Positive-pressure mechanical ventilation
In addition to these industry-derived improvements, our understanding of intraoperative changes in respiratory mechanics has also improved so that appropriate MV settings can be used in patients with airflow obstruction or lung or chest wall abnormalities. Recent concern about the risk of developing acute lung injury in previously healthy patients after intraoperative MV is also discussed in this chapter. A list of the abbreviations for terms commonly used in respiratory physiology is provided in Box 223-1.
Respiratory mechanics
Two independent patient-related forces have to be overcome to achieve lung inflation by positive-pressure MV (Figure 223-1): (1) resistance to the flow of gases within the airway and (2) compliance and elastance of the lung and chest wall, respectively. The latter concept can be difficult for the anesthesia provider to understand. In an inflated lung, the elastin within the lung is stretched. If you then remove a lung from the thoracic cavity and if the airway is open, the lung will collapse. This does not happen within the thoracic cavity because the parietal pleura is adherent to the thoracic cavity. In an intubated paralyzed patient at end expiration, or when the lungs are at their functional residual capacity, positive pressure must be applied to expand the lungs to deliver a breath. The amount of pressure (plateau pressure [Pplat]) applied to expand the lungs to a known volume is a measure of the compliance of the lung (Compliance = ΔV/ΔP). For example, 5 cm of H2O pressure in a patient with a VT of 500 would equal a compliance of 100. In a patient with a diseased lung (e.g., with severe acute respiratory distress syndrome), the compliance would typically be less than 20.
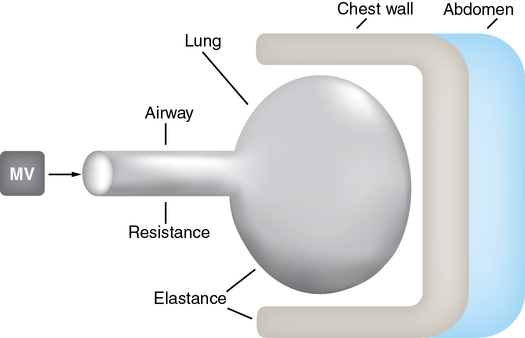
< ?xml:namespace prefix = "mml" />

Mode of mechanical ventilation
Volume control mechanical ventilation
During volume control mode, VT and TI (or respiratory rate and inspiratory: expiratory [I:E] ratio) are set by the clinician and inspiratory flow remains constant (Figure 223-2). In volume control MV, the resistive impedance (transmission of the VT through the airway) and the elastic impedance (compliance of the lung or chest wall) both contribute to the peak airway pressure (Ppeak).
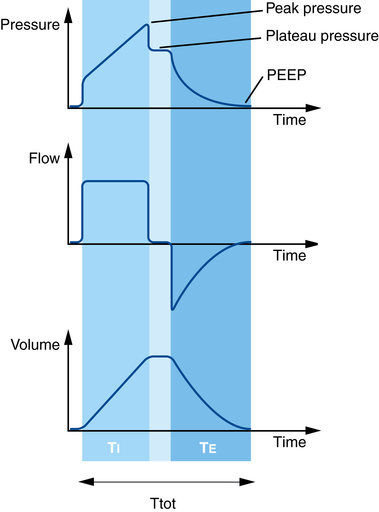
A short end-inspiratory pause may be included in the respiratory cycle in volume control MV so that, after the VT has been fully delivered and just prior to exhalation, airflow is reduced to zero for a fixed proportion of TI. The airway pressure recorded during this end-inspiratory interval is called the plateau (Pplat), pause, or end-inspiratory hold pressure (see Figure 223-2). In many anesthesia ventilators, the default setting in volume control mode may include no end-inspiratory pause so that only Ppeak is recorded. However, it is always possible (and desirable) to include an end-inspiratory pause when using volume control MV so that both Ppeak and Pplat are recorded.
Pressure control mechanical ventilation
In pressure control MV, inspiratory pressure and TI (or respiratory rate and I:E ratio) are set by the clinician and remain constant, but the delivered VT and inspiratory flow will vary during inspiration (Figure 223-3). In pressure control MV, in which inspiratory pressure remains constant for the duration of TI, no Ppeak or end-inspiratory Pplat is registered (as occurs in volume control mode when an end-inspiratory pause is added into the respiratory cycle). Therefore, the relationship between Ppeak and Pplat that is informative in volume control MV cannot be used in pressure control MV.