Ann Plast Surg. 2006;57:521-528.
252. Vender J.R., Hester S., Houle P.J., et al. The use of closed-suction irrigation systems to manage spinal infections. J Neurosurg Spine. 2005;3:276-282.
253. Mehbod A.A., Ogilvie J.W., Pinto M.R., et al. Postoperative deep wound infections in adults after spinal fusion: management with vacuum-assisted wound closure. J Spinal Disord Tech. 2005;18:14-17.
254. Yoshimoto H., Sato S., Nakagawa I., et al. Deep vein thrombosis due to migrated graft bone after posterior lumbosacral interbody fusion. J Neurosurg Spine. 2007;6:47-51.
255. Gill B., Heavner J.E. Postoperative visual loss associated with spine surgery. Eur Spine J. 2006;15:479-484.
256. Heitz J.W., Audu P.B. Asymmetric postoperative visual loss after spine surgery in the lateral decubitus position. Br J Anaesth. 2008;101:380-382.
257. Lee L.A., Roth S., Posner K.L., et al. The American Society of Anesthesiologists Postoperative Visual Loss Registry: analysis of 93 spine surgery cases with postoperative visual loss. Anesthesiology. 2006;105:652-659.
258. American Society of Anesthesiologists Task Force on Perioperative Blindness. Practice advisory for perioperative visual loss associated with spine surgery: a report by the American Society of Anesthesiologists Task Force on Perioperative Blindness. Anesthesiology. 2006;104:1319-1328.
259. Kamming D., Clarke S. Postoperative visual loss following prone spinal surgery. Br J Anaesth. 2005;95:257-260.
260. Murphy M.A. Bilateral posterior ischemic optic neuropathy after lumbar spine surgery. Ophthalmology. 2003;110:1454-1457.
261. Stevens W.R., Glazer P.A., Kelley S.D. Ophthalmic complications after spinal surgery. Spine. 1997;22:1325-1329.
262. Myers M.A., Hamilton S.R., Bogosian A.J., et al. Visual loss as a complication of spine surgery: a review of 37 cases. Spine. 1997;22:1319-1324.
263. Patil C.G., Lad E.M., Lad S.P., et al. Visual loss after spine surgery: a population-based study. Spine. 2008;33:1491-1496.
264. Foster M.R. Rhabdomyolysis in lumbar spine surgery: a case report. Spine. 2003;28:E276-E278.
265. Papadakis M., Sapkas G., Tzoutzopoulos A. A rare case of rhabdomyolysis and acute renal failure following spinal surgery. J Neurosurg Spine. 2008;9:387-389.
266. Prabhu M., Samra S. An unusual case of rhabdomyolysis following surgery in the prone position. J Neurosurg Anesthesiol. 2000;12:359-363.
267. Banit D.M., Wheeler A.H., Darden B.V. Recurrent transverse myelitis after lumbar spine surgery: a case report. Spine. 2003;28:E165-E168.
268. Vavken P., Krepler P. Sacral fractures after multi-segmental lumbosacral fusion: a series of four cases and systematic review of literature. Eur Spine J. 2008;17:S285-S290.
269. Fourney D.R., Prabhu S.S., Cohen Z.R., et al. Early sacral stress fracture after reduction of spondylolisthesis and lumbosacral fixation: case report. Neurosurgery. 2002;51:1507-1511.
270. Klineberg E., McHenry T., Bellabarba C., et al. Sacral insufficiency fractures caudal to instrumented posterior lumbosacral arthrodesis. Spine. 2008;33:1806-1811.
271. Papadopoulos E.C., Cammisa F.P.Jr., Girardi F.P. Sacral fractures complicating thoracolumbar fusion to the sacrum. Spine. 2008;33:E699-E707.
272. Khan M.H., Smith P.N., Kang J.D. Sacral insufficiency fractures following multilevel instrumented spinal fusion: case report. Spine. 2005;30:E484-E488.
273. Koh Y.D., Kim J.O., Lee J.J. Stress fracture of the pelvic wing-sacrum after long-level lumbosacral fusion: a case report. Spine. 2005;30:E161-E163.
274. Matthews V., McCance S.E., O’Leary P.F. Early fracture of the sacrum or pelvis. An unusual complication after multilevel instrumented lumbosacral fusion. Spine. 2001;26:E571-E575.
275. Whitecloud T.S., Davis J.M., Olive P.M. Operative treatment of the degenerated segment adjacent to a lumbar fusion. Spine. 1994;19:531-536.
276. Kumar M.N., Jacquot F., Hall H. Long-term follow-up of functional outcomes and radiographic changes at adjacent levels following lumbar spine fusion for degenerative disc disease. Eur Spine J. 2001;10:309-313.
277. Min J.H., Jang J.S., Jung B.J., et al. The clinical characteristics and risk factors for the adjacent segment degeneration in instrumented lumbar fusion. J Spinal Disord Tech. 2008;21:305-309.
278. Gertzbein S.D., Hollopeter M.R. Disc herniation after lumbar fusion. Spine. 2002;27:E373-E376.
279. Ghiselli G., Wang J.C., Bhatia N.N., et al. Adjacent segment degeneration in the lumbar spine. J Bone Joint Surg Am. 2004;86:1497-1503.
280. Aiki H., Ohwada O., Kobayashi H., et al. Adjacent segment stenosis after lumbar fusion requiring second operation. J Orthop Sci. 2005;10:490-495.
281. Disch A.C., Schmoelz W., Matziolis G., et al. Higher risk of adjacent segment degeneration after floating fusions: long-term outcome after low lumbar spine fusions. J Spinal Disord Tech. 2008;21:79-85.
282. Cheh G., Bridwell K.H., Lenke L.G., et al. Adjacent segment disease following lumbar/thoracolumbar fusion with pedicle screw instrumentation: a minimum 5-year follow-up. Spine. 2007;32:2253-2257.
283. Yang J.Y., Lee J.K., Song H.S. The impact of adjacent segment degeneration clinical outcome after lumbar spinal fusion. Spine. 2008;34:503-507.
284. Ekman P., Moller H., Shalabi A., et al. A prospective randomized study on the long-term effect of lumbar fusion on adjacent disc degeneration. Eur Spine J. 2009;18:1175-1186.
285. Lai P.L., Chen L.H., Niu C.C., et al. Relation between laminectomy and development of adjacent segment instability after lumbar fusion with pedicle fixation. Spine. 2004;29:2527-2532.
286. Cakir B., Carazzo C., Schmidt R., et al. Adjacent segment mobility after rigid and semirigid instrumentation of the lumbar spine. Spine. 2009;34:1287-1291.
287. Maigne J.Y., Planchon C.A. Sacroiliac joint pain after lumbar fusion. A study with anesthetic blocks. Eur Spine J. 2005;14:654-658.
288. Bednar D.A., Al-Tunaib W. Failure of reconstitution of open-section, posterior iliac-wing bone graft donor sites after lumbar spinal fusion. Observations with implications for the etiology of donor site pain. Eur Spine J. 2005;14:95-98.
289. Singh K., Phillips F.M., Kuo E., et al. A prospective, randomized, double-blind study of the efficacy of postoperative continuous local anesthetic infusion at the iliac crest bone graft site after posterior spinal arthrodesis: a minimum of 4-year follow-up. Spine. 2007;32:2790-2796.
290. Schwartz C.E., Martha J.F., Kowalski P., et al. Prospective evaluation of chronic pain associated with posterior autologous iliac crest bone graft harvest and its effect on postoperative outcome. Health Qual Life Outcomes. 2009;7:49.
291. Delawi D., Dhert W.J., Castelein R.M., et al. The incidence of donor site pain after bone graft harvesting from the posterior iliac crest may be overestimated: a study on spine fracture patients. Spine. 2007;32:1865-1868.
292. Kim D.H., Rhim R., Li L., et al. Prospective study of iliac crest bone graft harvest site pain and morbidity. Spine J. 2009;9:886-892.
293. Ivanov A.A., Kiapour A., Ebraheim N.A., et al. Lumbar fusion leads to increases in angular motion and stress across sacroiliac joint: a finite element study. Spine. 2009;34:E162-E169.
294. Berg S., Fritzell P., Tropp H. Sex life and sexual function in men and women before and after total disc replacement compared with posterior lumbar fusion. Spine J. 2009;9:987-994.
295. Naraghi F., Wolfer L., Lewis M., et al. BMP-2 dose correlates with increased postoperative edema and swallowing complications after cervical fusion. Spine. 2006;6:S7-S8.
296. Patel V.V., Zhao L., Wong P. Controlling bone morphogenetic protein diffusion and bone morphogenetic–stimulated bone growth using fibrin glue. Spine. 2006;31:1201-1206.
297. Patel V.V., Zhao L., Wong P., et al. An in vitro and in vivo analysis of fibrin glue use to control bone morphogenetic protein diffusion and bone morphogenetic protein–stimulated bone growth. Spine J. 2006;6:397-404.
298. Brower R.S., Vickroy N.M. A case of psoas ossification from the use of BMP-2 for posterolateral fusion at L4-L5. Spine. 2008;33:E653-E655.
299. Joseph V., Rampersaud Y.R. Heterotopic bone formation with the use of rhBMP2 in posterior minimal access interbody fusion: a CT analysis. Spine. 2007;32:2885-2890.
300. Hwang C.J., Vaccaro A.R., Lawrence L.P., et al. Neurologic impairment from ectopic bone in the lumbar canal: a potential complication of off-label PLIF/TLIF use of bone morphogenetic protein-2 (BMP-2). Spine J. 2008;8:1011-1018.
301. Lewandrowski K.U., Nanson C., Calderon R. Vertebral osteolysis after posterior interbody lumbar fusion with recombinant human bone morphogenetic protein 2: a report of five cases. Spine J. 2007;7:609-614.
302. Hwang C.J., Vaccaro A.R., Lawrence J.P., et al. Immunogenicity of bone morphogenetic proteins. J Neurosurg Spine. 2009;10:443-451.
303. Connoly P.J., Grob D. Bracing of patients after fusion for degenerative problems of the lubar spine—yes or no? Spine. 1998;23:1426-1428.
304. Johnsson R., Stromqvist B., Axelsson P., et al. Influence of spinal immubilization on consolidation of posterolateral lumbosacral fusion. A roentgen stereophotogrammetric and radiographic analysis. Spine. 1992;17:16-21.
305. Yee A.J., Yoo J.U., Marsolais E.B., et al. Use of postoperative lumbar corset after lumbar spinal arthrodesis for degenerative conditions of the spine: a prospective randomized trial. J Bone Joint Surg Am. 2008;90:2062-2068.
306. Bible J.E., Biswas D., Whang P.G., et al. Postoperative bracing after spine surgery for degenerative conditions: a questionnaire study. Spine J. 2009;9:309-316.
307. Resnick D.K., Choudhri T.F., Dailey A.T., et al. Guidelines for the performance of fusion procedures for degenerative disease of the lumbar spine. Part 4: radiographic assessment of fusion. J Neurosurg Spine. 2005;2:653-657.
308. Brodsky A.E., Kovalsky E.S., Khalil M.A. Correlation of radiologic assessment of lumbar spine fusions with surgical exploration. Spine. 1991;16:S261-S265.
309. Kant A.P., Daum W.J., Dean S.M., et al. Evaluation of lumbar spine fusion: plain radiographs versus direct surgical exploration and observation. Spine. 1995;20(21):2313-2317.
310. Romero N.C., Glaser J., Walton Z. Are routine radiographs needed in the first year after lumbar spinal fusions? Spine. 2009;34:1578-1580.
311. Bono C.M., Khandha A., Vadapalli S., et al. Residual sagittal motion after lumbar fusion: a finite element analysis with implications on radiographic flexion-extension criteria. Spine. 2007;32:417-422.
312. Carreon L.Y., Dijurasovic M., Glassman S.D., et al. Diagnostic accuracy and reliability of fine-cut CT scans with reconstructions to determine the status of an instrumented posterolateral fusion with surgical exploration as reference standard. Spine. 2007;32:892-895.
313. Andrews C.L. Evaluation of the postoperative spine: spinal instrumentation and fusion. Semin Musculoskelet Radiol. 2000;4:259-279.
314. Tokuhashi Y., Matsuzaki H., Oda H., et al. Clinical course and significance of the clear zone around the pedicle screws in the lumbar degenerative disease. Spine. 2008;33:903-908.
315. Brooks D., Eskander M., Balsis S., et al. Imaging assessment of lumbar pedicle screw placement: sensitivity and specificity of plain radiographs and computer axial tomography. Spine. 2007;32:1450-1453.
316. Choma T.J., Denis F., Lonstein J.E., et al. Stepwise methodology for plain radiographic assessment of pedicle screw placement: a comparison with computed tomography. J Spinal Disord Tech. 2006;19:547-553.
317. Shah R.R., Mohammed S., Saifuddin A., et al. Comparison of plain radiographs with CT scan to evaluate interbody fusion following the use of titanium interbody cages and transpedicular instrumentation. Eur Spine J. 2003;12:378-385.
318. Bohnsack M., Gosse F., Ruhmann O., et al. The value of scintigraphy in the diagnosis of pseudoarthrosis after spinal fusion surgery. J Spinal Disord. 1999;12:482-484.
319. Rihn J.A., Lee J.Y., Khan M., et al. Does lumbar facet fluid detected on magnetic resonance imaging correlate with radiographic instability in patients with degenerative lumbar disease? Spine. 2007;32:1555-1560.
320. Deyo R.A., Cherkin D., Conrad D., et al. Cost, controversy, crisis: low back pain and the health of the public. Ann Rev Publ Health. 1991;12:141-156.
321. Resnick D.K., Choudhri T.F., Dailey A.T., et al. Guidelines for the performance of fusion procedures for degenerative disease of the lumbar spine. Part 3: assessment of economic outcome. J Neurosurg Spine. 2005;2:647-652.
322. Deyo R.A., Gray D.T., Kreuter W., et al. United States trends in lumbar fusion surgery for degenerative conditions. Spine. 2005;30:1441-1445.
323. Gray D.T., Deyo R.A., Kreuter W., et al. Population-based trends in volumes and rates of ambulatory lumbar spine surgery. Spine. 2006;31:1957-1963.
324. Weinstein J.N., Lurie L.D., Olsen P.R., et al. United States’ trends and regional variations in lumbar spine surgery: 1992-2003. Spine. 31, 2006. 2707–2714
325. Deyo R.A., Mirza S.K. Trends and variations in the use of spine surgery. Clin Orthop Relat Res. 2006;443:139-146.
326. Deyo R.A., Ciol M.A., Cherkin D.C., et al. Lumbar spinal fusion: a cohort study of complications, reoperations and resource use in the Medicare population. Spine. 1993;18:1463-1470.
327. Whang P.G., Lim M.R., Sasso R.C., et al. Financial incentives for lumbar surgery: a critical analysis of physician reimbursement for decompression and fusion procedures. J Spinal Disord Tech. 2008;21:381-386.
328. Miyazaki M., Sugiyama O., Tow B., et al. The effects of lentiviral gene therapy with bone morphogenetic protein-2–producing bone marrow cells on spinal fusion in rats. J Spinal Disord Tech. 2008;21:372-379.
329. Yoon S.T., Boden S.D. Spine fusion by gene therapy. Gene Ther. 2004;11:360-367.
330. Lopez M.J., McIntosh K.R., Spencer N.D., et al. Acceleration of spinal fusion using syngeneic and allogeneic adult adipose derived stem cells in a rat model. J Orthop Res. 2009;27:366-373.
331. Sheyn D., Pelled G., Zilberman Y., et al. Nonvirally engineered porcine adipose tissue–derived stem cells: use in posterior spinal fusion. Stem Cells. 2008;26:1056-1064.