Chapter 105 Pediatric Neurorehabilitation Medicine
Introduction
Rehabilitation is the process of restoration following injury or disease, with the goal of maximizing an individual’s ability to function in a normal manner. The application of rehabilitation extends far beyond the bounds of traditional medicine since one of its chief aims is to protect or restore personal and social identity [Ward and McIntosh, 2003]. Neurorehabilitation therefore encompasses two distinct, but overlapping, concepts. The first is that neurorehabilitation is a medical subspecialty that treats patients with disabling (and often chronic) diseases of the central and peripheral nervous system [Selzer, 1992]. So defined, neurorehabilitation translates into the active process designed to reduce the effects of a primary neurological condition on performance of activities in daily life. A second aspect stresses the specific therapeutic modalities that are implemented to overcome or improve any neurological impairment that interferes with daily life [Ward and McIntosh, 2003]. Both aspects clearly have practical implications for neurorehabilitation as it is carried out in children [Taylor, 1991].
The principles, goals, and challenges of both pediatric neurorehabilitation and rehabilitation in general are best understood in the context of the International Classification of Functioning, Disability and Health, which replaces the previous scheme entitled the International Classification of Impairments, Disabilities and Handicaps [Thomas-Stonell et al., 2006]. As originally defined by the World Health Organization, impairment is the loss or abnormality of physiologic, psychologic, or anatomic structure or function, while disability, the lack of ability or restricted ability to perform a functional task, is very dependent upon the individual’s environment. Thus, one of the ways in which neurorehabilitation can be carried out is to modify or adapt the environment. Appropriate neurorehabilitation of disability in childhood must be predicated upon a clear understanding of normal development, as well as the ramifications of abnormal development. Finally, it must be recognized that disability does not flow solely or directly from the type and degree of impairment. Instead, disability is best viewed as a three-dimensional construct, consisting of impairment, activity limitations, and participation restrictions [WHO, 1997]. Each dimension is the result of interaction between the biologic features intrinsic to the individual’s medical condition and the child’s physical and social environment [Bickenbach et al., 1999].
The concept of dependency also plays a fundamental role in medical rehabilitation [Ward and McIntosh, 2003]. Dependency is more readily quantifiable than disability, and degree of dependency is a critical element in the cost of on-going medical and rehabilitation care, as well as in eventual quality of life. While a variety of scales have been developed to measure a patient’s potential autonomy, or conversely, the burden imposed on a caregiver, use in pediatric patients is limited because of the inter-related issues of anticipated development and maturation. In our experience and that of others, the WeeFIM (Functional Independence Measure) is a very useful rehabilitation tool [Rice et al., 2005].
The disablement/dependency paradigm in pediatric neurorehabilitation emphasizes movement towards appropriate functional outcomes, as well as diagnosis, and thus is more informative than traditional neurodiagnostic categories [Taylor, 1991]. There also appear to be other distinct advantages to conceptualizing neurorehabilitation in this manner. First, therapeutic interventions and treatment modalities should be based primarily on the disablement and resultant activity limitations, as opposed to the underlying pathologic process producing the impairment. In practical terms, this means that rehabilitation techniques often may be transferred successfully from one child to another despite differences in their primary neurological diagnosis [Ward and McIntosh, 2003]. Similarly, the process of neurorehabilitation logically involves a multidisciplinary team, comprised of physicians, nurses, therapists, and other healthcare professionals, who work in collaboration with the patient and the patient’s family. Finally, the paradigm provides a framework for patients that can be expanded to encompass all of the multifaceted aspects of neurorehabilitation inherent in the practice of child neurology. For example, the paradigm works just as well in describing an adolescent with seizures (impairment) who is prohibited from driving an automobile (disablement) and thus relies on his parents for rides (dependency), as it does for a child with a lower thoracic spinal cord injury causing paralysis of the legs and thus a limited capacity to ambulate. In both cases, there are resultant activity limitations that will affect social life and future employment opportunities.
Mechanisms Underlying Functional Recovery in the Nervous System
Implicit in any consideration of the rehabilitation process is the recognition that the potential for recovery of function exists. The degree of recovery following injury to the nervous system is variable but rarely complete. None the less, a better understanding of the cellular and molecular bases of neuronal dysfunction, as well as the potential mechanisms by which recovery of function occurs, is the framework upon which development of any pediatric neurorehabilitation program must rest [Noetzel, 2003]. Since each proposed mechanism of recovery follows a different time course, the implications are very important in defining an appropriate therapy program.
Mechanisms regarding potential recovery of neurological function are, at best, poorly understood. However, several general conclusions, based upon an increasing amount of scientific evidence, can be drawn. First, significant capabilities of biologic modification in response to injury and developmental aberrations exist within the nervous system. The potential mechanisms by which the nervous system can respond to injury are exceedingly varied and more dynamic than suggested by prior studies [Taub et al., 2002]. Finally, meaningful recovery of function continues for an extended period of time, one that is longer than previously recognized [Graham, 1999]. These findings stand in contrast to former beliefs that recovery essentially was a biphasic process, in that any improvement that occurred within the first few months following an injury was felt to be due to reversibility of factors affecting dysfunctional, as opposed to dead, tissue. Improvement in functional capabilities at later time periods was attributed to nonphysiologic factors, such as learning and behavior modification. Presently, however, there is strong evidence that changes in neuronal circuitry, as opposed to psychologic factors, mediate recovery of function [Johnston, 2009]. The level of scientific information does not allow us to separate out one set of factors from another. Concerning acquired injury to the central nervous system (CNS), it is appropriate to acknowledge that there is ongoing debate concerning the relative contributions of biological healing and learned adaptations to the process of functional recovery.
Resolution of Temporary Dysfunction
The mechanisms underlying recovery of function that occurs within the hours to days following injury to the nervous system most likely relate to resolution of temporary dysfunction in areas of the brain that have not been damaged irreversibly. Potential factors causing transient functional impairment include mild tissue hypoxia, elevated intracranial pressure, edema (both cytotoxic and vasogenic), small contusions and/or focal hematomas, and reversible depression of metabolic and enzymatic activity in areas of the nervous system remote from the primary injury. Clinical and experimental evidence supports each of the first four factors described above [Waxman, 1988; Duffau, 2006]. Furthermore, focal brain injury can result in depression of metabolic activity in noncontiguous brain areas, as a result of a decrease in transmitter synthesizing enzymes in regions of damage [Shreiber et al., 1999]. In a similar fashion, the concept of “diaschisis” has evolved, as a form of neural shock in which uninvolved areas of the brain are rendered temporarily nonfunctional when deprived of appropriate input from a remote area of injured brain [Duffau, 2006]. Recovery of function presumably transpires as a result of increased neurotransmitter synthesis, or more likely, elevated production of neurotransmitter receptors. Some portion of early recovery (12–24 hours lasting up to a week) in humans following brain injury may relate to resolution of similar metabolic derangements [Hovda et al., 1995].
Reorganization of Neuronal Connections
Another potential mechanism by which recovery may occur following nervous system injury is via modification of active neural connections. Three specific modifications of neural connections – axonal regeneration, axon retraction, and collateral sprouting – have been touted as the most promising examples of reorganizational mechanisms in the nervous system. Axonal regeneration, the process by which damaged axons regrow to their normal target, serves as a dominant process of recovery in the peripheral nervous system. Whether it plays any significant role in the restoration of brain function in humans is debatable, since regeneration in the mammalian CNS rarely has been documented, and then only under highly artificial conditions [Fawcett et al., 2001]. Other mechanisms by which neuronal reorganization transpires include axon retraction and the associated process of pruning of synapses in the developing brain. In the early stages of development, certain axons project branches that ultimately are destined to be retracted at a later time. Similarly, maturation of the cerebral cortex in children in early postnatal life is characterized by an initial proliferation of synapse formation, followed later by activity-dependent pruning of excessive synapses [Huttenlocher and Dabholkar, 1997]. The duration of this dynamic change in synaptic number varies between specific regions of cortex, but in the frontal lobe, synaptic thickening still is evident well into teenage years [Huttenlocher, 1990]. The time course of programmed retraction of axon collaterals, although less well established, likely also is confined to early development [Saxena and Caroni, 2007]. Research now indicates that this normal retraction process may not occur if nervous system injury is sustained early on, thus providing a mechanism for retention of function. For example, positron emission tomographic (PET) and functional magnetic resonance imaging (fMRI) studies have demonstrated that, following hemispherectomy for refractory childhood epilepsy, recovery of motor function in the leg contralateral to the surgery is associated with enhanced activation of the ipsilateral hemisphere, presumably via persistent ipsilateral corticospinal tracts [de Bode et al., 2005]. However, following stroke, neuronal activity in the cortex opposite the side of damage actually correlates with reduced functional recovery [Carmichael, 2006]. Multiple brain activation paradigms in stroke patients have revealed that functional improvement largely is dependent on cortical reorganization strategies within brain connected with or adjacent to the region of injury. The mechanism primarily responsible for this type of recovery is collateral sprouting, also termed reactive synaptogenesis. This process involves the axonal outgrowth from undamaged neurons and the establishment of synaptic contact at sites vacated by degenerating or dying neurons. Collateral sprouting typically is confined to brain regions normally innervated by nerve cells sharing common features with the injured neurons. Additionally, the axonal growth cones respect certain anatomic boundaries and, thus, their movement is not into novel nervous system domains. Collateral sprouting appears to be greatly influenced by growth and apoptotic factors [Carmichael et al., 2005]. Thus, it is not surprising that the magnitude and rate of sprouting following an acute injury to the nervous system appear much greater in the immature brain. Recent work has revealed events at the cellular level that drive this regenerative process through activation of genes responsible for molecular growth-promoting programs [Carmichael, 2006].
A final anatomic mechanism involved in modifying neural connections in response to brain injury consists of a resting population of neural progenitor cells, which retain the capacity to proliferate, divide, and differentiate following injury, especially stroke, when activated by the correct signals [Zhang et al., 2005]. In addition, damage in the CNS also appears to initiate a cascade of molecular signals that recruit migrating neuroblasts into the areas of injury. Whether these cells, through neurogenesis, promote restoration of function in humans, however, has not been established.
Plasticity of the Nervous System
Another explanation for restoration of neurological function following injury is that the nervous system has developed characteristics that allow a certain degree of malleability both during development and in reaction to damage. Plasticity is a term that encompasses multiple capabilities of the nervous system to encode information in response to learning, as well as adapting to environmental changes [Johnston, 2009]. In the context of rehabilitation, plasticity may relate both to redundancy within the nervous system and to the process of vicarious functioning. Redundancy implies that there are latent neural connections and silent synapses, which can subserve a particular function if the primary control pathway is damaged [Jenkins and Merzenich, 1987]. It is a compensatory strategy in which a damaged neural system subsequently comes to rely on new queues or different receptors, with resultant reorganization of the cortex. This type of acute adaptation has been well defined in experimental conditions. In human recovery, its role is gaining acceptance; the best evidence supporting its existence comes from fMRI and PET studies documenting recovery of function in young patients undergoing surgical hemispherectomy [Villablanca and Hovda, 2000; de Bode et al., 2005]. Various neurologic functions, including motor performance and expressive language, can shift from a damaged area of brain to the corresponding location in the opposite hemisphere [Hertz-Pannier et al., 2002].
The process by which neural tissues not normally involved in performance of a particular task alter their characteristics to assume control of the task has been labeled vicarious functioning [Graham, 1999]. Vicarious functioning most commonly occurs in an area of the brain adjacent to the injured site or systematically related to the damaged tissue. Evidence supporting this construct comes from experimental two-stage lesions, as well as PET studies involving the immature visual cortex. For example, in adults who lost sight at an early age, Braille reading not only activated the somatosensory cortex representing the reading fingers, but also the primary visual cortex [Sadato et al., 1996]. The concept of vicarious functioning implies a highly developed capability for CNS reorganization, which typically is not attributed to the mature nervous system. Thus, while the role of vicarious functioning in children is likely, its role for recovery in adults is less certain.
Mechanisms of Late Recovery
Clearly, recovery following damage to the nervous system is a highly complex and a remarkable series of processes. The postulated mechanisms appear to favor the immature or developing brain, thus accounting for the relatively better outcomes typically demonstrated in children. The proposed events of neural reorganization that emerge in response to injury are, for the most part, well-recognized, normal developmental processes of maturation, which are reactivated or potentiated in response to CNS damage [Finger and Almli, 1985]. Hopefully, as our understanding of these mechanisms of recovery increases, there will come a time when neurorehabilitation therapy will be tailored to fit the particular circumstances of nervous system injury and restoration.
Principles of Pediatric Neurorehabilitation
Disorders involving the CNS represent a large proportion of all severe and complex disabilities, especially those secondary to trauma and other acquired injury. This situation is especially true in the pediatric population, where injury to or malformation of the brain and spinal cord accounts for the vast majority of children referred for rehabilitation. Pediatric neurologists therefore are uniquely positioned to contribute in a meaningful way to the discipline of pediatric rehabilitation [Taylor, 1991].
Pediatric rehabilitation has several guiding principles based upon the nature of the discipline, as well as the age and developmental level of the patients requiring treatment (Box 105-1) [Noetzel, 2003]. A fundamental principle of neurorehabilitation is that the process mandates a coordinated transdisciplinary team working in unison to provide integrated evaluations and therapeutic interventions. A variety of “nonmedical” issues, such as home and school accessibility, psychosocial adaptation to disability, and school reintegration, mandates that the team include individuals who can address these all-important considerations. A second essential principle is that the rehabilitation process must concentrate on strategies designed to effect true functional improvement, as opposed to enacting treatments that merely decrease symptoms without resulting in improvement in a patient’s capabilities. In pediatric rehabilitation, this means establishing practical management decisions that are endorsed not only by the patient, but also by the family. In order to accomplish this goal, the team must have a clear understanding of the physical, emotional, cognitive, and social consequences of a child’s injury [Ylivsaker et al., 1999]. The degree, extent, and rate of recovery, which varies significantly among children, as well as differences in functioning from setting to setting and task to task in a single child, mandate continual reassessment. As previously described, the immature brain’s response to injury is both varied and dynamic. This evolving biologic recovery, in combination with substitute/alternative cognitive processes and movement patterns, necessitates an on-going program of assessment and reassessment. Additionally, progress from the baseline condition hopefully will be observed as a direct response to the therapeutic interventions. Rehabilitation, especially as it pertains to nervous system injury, has been described as a spiral management process in which, following an initial evaluation, a treatment program is initiated; this, in turn, is constantly revised and updated, based on successive reassessments, taking into consideration therapy-mediated improvements [Ward and McIntosh, 2003].
Another fundamental and distinguishing principle of pediatric rehabilitation is that these frequent reassessments of cognitive, motor, and psychosocial deficits, especially when resulting from acquired nervous system injury, must be guided by an understanding of normative development. Detailed understanding of the patterns of cognitive development during maturation is especially important in the rehabilitation of infants and children for several reasons. First, normative developmental milestones can be identified as sequential goals within an individual’s therapy program [Ylivsaker et al., 1999]. In addition, as a result of injury or illness, the pediatric patient typically loses age-appropriate developmental capabilities. As such, the manner in which therapy can be provided to the patient and the individual’s ability to respond to it will be limited. This is most notable in children and adolescents early in the recovery process, who lack the developmental skills necessary to learn complex strategies upon which functional substitution is predicated [Noetzel, 2003]. Finally, an understanding of cognitive development allows more accurate prediction of the long-term effects of cerebral injury sustained by young children. This is most pertinent for areas of brain subserving functions that normally mature later in childhood, such as executive functioning within the frontal lobes.
A final guiding principle of rehabilitation in the pediatric patient is that intervention should begin as soon as possible. Coma is not a contraindication to the initiation of rehabilitation management strategies, and thus, once the patient is medically stable, therapy can and should be instituted, even while the child is still in the intensive care unit [Michaud et al., 1993]. This early phase of intervention is designed to limit maladaptive behavioral habits and movement patterns, and to prevent or at least minimize complications that can take months to resolve, if not properly and promptly addressed following the acute injury. Specific goals include: prevention of physical deformities, such as contractures due to abnormal spasticity and prolonged immobilization; maintenance of skin integrity; and reduction in the manifestations of dysautonomia secondary to fluctuations in muscle tone or bladder distinction [Noetzel, 2003].
Medical Aspects of Acute Pediatric Rehabilitation Management
Nutritional support is a key element of the acute management process [McLean et al., 1995]. In any child with an acquired nervous system injury, the risk for compromised nutrition is very high. There are a variety of causes that contribute to this risk, such as a hypermetabolic state resulting from systemic trauma and/or infection [Feldman et al., 1993]; delayed gastric emptying and intestinal dysmotility and stasis; oral pharyngeal dysfunction, which, even if recognized, can lead to aspiration pneumonia; and underestimation of caloric requirements necessary for repair and growth [Phillips et al., 1987]. Feeding through a nasogastric tube should be implemented while the patient is in the intensive care unit. An exception would be cases of major intra-abdominal injury; in these instances, hyperalimentation may be warranted. Oropharyngeal dysfunction and resultant swallowing difficulties secondary to bilateral cerebral hemisphere injury and/or lower cranial nerve involvement are an almost universal complication of acquired brain injury in the pediatric population [Bruce, 1990]. Thus, a detailed evaluation by a rehabilitation feeding team is mandatory [Logemann and Ylvisaker, 1998]. As neurological recovery proceeds, gradual introduction of oral feeding begins, with a commensurate decrease in supplemental alimentation based upon assessment of caloric need by a pediatric dietician.
Other medical issues often impact on acute neurorehabilitation management and, in addition, can impair the accuracy of on-going reassessment by the team of rehabilitation specialists. Fever and associated infection are a near-universal complication seen in pediatric patients with acute neurorehabilitation needs [Suz et al., 2006]. In those with intracranial trauma, the possibility of CNS infection must be considered [Bell et al., 1992]. In patients with penetrating cranial injury and basilar or compound skull fractures, the risk of bacterial meningitis and brain abscess is increased significantly. Sinusitis is a relatively common infection secondary to the frequent need for nasotracheal intubation or long-term nasogastric tube utilization. Other equally obvious sources of infection include indwelling urinary catheters and intravascular lines. Fever of central origin secondary to hypothalamic injury also has been documented in children with acquired brain injury. The setting commonly is one in which other clinical features of dysautonomia are observed, most notably elevated heart rate and blood pressure [Krach et al., 1997]. However, the diagnosis of fever of central origin is not a tenable one until all other sources of infection have been carefully evaluated and excluded, including drug-induced fever.
Dysautonomia, a syndrome characterized by simultaneous and paroxysmal sympathetic and muscle overactivity, typically follows severe traumatic, as well as other forms of acquired brain injury. The incidence varies between 5 and 12 percent in children [Blackman et al., 2004], its presence correlating with the most severe injury and the worst prognosis. Autonomic changes include marked tachycardia, hyperventilation, hypertension, fever, and increased sweating; motor changes include (decerebrate or decorticate) posturing, dystonia, rigidity, and spasticity [Pranzatelli et al., 1991]. Evidence-based treatment paradigms for dysautonomia in pediatric patients are nonexistent, with all evidence being anecdotal in nature. Gabapentin and bromocriptine have been reported to reduce the number and severity of paroxysms in pediatric patients [Russo and O’Flaherty, 2000], while in adults there is evidence that additional medications, such as propranolol, labetalol, clonidine, morphine, and midazolam, can be beneficial [Baguley, 2008]. Intrathecal baclofen also has been shown to be quite effective, even in children who are resistant to oral medications [Turner, 2003].
Disturbances in endocrine function, although less common than infection, also can complicate severe acquired brain injury [Einaudi and Bondone, 2007]. Damage to the pituitary and hypothalamic glands can produce the syndrome of inappropriate antidiuretic hormone secretion (SIADH), or conversely, central diabetes insipidus, in which ADH secretion is insufficient [Lin et al., 2009]. Although generally transient, both of these conditions mandate judicious fluid and electrolyte management, as well as pharmacologic treatment with desmopressin acetate in cases of diabetes insipidus.
Gastrointestinal disorders are also common in the pediatric population with acquired nervous system injury. These range from delayed gastric emptying and intestinal stasis to hemorrhage secondary to reflux esophogitis, gastric hyperacidity, and stress ulcers [Cochran et al., 1992]. Additionally, any child with a feeding tube is at risk for damage to the gastric mucosa. Thus, prophylactic management with H2 receptor blockers is indicated. Finally, in children with severe traumatic injuries involving the nervous system, there is a high incidence of skeletal fractures, typically of the long bones [Brown et al., 2006]. Such fractures may not be apparent early on in the comatose patient or in children with spinal cord injury, but reveal themselves during the early course of neurorehabilitation treatment [Sobus et al., 1993]. Close coordination between the orthopedic surgeons and the therapy team in regard to range of motion exercises and weight-bearing status is essential to optimize the response to therapy.
Comprehensive Pediatric Rehabilitation Programs

Over the last several years, eligibility criteria for admission to comprehensive neurorehabilitation services have been developed and refined (Box 105-2). At most major pediatric hospitals, these criteria include the following: a primary condition that is medically stable, but one that mandates intensive and multidisciplinary rehabilitation care; the premorbid physical and cognitive level of the child indicate potential for meaningful recovery; the patient is responsive to verbal or visual stimuli and has sufficient alertness to participate in the rehabilitation process; and the injury/illness diagnosis allows a reasonable expectation for improvement, with the potential to achieve clearly identifiable treatment goals within a reasonable period of time.
Management of Spasticity
Overview
Almost every single patient requiring acute neurorehabilitation services will manifest alterations in tone as a result of injury or illness. In addition, spasticity is a characteristic feature of many chronic motor disorders affecting infants, children, and adolescents. In the pediatric population, spasticity is most commonly found in children with hypertonic forms of cerebral palsy. However, spasticity can result from any disorder causing damage to or abnormal development of nerve cells or pathways controlling motor movements, either in the brain or in the spinal cord [Sanger et al., 2003]. Thus, spasticity is commonly observed in children with brain tumors and those with vascular, traumatic, infectious, and hypoxic injury to the brain or spinal cord.
In the early phase following CNS injury, absent or reduced tone typically is encountered; over time, the pattern often evolves into spasticity as one part of the upper motor neuron syndrome [Noetzel and Miller, 1998]. Spasticity is defined as an exaggerated response to passive movement of a limb, inducing a velocity-dependent involuntary resistance of the stretched muscle. Spasticity is characterized by excessive and inappropriately timed activation of skeletal muscles, which often interferes with a child’s ability to move voluntarily in a normal fashion. The clinical manifestations of spasticity vary, depending upon the sites of injury within the CNS. Extent of damage, as well as intrinsic characteristics of recovery, influences the presentation of spasticity. However, in almost all pediatric patients, spasticity will impair passive movement and static postural alignment [Noetzel and Miller, 1998]. In addition, impaired activation of voluntary movement, resulting in weakness and clumsiness, often may accompany spasticity [Tilton, 2009]. Spasticity must be differentiated from other manifestations of impaired movement, such as dystonia and rigidity, both of which typically occur within the context of an associated or a secondary injury due to ischemia and hypoxia [Sanger et al., 2003].
Spasticity to any significant degree interferes with a child’s control of voluntary movement, coordination, exercise tolerance, and range of motion in the joints. Invariably, this results in a state of excessive energy expenditure, compared to able-bodied children [Rose et al., 1990]. Spasticity typically impedes a child’s independence in activities of daily living, and may cause pain and disturb sleep. In the more severely affected individuals, patient care often is especially difficult. Over time, spasticity reduces protein synthesis in muscles and impairs longitudinal growth; the end result typically is permanent shortening of muscles (contractures) and the development of bony deformities [Lieber et al., 2004].
Treatment of spasticity should be predicated upon establishing goals that improve a child’s functional capabilities [Tilton, 2009]. Thus, the number one reason for attempting to decrease spasticity in the pediatric population is to improve functional movement, either upper-extremity use in activities of daily living, or leg and trunk movement to achieve ambulation. Improvement of head, neck, and trunk posture by reducing spasticity may have additional benefits in some children: namely, better oral feeding and breath support for speech. It must be recognized, however, that spasticity may impart advantages in certain individual cases, including maintenance of muscle bulk and tone; support of circulatory function; assistance in transfers and ambulation; and assistance in activities of daily living. Once the goals of treatment have been established, decisions can be made as to which treatment option is most likely to achieve the desired success (Figure 105-1).
Rehabilitation Therapy
The main focus of relieving spasticity in the earliest stages of rehabilitation is to minimize the complication of joint and muscle contractures, with a secondary goal of eliminating painful spasms [Michaud et al., 1993]. Management of spasticity in comatose or obtunded patients begins with proper positioning, range of motion exercises, and splinting, typically used in combination [Noetzel and Miller, 1998]. Potential exacerbating factors, including external sensory stimuli (such as a catheter leg bag), irritation of the skin, bladder or bowel distention, and occult fractures, should be sought and eliminated [Barnes et al., 1993]. Range of motion (ROM) should be carried out daily to all joints, regardless of alteration of tone. As recovery ensues, the ROM exercises can be combined with physical treatment modalities and specific affected muscles targeted. Physical agents, such as heat, cold, water, and electrical stimulation, are effective adjuncts in the treatment of spasticity, with few side effects or contraindications [Giebler, 1990]. These measures likely reduce spasticity through inhibition and fatigue-induced direct relaxation of the spastic muscle. An alternative mode of action is via facilitation of the antagonists of a spastic muscle, creating relaxation by reciprocal inhibition. Finally, reduction in pain due to the use of physical modalities also may be operant in the reduction of tone. The degree of benefit varies among patients, but the duration of effect is rather short and time-dependent upon the length of the application.
Positioning is designed to facilitate proper postural alignment and symmetry and to correct weight-bearing distribution throughout the body as a means of minimizing flexible postural imbalances. Tone-inhibiting techniques of positioning can use gravity to stretch spastic muscles and thus promote relaxation. In the latter stages of recovery, proper position can facilitate active contraction of functionally weak muscle groups [Hallenborg, 1990]. Proper therapeutic alignment of the body is designed to counter the abnormal position assumed by the body at rest or when moved. Various reflex patterns (such as the asymmetric tonic neck reflex – ATNR), which may become activated depending on the location and extent of CNS damage, often underlie abnormal trunk and neck postures. Thus, specific positions or exercises that promote the disinhibited reflexes should be avoided. For example, supporting the head and neck properly will minimize the head-turning movements that typically elicit the ATNR and which, in turn, can further disrupt positioning of the remainder of the body.
Other mainstays in the treatment of spasticity are casting and splinting, which are designed to prevent formation or worsening of contractures in a spastic extremity [Barnes et al., 1993]. Inhibitive casting may also result in a lowering of tone via one of several mechanisms, including the effects of neutral warmth and constant pressure on the spastic muscle; reduction in sensory input from cutaneous receptors, resulting in habituation; decrease in the sensitivity of muscle spindles to stretch; and inhibition of the excitability of gamma motor neurons [Feldman, 1990]. Splints or orthotics may be utilized in children who have progressed further in their recovery to produce functional benefit without necessarily altering spasticity (Figure 105-2). For example, an appropriately designed wrist and finger extension splint may improve functional hand activities, such as keyboarding, without altering the underlying tone. An extension of this approach is constraint-induced or forced-use therapy. A typical constraint protocol has two major components: constraint of the less involved upper limb, coupled with a behavioral program that repetitively encourages graded unilateral movements of the more poorly functioning arm and hand. Several randomized trials have documented increased dexterity and new motor skills acquisition, lasting for at least 6 months [Gordon et al., 2006; Deluca et al., 2006; Taub et al., 2004]. Experimental and clinical evidence seems to support the hypothesis that the damaged cortex and its connections can respond to sensory stimulation with sustainable and functional performance benefits, and that this response is a function of the enhanced plasticity of the young brain. Constraint-induced therapy clearly is a promising therapeutic approach in children with stroke-related hemiparesis from either prenatal or postnatal stroke [Pidcock et al., 2009].
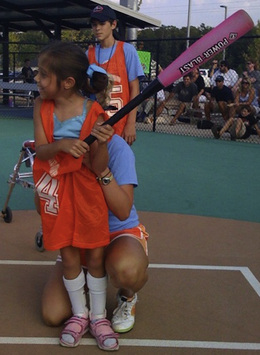
Fig. 105-2 Ankle–foot orthotics.
(Courtesy of Dr. Janice E. Brustrom-Hernandez and Anna Marie Champion)
In the latter stages of recovery, or in patients with chronic motor deficits such as cerebral palsy, equipment typically is a very important adjuvant to the rehabilitation process. Standing frames allow a child with reduced weight-bearing ability to be placed in an appropriate position to minimize the development of contractures. Other benefits to partial weight-bearing include improved bone maturation and better pulmonary function. In patients with cerebral palsy, deficits in both static and dynamic balance are common, and may prevent independent ambulation, even if spasticity is well controlled. Adaptive equipment, such as walkers and crutches, often is required (Figure 105-3); utilization of more creative assistive devices, especially when applied to recreational activities, has further enhanced the independence of patients with motor disability Figure 105-4).
Oral Medications
Pharmacologic agents are an additional treatment modality for the management of spasticity in the acute and chronic pediatric neurorehabilitation patient. Despite the fact that a large number of antispasticity medications have been available for many years, data currently available (Table 105-1) are too limited to conclude that any of these agents is truly effective in reducing spasticity in the acutely brain-injured pediatric patient. Much of the problem derives from the lack of the multicenter protocols that typically are necessary to answer these sorts of questions in an adequate fashion. In patients with spastic cerebral palsy, data on efficacy with regard to tone reduction are better. However, few appropriately designed studies have examined the role of oral antispasticity medication in improving functional capability in a child with cerebral palsy. Moreover, the side effects of antispasticity medication in the pediatric population give cause for concern. Thus, antispasticity medication should be utilized only when severe spasticity cannot be adequately managed by other therapeutic modalities. In addition, continued use of any oral antispasticity medication clearly must be justified by on-going demonstrable benefit to the individual patient. Finally, antispasticity medication should be prescribed in those situations in which meaningful functional goals, such as greater independence in activities of daily living or improved ambulation, can be achieved, as opposed to mere clinical ones, i.e., decreased resistance to stretch or a reduction in clonus. Notable exceptions to the above “rule” include the institution of an oral medication to facilitate hygiene, improve appropriate positioning, reduce the requirements of care-giving, and ease rehabilitation procedures, such as proper fitting of orthotics.
Commonly employed medications treating spasticity in the pediatric population include diazepam (and other benzodiazepines), baclofen, tizanidine, and dantrolene (see Table 105-1) [Whyte and Robinson, 1990; Tilton, 2009]. The benzodiazepines act as postsynaptic gamma-aminobutyric acid A (GABA-A) agonists within the brain and spinal cord. Both high- and low-affinity receptors have been identified, impacting on the speed and rate of response, as well as the degree and duration of unwanted side effects. The use of diazepam, especially in acute brain-injured patients, is severely limited by its sedative side effects, often producing under-arousal and impaired attention, even at relatively low doses. However, in double-blind, placebo-controlled studies in patients with cerebral palsy, diazepam produced significant reduction in spasticity, especially in younger children and those with athetosis [Pranzatelli, 1996]. Studies also indicate that the beneficial effects of diazepam in combination with dantrolene are additive [Nogen, 1979]. Other side effects include dizziness, mild weakness, confusion, memory impairment, and a withdrawal syndrome that often overshadow any perceived clinical benefit [Gracies et al., 1997]. Clorazepate and clonazepam also have demonstrated antispasticity action in adult patients. Limited studies of these medications in children with spasticity mainly have documented the adverse effect of intolerable sedation [Gracies et al., 1997]. An evidence-based practice parameter on the medical management of spasticity in children with cerebral palsy has recently been published [Delgado et al., 2010].
Baclofen is a presynaptic inhibitor of GABA-B. As such, baclofen seems much better tolerated, since its mode of action appears to be via polysynaptic inhibitory pathways, mainly within the spinal cord [Price et al., 1984]. Baclofen has been moderately effective in reducing spasticity secondary to acquired spinal cord injury, with reduced efficacy in brain injury from trauma or stroke. The doses required to achieve this result have been quite variable (see Table 105-1). In the chronic motor disturbance of cerebral palsy, placebo-controlled, double-blind crossover studies have reported significant reduction in spasticity and improved use of extremities with oral baclofen [Milla and Jackson, 1977; Pranzatelli, 1996]. The adverse effects of baclofen include sedation, weakness, confusion, fatigue, hypotonia, nausea, and dizziness. Use of baclofen may potentiate antihypertensives, and sudden withdrawal of the medication has been associated with seizures, hallucinations, and rebound spasticity.
Tizanidine is a newer anti-spasticity medication that acts as an α2-agonist. Its mechanism of action is via inhibition of the release of excitatory amino acids from spinal interneurons, and facilitation of the action of glycine at spinal and supraspinal levels [Milanov and Georgiev, 1994]. Tizanidine also causes inhibition of facilitatory ceruleospinal pathways, and in animal studies it has been documented to have antinociceptive properties. While there have been numerous controlled studies in adults with spasticity of multiple etiologies, studies of tizanidine in children are few [Tilton, 2009]. In a single open-label study in 30 children with spastic diplegic cerebral palsy, motor ability was improved at moderate doses (1–2 mg three times a day.) [Brin et al., 1999]. Side effects include drowsiness, dizziness, and dry mouth, and less frequently, hallucinations and orthostatic hypotension (see Table 105-1) [Gracies et al., 1997]. Tizanidine is metabolized by the liver, so its use should be avoided in patients with known hepatic disease. Clonidine also is an α2-agonist and the predecessor of tizanidine, with a similar side-effect profile [Dall et al., 1996]. Its usefulness as an antispasticity agent is limited, mainly due to poor tolerability, bradycardia, and hypotension. However, in a patient with an acute brain injury resulting in hypertension, tachycardia, and autonomic dysfunction, clonidine may be extremely beneficial.
Dantrolene sodium is the least sedating of the antispasticity medications, since its site of action is within the muscle. This agent suppresses the release of calcium ions from the sarcoplasmic reticulum, thus uncoupling electrical excitation from muscle contraction. Despite proven efficacy in placebo-controlled studies of children with cerebral palsy [Haslam et al., 1974; Joynt and Leonard, 1980], use of dantrolene is limited by the need for careful titration to minimize the potential for severe generalized weakness. Nausea and diarrhea are common side effects. In addition, liver function tests must be carefully monitored and the duration of treatment limited because of the risk of potential hepatic injury [Katz, 1988].
Concerns regarding efficacy and adverse side effects from the more standard antispasticity medications have prompted investigation of newer agents. Gabapentin has been reported to reduce Ashworth Scale scores in adults with multiple sclerosis [Mueller et al., 1997], and in adults with spinal cord injury, the medication can result in modest clinical improvement of spasticity [Priebe et al., 1997]. Pregabalin, also a GABA analogue, may be of value in the treatment of spasticity [Bradley and Kirker, 2008]. However, presently, there are no published reports on either of these medications in children.
In contrast, modafinil has been investigated in children with cerebral palsy, with early case reports suggesting improved gait speed and Ashworth scores. More recently, however, a randomized double-blind AB/BA crossover trial to evaluate the effect of oral modafinil versus placebo did not find evidence that modafinil reduced spasticity nor had a positive impact on quality of life in children with spastic cerebral palsy [Murphy et al., 2008].
Neuromuscular Blockade: Alcohol, Phenol, and Botulinum Toxin Injections
Neuromuscular blockade (alternatively labeled chemodenervation) can be achieved through the perineural injection of phenol or ethyl alcohol, or intramuscular injection of botulinum toxin to decrease nerve–muscle transmission. These forms of injectable therapy are best suited for targeting especially problematic muscles in children with generalized spasticity or for focal spasticity. While phenol and alcohol both have been employed in pediatric patients with cerebral palsy, rigorous testing has not been carried out [Tilton, 2003]. The concentration of phenol (typically 3–5 percent) and alcohol (30–55 percent), when placed perineurally, causes axonal degeneration and thus denervation, with tone reduction lasting from a few weeks to 1–3 years. Despite this favorable duration of benefit, chemodenervation with phenol and alcohol is used relatively infrequently, mainly due to the significant risk of pain and paresthesia. In addition, the procedure mandates sedation or anesthesia, since electrical stimulation is necessary to identify the target nerve, thus further minimizing its attractiveness to the parents of young children.
In contrast, botulinum toxin is increasingly used in managing severe spasticity that involves only a few muscles or muscle groups [Tilton, 2003]. A variety of studies, mainly on children with cerebral palsy, have documented reduction in spasticity and modest functional improvement in injected muscle groups, both in the arms and legs [Edgar, 2001; Fehlings et al., 2000; Steenbeek et al., 2005]. Patient ages range from 12 months to late teens, with muscle size being the most important determinant in patient selection (Box 105-3). Intramuscular injection of botulinum toxin reduces spasticity by inhibiting the release of the neurotransmitter (acetylcholine) responsible for transforming nerve impulses into muscle contraction. As the effects of botulinum injections are local, dose-dependent, and temporary, this treatment is best utilized in those patients who have specific “problem muscles” that interfere with major functional aspects of living, such as ambulation or upper-extremity movement. The limitation in muscle movement must be the result of spasticity and not of a fixed contracture. In addition, there should be sufficient underlying strength so that weakening of the problem muscle does not produce paresis. A clinically apparent affect is most notable 2–4 weeks after injection, and on-going benefit typically lasts 3–4 months or longer, depending on the severity and the particular muscles involved. The total dose of botulinum that can be administered at a single point in time is limited. In most centers, botulinum toxin serotype A is utilized, and typically, the total maximum dose is between 12 and 16 units/kg [Tilton, 2009]. Therefore, only a few select muscles or muscle groups can be injected. In addition, there must be appropriate spacing between injections (even to different muscles) in order to minimize the development of antibodies to the toxin. None the less, judicious use of this agent, often in patients intolerant of splinting or with severe postural deformities, can be a beneficial adjunct to maintain range of motion. The vast majority of children with spasticity secondary to acquired nervous system injury will demonstrate significant motor recovery and lessening of spasticity within 6–12 months [Noetzel and Miller, 1998]. In these patients, the reversible denervation caused by botulinum toxin on the neuromuscle junction must be viewed as a positive attribute. In children with chronic motor disorders, such as spastic cerebral palsy, serial injections often are required, typically every 4–6 months. Botulinum injections can be employed with other therapies, including intrathecal baclofen and dorsal rhizotomy (see below). Botulinum injections typically are coupled with an intensification of therapy and/or serial casting in order to secure maximum benefits from the injections [Booth et al., 2003; Newman et al., 2007]. Systemic side effects are rare, but pain on injection and a transient flulike syndrome have been noted. More recently, there have been reports of severe systemic botulism following injections of both high and medium doses, resulting in deterioration in respiratory and oral-motor function [Crowner et al., 2007; Howell et al., 2007].
Intrathecal Baclofen Therapy
In patients with more severe or extensive spasticity, intrathecal baclofen (ITB) therapy can be employed to reduce tone. The components of an ITB system include a pump implanted under the skin on the abdomen, which infuses the drug at a predetermined rate; a catheter, which delivers the drug to the intrathecal space of the spinal cord; and a programmer, which allows for adjustable and precise dosing. ITB therapy should be considered in pediatric patients whose severe spasticity interferes with function or care, and those who have painful spasticity-related spasms (Box 105-4). In addition, children and adolescents who are refractory to other treatments or unable to tolerate side effects from oral medications are also reasonable candidates. As the baclofen is delivered directly to the cerebrospinal fluid and diffuses into the spinal cord to act at GABA-B receptor sites, the doses administered are much lower than those taken orally, and the potential for systemic side effects is reduced. ITB formerly was utilized mainly in patients with predominantly lower-extremity spasticity [Albright et al., 1993]; more recent emphasis has been on children with quadriplegic cerebral palsy and mixed cerebral palsy with dystonia [Gilmartin et al., 2000; Van Schaeybroeck et al., 2000]. In those patients, the catheter tip typically is placed between T2 and C6 for maximum efficacy.
ITB therapy is not the optimal treatment if spasticity affects predominantly the arms; trunk control is poor; spasticity is focal or not severe; and families cannot fully commit to the requirements of ITB therapy maintenance (see Box 105-4). The pump reservoir must be refilled percutaneously every 2–6 months, depending on the daily dose, and the battery life of 5–7 years mandates replacement of the entire pump at the end of that time. Contraindications in patient selection include an allergic (hypersensitivity) reaction to oral baclofen, unrealistic expectations of benefit, and small size/low weight. In most centers, a child must weigh a minimum of 30–40 pounds so as to accommodate the size of the pump in the abdominal pocket. Spinal fusion also is a relative contraindication with respect to typical surgical approach.
ITB therapy complications due to surgical implantation and refilling procedures have been documented, the most worrying of which are CNS infection and peritonitis. Complications from components of the device, i.e., catheter and pump, are also encountered (Box 105-5). Even though the dose of intrathecal baclofen is lower than for oral medication, side effects, such as somnolence, dizziness, headache, and hypotonia, have been reported. Signs of overdose may range from lightheadedness, itching, excessive weakness and ascending low tone to respiratory depression, seizures, and loss of consciousness. Withdrawal from the medication is a potential medical emergency, presenting as altered mental status, paresthesias, exaggerated rebound spasticity, and muscle rigidity that may advance to a rhabdomyolysis and organ failure, causing death [Alden et al., 2002]. A key element in early diagnosis is the combination of fever and pruritus. Management of withdrawal is predicated on prompt recognition, followed by close observation and institution of oral baclofen or intravenous benzodiazepine administration, both of which are beneficial, but with variable success, particularly in severe cases. One set of investigators postulated that ITB withdrawal might be a form of serotonergic syndrome that occurs from loss of GABA-B receptor-mediated presynaptic inhibition of serotonin. In their study, cyproheptadine was a useful adjunct to baclofen and benzodiazepines in the management of acute ITB withdrawal syndrome [Meythaler et al., 2003].
Selective Dorsal Rhizotomy
Selective dorsal rhizotomy (SDR) is a neurosurgical technique performed on the lower spinal cord; it is designed to reduce moderate to severe lower-extremity spasticity by minimizing aberrant muscle spindle input believed to trigger muscle overactivity. Bone covering the first two lumbar segments of the spine is removed. Individual sensory nerve roots are identified, selected afferent fibers in the dorsal roots from L1 to S2 are dissected, and those rootlets that demonstrate excessive activity, as determined by electromyography (40–60 percent of the rootlets in the L4–S1 distribution), are severed [Park et al., 1993]. Postoperatively, the child is enrolled in an intensive program of physical therapy. Clinical studies have demonstrated that the combination of SDR and physical therapy is beneficial in reducing spasticity in children with spastic diplegic cerebral palsy [Engsberg et al., 2006]. Furthermore, randomized studies of SDR have demonstrated that SDR produces gains in strength, gait speed, and overall gross motor function, with evidence that the procedure may be most efficacious in marginal ambulators [McLaughlin et al., 1998; Wright et al., 1998]. The benefit to gross motor function shows a direct relation between the percentage of dorsal nerve roots transected and functional improvement [McLaughlin et al., 2002]. In view of the surgical approach in SDR, concern has been raised about the long-term risk of spinal deformity and an adverse effect on bladder function. Most studies, however, have suggested that these risks are minimal when the surgery is carried out using the limited two-segment laminectomy. Persistent loss of strength following SDR has not been objectively demonstrated [Engsberg et al., 1999].
Patients most suitable for SDR are those with spastic diplegia or mild quadriplegia who have some degree of ambulatory capability with isolated leg movements, good strength, and trunk control [Engsberg et al., 1999; Chicoine et al., 1996], but whose full potential is limited by lower-extremity spasticity (Box 105-6). Most of these individuals have a history of prematurity or evidence of periventricular leukomalacia on imaging studies. Surgery typically is carried out on children between the ages of 4 and 10 years [Tilton, 2009]. Unsuitable candidates for SDR include those whose lower-extremity spasticity is a result of congenital or neonatal CNS infection and head trauma or hypoxic brain injury after the newborn period (Box 105-7). In addition, children with very weak or low-tone abdominal muscles and those who have predominant dystonia or ataxia, as well as those with limited potential for functional gains, are less likely to benefit from an SDR.
Orthopedic Surgery
Even with optimal treatment, spasticity may progress to cause muscle contractures and bony deformities. Thus, children with spasticity may require orthopedic surgical intervention. The most common orthopedic procedures include tendon releases for fixed contractures at the ankle, surgery to correct hip subluxation or dislocation, and instrumentation for scoliosis. The benefit of orthopedic surgery is most pronounced in patients who have achieved some degree of skeletal maturation (typically between ages 6 and 12 years) [Renshaw et al., 1996]. A single multilevel surgical intervention, if feasible, is most advantageous, as opposed to multiple procedures, each with their individual postsurgical recovery time. All of these surgical interventions require intensification of a physical therapy program to strengthen and improve the range of motion in the surgically improved extremity.
Acquired Brain Injury
A major source of pediatric disability and medical expense in the United States is acquired brain injury; typically, it is the most common condition necessitating treatment in a rehabilitation setting. Traumatic brain injury is the most common diagnosis mandating admission to an acute neurorehabilitation service [Noetzel and Miller, 1998]. When combined with stroke, hypoxic and ischemic brain injury, brain tumor, and CNS infection, acquired brain injury represents about 75 percent of all pediatric admissions.
Rehabilitation of a child with acquired brain injury often is complicated by the nature of the cerebral damage. Even though the primary injury may be focal, damage to the brain typically is distributed through multiple locations in most patients. In addition, secondary injury from cerebral edema, hypoxia, or ischemia, often in the setting of elevated intracranial pressure, may inflict more diffuse damage. Infants and young children, especially those with nonaccidental traumatic brain injury secondary to abuse are particularly susceptible to this process [Noetzel and Miller, 1998]. Although the pathology of acquired brain injury is quite variable, certain neurorehabilitation issues, including spasticity, require attention in almost every child recovering from this type of injury.
Behavioral Disturbances
Behavioral aberrations are a nearly universal consideration and can be observed in all stages of recovery, once coma resolves [Chorazy et al., 1998; Ylivsaker et al., 1999]. Agitation, characterized as nonpurposeful, random, motor and verbal activity, is fairly common and should be anticipated in the initial phase of recovery. Emotional regression and impaired information processing likewise are often recognized early on; like agitation, their resolution may be painfully slow, persisting for weeks or even months, depending on the severity of brain injury. Sedative medications usually are without benefit and, in fact, the symptoms may be aggravated by further clouding the sensorium. Therapists and family members should strive to prevent these behavioral disturbances from evolving into learned patterns that can minimize the long-term prospects of recovery and reduce the response to treatment.
Exceedingly limited understanding of deficits, in association with an intolerance to sensory stimulation, often is observed in the middle stages of recovery [Ylivsaker et al., 1999]. Hyperactivity and impulsive or even destructive behavior are the typical manifestations seen in a child between the ages of 3 and 8 years; in older children and adolescents, the behavioral theme is one of decreased motivation and poor initiation of action, combined with lethargy. Therapeutic intervention and management strategies require on-going careful assessment of the patient’s attention and arousal patterns. One approach is to transform the child’s environment to a more familiar one that additionally provides appropriate structure. Any medications with the potential for adverse cognitive or behavioral side effects should be withdrawn when possible. Since many medications may have less predictable side effects in pediatric patients with acquired brain injury, this task may be quite challenging [O’shanick, 1998]. None the less, it is essential to reassess the need for any medication constantly in these children. In addition, careful modulation of a child’s surroundings may be beneficial, with the goal of avoiding either overstimulation or prolonged sensory deprivation. Some patients appear to respond to a constancy of the auditory background, i.e., so-called white noise, while for others it may be necessary to minimize noise or activity in their environment. Alerting, orienting, and preparing a child for any therapy activity is important, especially if consistency can be maintained in how the child is approached or the environment modulated. In addition, it is essential to monitor the child’s response so as to determine whether or not environmental adaptations are actually beneficial [Ylivsaker et al., 1999].
Despite appropriate manipulation of the environment, there are children with acquired brain injury in whom aberrant behavior persists. In such instances, medication may need to be prescribed, recognizing that use of pharmacologic agents to manage behavior in this clinical setting has not been investigated in a scientifically rigorous manner [Levy et al., 2005; Francisco et al., 2007]. The proposed benefit of most medications is derived from a limited number of studies, typically involving only adult patients, and carried out in a non-blinded fashion and without appropriate controls. For some agents, efficacy is extrapolated from literature on how the medication has benefited patients with primary psychiatric disorders or, conversely, mental retardation. The most pressing behavioral issues in the pediatric population usually are aggression and severe agitation. Reasonable medications to try in these situations include beta blockers, carbamazepine, valproate, and tricyclic antidepressants; others would advocate clonidine, amantadine, and buspirone [Connor and Steingard, 1996; Krach and Kriel, 1999; Francisco et al., 2007]. Finally, stimulants may have a role in children with under-arousal, inattention, and slow cognitive processing, especially those with premorbid attention-deficit hyperactivity disorder (ADHD) [Whyte et al., 2004; Levin et al., 2007].
Communication and Cognitive Deficits
Deficits in communication and cognition are exceedingly common after brain injury and represent the largest cause of long-term disability in these patients. Although a variety of cognitive impairments are observed, the most common affect memory, arousal, attention, and learning of new information. Certain intellectual deficits, such as executive functioning, may not become apparent until years after injury, when they fail to emerge at the expected developmental age [Dombovy, 1998]. In the early phase of pediatric rehabilitation, establishing an appropriate level of arousal and sustained attention is essential in furthering recovery. Agents that act upon the cholinergic, adrenergic, dopaminergic, and serotonergic neurotransmitter systems commonly are employed in the pharmacologic management of under-arousal and/or reduced attention [Connor and Steingard, 1996; Krach and Kriel, 1999; Levy et al., 2005]. Tricyclic antidepressants and dopaminergic agents, commonly amantadine, also have been utilized [O’Shanick, 1998; Williams, 2007]. While not overwhelming, the results do demonstrate a likely overall beneficial impact on arousal/attention. Unfortunately, there are no credible published reports regarding the efficacy of pharmacologic intervention in children or adolescents, with respect to memory and learning of new information [Arciniegas and Silver, 2006].
There are several fundamental principles that guide therapeutic interventions for communication and cognitive impairment. First, as is true for spasticity, therapy should be initiated in the acute care setting, where the main focus is on providing appropriate sensory modulation. Next, cognitive retraining should pervade all aspects of the rehabilitation process, including time spent with nursing, music therapist, and child-life specialists. For example, during any therapeutic or even medical activity, informal memory training can be attempted. Finally, rehabilitation should be predicated not only on enhancing preserved function, but also on remediating residual deficits [Ylivsaker et al., 1999]. Continual reassessment of cognitive and linguistic capabilities, utilizing standardized tests, is essential. Based on these results, a systematic structured program of therapeutic interventions can be framed. Sensory deficits, especially auditory and visual difficulties, may impair the response to therapy, as well as impact the neurological assessment. In any child with an acquired brain injury, high vigilance for these clinical problems is indicated and, when appropriate, evaluations (such as audiograms, brain auditory-evoked potentials, visual-evoked responses, ophthalmologic consult, and so on) should be requested.
Post-Traumatic Seizures
Seizures resulting from brain trauma commonly occur within the first 7–10 days following injury [Kennedy and Freeman, 1986]. The frequency of early seizures is higher in children than adults, with an 8–10 percent incidence in the pediatric population [Annegers et al., 1980; Emanuelson and Uvebrant, 2009]. The risk is highest in young children under the age of 3 years; pediatric patients of any age with a severe injury; and in those with nonaccidental brain injury [Gilles and Nelson, 1998; Appleton and Demellweek, 2002; Chiaretti et al., 2000]. However, most early seizures follow relatively minor head injury, often within minutes of the trauma. In contradistinction, the risk for late post-traumatic epilepsy (PTE) is lower in children compared to adults. In pediatric patients with mild to moderate traumatic brain injury, the incidence of PTE is less than 1 percent [Annegers et al., 1980]. Even in children with severe traumatic brain injury, the incidence of post-traumatic epilepsy is roughly half that observed in adults [Appleton and Demellweek, 2002; Emanuelson and Uvebrant, 2009]. In the pediatric population, severity of traumatic brain injury, as well as the presence of early seizures, seems to be the most important predictive factor for PTE [Hahn et al., 1988; Emanuelson and Uvebrant, 2009]. Other risk factors include intracranial hemorrhage, focal neurological signs, and nonaccidental traumatic brain injury [Barlow et al., 2000].
Early seizures, especially those within days of the acute injury, typically do not mandate treatment. In recurrent early seizures, short-term anticonvulsant therapy, typically with phenytoin or, more recently, levetiracetam, may be indicated, especially in patients with raised intracranial pressure [Ates et al., 2006]. The goal of treatment is to suppress further seizures that may cause additional cerebral edema secondary to seizure-induced acidosis and increased blood flow [Kennedy and Freeman, 1986]. Initiation of anticonvulsant therapy also may be indicated in patients with early seizures and additional risk factors for late seizures, such as penetrating cerebral injury, intraparenchymal hemorrhage, and penetrating cerebral injury [Ates et al., 2006]. There is, however, no convincing evidence in children that prophylactic therapy is effective in preventing the development of PTE [Chang and Lowenstein, 2003]. As a result, there is little justification for routine prophylactic anticonvulsant administration in children, especially given concerns regarding potential adverse medication effects on cognition and behavior. In children started on anticonvulsants utilized to suppress additional early seizures, duration of treatment should be limited to between 7 and 14 days [Ates et al., 2006]. Even in the more severe cases of traumatic brain injury, most antiepileptic drugs can be tapered prior to discharge, unless multiple focal seizures have occurred. In these latter patients, a follow-up electroencephalogram (EEG) should be obtained; if epileptiform activity is not seen, then tapering of the anticonvulsant is initiated. For patients with persistent focal epileptiform discharges, longer-term treatment (typically 6–12 months) with carbamazepine often is necessary [Barlow et al., 2000].
Spinal Cord Injury
The incidence of acute spinal cord injury (SCI) in the United States is estimated to range between 30 and 40 cases per million individuals per year, with approximately 20 percent occurring in patients under the age of 20 years [Zidek and Srinuvasan, 2003]. As is true for adults, trauma accounts for the vast majority of SCI in children, but other disorders, such as demyelination, infarction, and tumors causing cord compression, also contribute to cases of myelopathy. The neurological examination of a child with suspected SCI consists of two main components: a sensory evaluation of dermatomes, testing each bilaterally for dull and light touch and pinprick; and assessment of strength bilaterally in key muscles in both the arms and legs. An understanding of motor movement and reflexes, as they relate to spinal level, obviously aids in the examination (Table 105-2). The level of injury is assigned as the lowest segment at which sensory and motor responses are intact bilaterally, although the levels may differ from side to side. In addition, the American Spinal Injury Association (ASIA) provides criteria defining the extent of injury (Box 105-8). This classification process not only informs rehabilitation decisions, but also has predictive value. The ultimate outcome in SCI, with respect to mobility and independence in activities of daily living, correlates with the level of functional motor activity. Recovery is usually maximal within the first 6 months following injury, regardless of whether damage to the spinal cord is classified as incomplete or complete [Yarkony, 1997]. Similar to the situation in acquired brain injury, there are certain medical and rehabilitation issues, including spasticity, that must be addressed in most children recovering from SCI.
Box 105-8 American Spinal Injury Association Classification of Spinal Cord Injury
A | Complete: No motor or sensory function preserved, including sacral segments S4-S5 |
B | Incomplete: Sensory but no motor function below the neurologic level, including the sacral segments S4–S5 |
C | Incomplete: Motor function is preserved below the neurologic level, and more than half of the key muscles have a muscle grade <3 |
D | Incomplete: Motor function is preserved below the neurologic level, and at least half of the key muscles below the neurologic level have a muscle grade >3 |
E | Motor and sensory functions are normal |
Pulmonary Issues
Pulmonary complications, such as ventilator failure, pulmonary edema, pneumonia, aspiration, and atelectasis, are a major source of morbidity and mortality in the acute stage of SCI [Zidek and Srinuvasan, 2003]. Patients with high cervical injuries (C1–C4) typically require lifelong ventilator support, since only the muscles of respiration (innervated by cranial nerve XI and C2–C4) have any degree of activity. Because the diaphragm, the major muscle of inspiration, is powered by C3–C4, individuals with injuries from C3–C5 have varying degrees of ventilator compromise, while injuries at the C6–C7 level usually allow for eventual discontinuation of ventilator support. Lack of appropriate progress may be a clue to additional weakness from direct injury to the thorax and abdomen. Aggressive pulmonary management, including postural drainage, cough assist, suctioning, chest percussion, and early mobilization, is essential in minimizing the development of chronic lung disease.
Autonomic Dysreflexia
Autonomic dysreflexia occurs in up to 70 percent of individuals with SCI [Blackmer, 2003]. It represents a syndrome of sympathetic overactivity in patients with SCI at or above the T6 level. Almost any noxious stimulus below the lesion produces a generalized sympathetic discharge that no longer can be inhibited by brainstem-mediated signals. As a consequence, vasoconstriction occurs below the level of injury, with resultant hypertension. Above the lesion, unopposed parasympathetic output creates excessive vasodilatation with hyperhydrosis, nasal congestion, flushing, and headache. The most common precipitating factors include bowel and bladder distention, infection, and pressure sores. Effective management begins with education of the patient and caregivers, and avoidance of causative stimuli [Blackmer, 2003]. Hypertension is an obvious source of morbidity, and may require pharmacologic treatment with agents such as nifedipine and captopril [Baguley, 2008].
Heterotopic Ossification
The incidence of heterotopic ossification occurs in 3–10 percent of children with SCI [Zidek and Srinuvasan, 2003]. It represents a pathologic process in which true bone formation takes place in aberrant locations, such as soft tissues or joints, most commonly involving the proximal limbs and axial musculature, but always distal to the spinal injury level [Shehab et al., 2002]. The onset of symptoms ranges between 1 and 4 months, and characteristically includes erythema, swelling, fever, and decreased range of motion. The pathophysiology of heterotopic ossification is poorly understood, but likely involves transformation of mesenchymal cells to cells with genetic machinery capable of producing bone [Kluger et al., 2000]. Diagnosis typically is based upon an elevated alkaline phosphatase level in the appropriate clinical setting. Confirmation by bone scan often is necessary, as plain radiographs may lag behind a patient’s symptoms by as much as 4–6 weeks. Treatment of heterotopic ossification includes salicylates (60 g/kg daily) for 6–8 weeks, which do appear to prevent continued growth of the ossified tissue if started promptly. Other medications, such as corticosteroids, indomethacin, and biphosphonates, have been beneficial in adults. Their use in the pediatric population has been tempered by concerns of potentially significant adverse effects [Mital et al., 1987; Kluger et al., 2000]. Surgical excision is warranted if the affected area impedes rehabilitation, but the timing of the operation is critical. Risk of recurrence is minimized by allowing full maturation of the heterotopic ossification, which usually evolves during the first 2 years after injury.
Other Medical Issues
In children with SCI, other major concerns in medical care include hypercalcemia, deep vein thrombosis (DVT), prevention of pressure ulcers, and avoidance of joint contractures. Hypercalcemia, resulting from bone absorption, may be seen in up to 25 percent of patients within 4 months of injury [Zidek and Srinuvasan, 2003]. Symptoms of nausea, emesis, anorexia, and lethargy are common, especially when calcium levels rise above 12 mg/dL. Conservative treatment consists of prompt mobilization, fluid hydration, and, when necessary, diuretics to increase renal excretion of calcium. Both calcitonin and etidronate also have been beneficial when standard measures fail [Meythaler et al., 1993].
Children with SCI are at increased risk of DVT. In adolescents between the ages of 15 and 18 years, the incidence of DVT is 10 percent, which is substantially lower than that found in adults. Typically, thrombosis occurs within the first 3 months following injury. Symptoms include warmth, swelling, tenderness, and discoloration in the affected extremity. Detection of DVT usually can be accomplished with noninvasive ultrasonography. In patients with SCI, thromboprophylaxis is recommended for the first 2–3 months after injury, although the data are more compelling in adults compared to children [Ploumis et al., 2009]. Low-molecular-weight heparin therapy recently has gained favor for prophylaxis and treatment of DVT in pediatric patients, with monitoring of antifactor Xa levels.
Rehabilitation Strategies
In children, the profound multisystemic disruption created by an SCI is exacerbated secondary to impairment of development, physical growth, and psychological maturation. In many ways, an SCI sustained in childhood can produce greater disability than a similar injury in an adult. In addition to the profound multisystem trauma that typically occurs in an SCI, in a child there often is secondary impairment created by the disruption of normal development, physical growth, and psychosocial maturation. While there are rehabilitation goals dictated by specific deficits in bodily function, the overriding aim is to treat the needs of the whole child, thereby maximizing the potential for independence later in life. Patients with high cervical injuries (C1–C4) require significant adaptive equipment, utilizing oral-motor and head movements to activate switches, in addition to ventilatory support. In most children with cervical cord injuries, a power wheelchair is indicated, with the method of control determined by the level of injury. For example, a child with a C6 injury level can activate the biceps and is capable of flexion at the elbow, as well as wrist extension. Appropriate splinting and orthotics may facilitate greater independence in activities such as feeding and upper-extremity dressing in children. With slightly lower injury levels, patients have adequate arm extension and improved hand function, which are critical for wheelchair propulsion (Figure 105-5), the ability to self-transfer, and participation in bowel and bladder training programs. Programs and equipment designed to assist ambulation also are predicated on the level of injury and residual muscle function in the trunk and legs (see Figure 105-5) [Zidek and Srinuvasan, 2003]. Finally, in all patients, regardless of injury level, pressure relief is necessary, and patients, as well as their caretakers, need to be instructed in this maneuver so as to minimize the development of decubitus ulcers.
References
The complete list of references for this chapter is available online at www.expertconsult.com.
Albright A.L., Barron W.B., Fasick M.P., et al. Continuous Intrathecal Baclofen Infusion for Spasticity of Cerebral Origin. JAMA. 1993;270(20):2475-2477.
Alden T.D., Lytle R.A., Park T., et al. Intrathecal baclofen withdrawal: A case report and review of the literature. Childs Nerv Sys. 2002;18:522-525.
Annegers J.F., Grabow J.D., Groover R.V., et al. Seizures after head trauma: a population study. Neurology. 1980;30:683-689.
Appleton R.E., Demellweek C. Post-traumatic epilepsy in children requiring inpatient rehabilitation following head injury. J Neurol Neurosurg Psychiatry. 2002;72(5):669-672.
Arciniegas D.B., Silver J.M. Pharmacotherapy of posttraumatic cognitive impairments. Behav Neurol. 2006;17(1):25-42.
Ateş O., Ondül S., Onal C., et al. Post-traumatic early epilepsy in pediatric age group with emphasis on influential factors. Childs Nerv Syst. 2006;22(3):279-284.
Baguley I.J. Autonomic complications following central nervous system injury. Semin Neurol. 2008;28:716-725.
Barlow K.M., Spowart J.J., Minns R.A. Early post-traumatic seizures in non-accidental head injury: relation to outcome. Dev Med Child Neurol. 2000;42(9):591-594.
Barnes M., McLellan L., Sutton R. Spasticity. In: Greenwood R., Barnes M.P., McMillan T.M., et al, editors. Neurological Rehabilitation. Edinburgh: Churchill Livingston; 1993:161-172.
Bell L.M., Baker M.D., Beatty D. Infections in severely traumatized children. J Pediatr Surg. 1992;27(11):1394-1398.
Bickenbach J.E., Chatterji S., Bradley E.M., et al. Models of disablement, universalism and the international classification of impairments, disabilities and handicaps. Soc Sci Med. 1999;48:1173-1187.
Blackman J.A., Patrick P.D., Buck M.L., et al. Paroxysmal autonomic instability with dystonia after brain injury. Arch Neurol. 2004;61:321-328.
Blackmer J. Rehabilitation medicine: 1. Autonomic dysreflexia. CMAJ. 2003;169(9):931-935.
Booth M.Y., Yates C.C., Edgar T.S., et al. Serial casting vs. combined intervention with botulinum toxin A and serial casting in the treatment of spastic equinus in children. Pediatr Phys Ther. 2003;15:216-220.
Bradley L.J., Kirker S.G. Pregabalin in the treatment of spasticity: a retrospective case series. Disabil Rehabil. 2008;30(16):1230-1232.
Brin I.L., Kurenkov A.L., Gotlib V.I. [The use of sirdalud in cerebral palsy in children]. Zh Nevrol Psikhiatr Im S S Korsakova. 1999;99:30-33.
Brown J.K., Jing Y., Wang S., et al. Patterns of severe injury in pediatric car crash victims: Crash Injury Research Engineering Network database. J Pediatr Surg. 2006;41(2):362-367.
Bruce D.A. Head injuries in the pediatric population. Curr Prob Pediatr. 1990;20(2):61-107.
Carmichael S.T. Cellular and molecular mechanisms of neural repair after stroke: making waves. Ann Neurol. 2006;59:735-742.
Carmichael S.T., Archibeque I., Luke L., et al. Growth-associated gene expression after stroke: evidence for a growth-promoting region in peri-infarct cortex. Exp Neurol. 2005;193:291-311.
Chang B.S., Lowenstein D.H. Practice parameter: Antiepileptic drug prophylaxis in severe traumatic brain injury: Report of the Quality Standards Subcommittee of the American Academy of Neurology. Neurology. 2003;60(1):10-16.
Chiaretti A., De Benedictis R., Polidori G., et al. Early post-traumatic seizures in children with head injury. Childs Nerv Syst. 2000;16(12):862-866.
Chicoine M.R., Park T.S., Voger G.P., et al. Predictors of ability to walk after selective dorsal rhizotomy in children with cerebral palsy. Neurosurgery. 1996;38:711-714.
Chorazy A.J.L., Crumrine P.K., Hanchett J.M., et al. Rehabilitation medical management. In: Ylvisaker M., editor. Traumatic brain injury rehabilitation: children and adolescents. Boston: Butterworth-Heinemann; 1998:27-39.
Cochran E.B., Phelps S.J., Tolley E.A., et al. Prevalence of, and risk factors for, upper gastrointestinal tract bleeding in critically ill pediatric patients. Crit Care Med. 1992;20(11):1519-1523.
Connor D.F., Steingard R.J. A clinical approach to the pharmacotherapy of aggression in children and adolescents. Ann N Y Acad Sci. 1996;794:290-307.
Crowner B.E., Brunstrom J.E., Racette B.A. Iatrogenic botulism due to therapeutic botulinum toxin a injection in a pediatric patient. Clin Neuropharmacol. 2007;30(5):310-313.
Dall J.T., Harmon R.L., Quinn C.M. Use of clonidine for treatment of spasticity arising from various forms of brain injury: a case series. Brain Inj. 1996;10:453-458.
de Bode S., Firestine A., Mathern G.W., et al. Residual motor control and cortical representation of function following hemispherectomy: effects of etiology. J Child Neurol. 2005;2:64-75.
Delgado M.R., Hirtz D., Aisen M., et al. Practice Parameter: Pharmacological Treatment of Spasticity in Children and Adolescents with Cerebral Palsy (an evidence-based review). Report of the Quality Standards Subcommittee of the American Academy of Neurology and the Practice Committee of the Child Neurology Society. Neurology. 2010. in press
Deluca S.C., Echols K., Law C.R., et al. Intensive pediatric constraint induced therapy for children with cerebral palsy: Randomized, controlled, crossover trial. J Child Neurol. 2006;21:931-938.
Dombovy M.L. Traumatic brain injury. In: Lazar R.B., editor. Principles of neurologic rehabilitation. New York: McGraw-Hill; 1998:79-103.
Duffau H. Brain plasticity: from pathophysiological mechanisms to therapeutic applications. J Clin Neurosci. 2006;13(9):885-897.
Edgar T. Clinical utility of botulinum toxin in the treatment of cerebral palsy: comprehensive review. J Child Neurol. 2001;16(1):37-46.
Einaudi S., Bondone C. The effects of head trauma on hypothalamic-pituitary function in children and adolescents. Curr Opin Pediatr. 2007;19(4):465-470.
Emanuelson I., Uvebrant P. Occurrence of epilepsy during the first 10 years after traumatic brain injury acquired in childhood up to the age of 18 years in the south western Swedish population-based series. Brain Inj. 2009;23(7):612-616.
Engsberg J.R., Ross S.A., Collins D.R., et al. Effect of selective dorsal rhizotomy in the treatment of children with cerebral palsy. J Neurosurg. 2006;105(1 Suppl):8-15.
Engsberg J.R., Ross S.A., Park T.S. Changes in ankle spasticity and strength following selective dorsal rhizotomy and physical therapy for spastic cerebral palsy. J Neurosurg. 1999;91(5):727-732.
Fawcett J.W., Rosser A.E., Dunnett S.B. Anatomical plasticity. In: Fawcett J.W., Rosser A.E., Dunnett S.B., editors. Brain damage, brain repair. Oxford: Oxford University Press; 2001:171-195.
Fehlings D., Rang M., Glazier J., et al. An evaluation of botulinum-A toxin injections to improve upper extremity function in children with hemiplegic cerebral palsy. J Pediatr. 2000;137:331-337.
Feldman P.A. Upper Extremity Casting and Splinting. In: Glenn M.B., Whyte J., editors. The Practical Management of Spasticity in Children and Adults. Philadelphia: Lea & Febiger; 1990:149-166.
Feldman Z., Contant C.V., Pahwa R., et al. The relationship between hormonal mediators and systemic hypermetabolism after severe head injury. J Trauma. 1993;34:806-816.
Finger S., Almli C.R. Brain damage and neuroplasticity: mechanisms of recovery or development. Brain Res Rev. 1985;10:177-186.
Francisco G.E., Walker W.C., Zasler N.D., et al. Pharmacological management of neurobehavioural sequelae of traumatic brain injury: a survey of current physiatric practice. Brain Inj. 2007;21(10):1007-1014.
Giebler K.B. Physical Modalities. In: Glenn M.B., Whyte J., editors. The Practical Management of Spasticity in Children and Adults. Philadelphia: Lea & Febiger; 1990:118-148.
Gilles E.E., Nelson M.D.Jr. Cerebral complications of non-accidental head injury in childhood. Pediatr Neurol. 1998;19:119-128.
Gilmartin R., Bruce D., Abbott R., et al. Intrathecal baclofen for the management of spastic cerebral palsy: Multicenter trial. J Child Neurol. 2000;15:71-77.
Gordon A.M., Charles J., Wolf S.L. Efficacy of constraint-induced movement therapy on involved upper-extremity use in children with hemiplegic cerebral palsy in not age dependent. Pediatrics. 2006;117:363-373.
Gracies J.M., Nance P., Elovic E., et al. Traditional pharmacological treatments for spasticity part II: general and regional treatments. Muscle Nerve. 1997;20:S92-S120.
Graham D.I. Pathophysiological aspects of injury and mechanisms of recovery. In: Rosenthal M., Griffith E.R., Kreutzer J.S., et al, editors. Rehabilitation of the adult and child with traumatic brain injury. ed 3. Philadelphia: FA Davis Company; 1999:19-41.
Hahn Y.S., Fuchs S., Flannery A.M., et al. Factors influencing posttraumatic seizures in children. Neurosurgery. 1988;22(5):864-867.
Hallenborg S.C. Positioning. In: Glenn M.B., Whyte J., editors. The practical management of spasticity in children and adults. Philadelphia: Lea & Febiger; 1990:91-117.
Haslam R.H., Walcher J.R., Leitman P.S., et al. Dantrolene sodium in children with spasticity. Arch Phys Med Rehabil. 1974;55:384-388.
Hertz-Pannier L., Chiron C., Jambaque I., et al. Late plasticity for language in a child’s non-dominant hemisphere: pre- and post-surgery fMRI study. Brain. 2002;125:361-372.
Hovda D.A., Lee S.M., Smith M.L., et al. The neurochemical and metabolic cascade following brain injury: moving from animal models to man. J Neurotrauma. 1995;12:903-906.
Howell K., Selber P., Graham H.K., et al. Botulinum neurotoxin A: an unusual systemic effect. J Paediatr Child Health. 2007;43(6):499-501.
Huttenlocher P.R. Morphometric study of human cerebral cortex development. Neuropsychologia. 1990;28:517-527.
Huttenlocher P.R., Dabholkar. Regional differences in synaptogenesis in human cerebral cortex. J Comp Neurol. 1997;387:167-178.
Illig K.R., Danilov Y.P., Ahmad A., et al. Funtional plasticity in extra striate visual cortex following neonatal visual cortex damage and manocular enucleation. Brain Res. 2000;882:241-250.
Jenkins W.M., Merzenich M.M. Reorganization of neocortical representations after brain injury: a neurophysiological model of the bases of recovery from stroke. Prog Brain Res. 1987;71:249-266.
Johnston M.V. Plasticity in the developing brain: implications for rehabilitation. Dev Disabil Res Rev. 2009;15(2):94-101.
Joynt R.L., Leonard J.A. Dantrolene sodium suspension in treatment of spastic cerebral palsy. Dev Med Child Neurol. 1980;22:755-767.
Katz R.T. Management of spasticity. Am J Phys Med Rehabil. 1988;67:108-116.
Kennedy C.R., Freeman J.M. Post-traumatic seizures and post-traumatic epilepsy in children. J Head Trauma Rehabil. 1986;1(4):66-73.
Kluger G., Kochs A., Holthausen H. Heterotopic ossification in childhood and adolescence. J Child Neurol. 2000;15(6):406-413.
Krach L.E., Kriel R.L. Traumatic brain injury. In: Molnar G.E., Alexander M.A., editors. Pediatric rehabilitation. ed 3. Philadelphia: Hanley & Belfus; 1999:245-268.
Krach L.E., Kriel R.L., Morris W.F., et al. Central autonomic dysfunction following acquired brain injury in children. J Neurol Rehabil. 1997;11:41-45.
Levin H., Hanten G., Max J., et al. Symptoms of attention-deficit/hyperactivity disorder following traumatic brain injury in children. J Dev Behav Pediatr. 2007;28(2):108-118.
Levy M., Berson A., Cook T., et al. Treatment of agitation following traumatic brain injury: a review of the literature. NeuroRehabilitation. 2005;20(4):279-306.
Lieber R.L., Steinman S., Barash I.A., et al. Structural and functional changes in spastic skeletal muscle. Muscle Nerve. 2004;29(5):615-627.
Lin J.J., Lin K.L., Hsia S.H., et al. Combined central diabetes insipidus and cerebral salt wasting syndrome in children. Pediatr Neurol. 2009;40(2):84-87.
Logemann J.A., Ylvisaker M. Therapy for feeding and swallowing disorders after traumatic brain injury. In: Ylvisaker M., editor. Traumatic brain injury rehabilitation: children and adolescents. Boston: Butterworth-Heinemann; 1998:85-99.
McLaughlin J.F., Bjornson K.F., Astley S.J., et al. Selective dorsal rhizotomy: efficacy and safety in an investigator-masked randomized clinical trial. Dev Med Child Neurol. 1998;40:220-232.
McLaughlin J., Bjornson K., Temkin N., et al. Selective dorsal rhizotomy: meta-analysis of three randomized controlled trials. Dev Med Child Neurol. 2002;44(1):17-25.
McLean D.E., Kaitz E.S., Keenan C.J., et al. Medical and surgical complications of pediatric brain injury. J Head Trauma Rehabil. 1995;10(5):1-12.
Meythaler J.M., Roper J.F., Brunner R.C. Cyproheptadine for intrathecal baclofen withdrawal. Arch Phys Med Rehabil. 2003;84(5):638-642.
Meythaler J.M., Tuel S.M., Cross L.L. Successful treatment of immobilization hypercalcemia using calcitonin and etidronate. Arch Phys Med Rehabil. 1993;74(3):316-319.
Michaud L.J., Duhaime A.C., Batshaw M.L. Traumatic brain injury in children. Pediatr Clin North Am. 1993;40(3):553-565.
Milanov I., Georgiev D. Mechanisms of tizanidine action on spasticity. Acta Neurol Scan. 1994;89:274-279.
Milla P.J., Jackson A.D.M. A controlled trial of baclofen in children with cerebral palsy. J Int Med Res. 1977;5:398-404.
Mital M., Garber J., Stinson J. Ectopic bone formation in children and adolescents with head injuries: its management. J Pediatr Orthop. 1987;7(1):83-90.
Mueller M.E., Gruenthal M., Olson W.L., et al. Gabapentin for relief of upper motor neuron symptoms in multiple sclerosis. Arch Phys Med Rehabil. 1997;78(5):521-524.
Murphy A.M., Milo-Manson G., Best A., et al. Impact of modafinil on spasticity reduction and quality of life in children with CP. Dev Med Child Neurol. 2008;50(7):510-514.
Newman C.J., Kennedy A., Walsh M., et al. A pilot study of delayed versus immediate serial casting after botulinum toxin injection for partially reducible spastic equinus. J Pediatr Orthop. 2007;27:882-885.
Noetzel M.J. Acute pediatric neurorehabilitation. In: Winn H.R., editor. Youman’s neurological surgery. ed 5. Philadelphia: Elsevier Science; 2003:3783-3791.
Noetzel M.J., Miller G. Traumatic brain injury as a cause of cerebral palsy. In: Clark G.D., Miller G., editors. The cerebral palsies: causes, consequences and management. Newton MA: Butterworth Heinmann; 1998:185-207.
Nogen A.G. Effect of dantrolene sodium on the incidence of seizures in children with spasticity. Childs Brain. 1979;5:420-425.
O’Shanick G. Pharmacologic intervention. In: Ylvisaker M., editor. Traumatic brain injury rehabilitation: children and adolescents. Boston: Butterworth-Heinemann; 1998:53-59.
Park T.S., Gaffney P.E., Kaufman B.A., et al. Selective lumbosacral dorsal rhizotomy immediately caudal to the conus medullaris for cerebral palsy spasticity. Neurosurgery. 1993;33(5):929-933.
Phillips R., Ott L., Young B., et al. Nutritional support and measured energy expenditure of the child and adolescent with head injury. J Neurosurg. 1987;67(6):846-851.
Pidcock F.S., Garcia T., Trovato M.K., et al. Pediatric constraint-induced movement therapy: a promising intervention for childhood hemiparesis. Top Stroke Rehabil. 2009;16(5):339-345.
Ploumis A., Ponnappan R.K., Maltenfort M.G., et al. Thromboprophylaxis in patients with acute spinal injuries: an evidence-based analysis. J Bone Joint Surg Am. 2009;91(11):2568-2576.
Pranzatelli M.R. Oral pharmacotherapy for the movement disorders of cerebral palsy. J Child Neurol. 1996;11:S13-S22.
Pranzatelli M.R., Pavlakis S.G., Gould R.G., et al. Hypothalamic-midbrain dysregulation syndrome: hypertension, hyperthermia, hyperventilation, and decerebration. J Child Neurol. 1991;6:115-122.
Price G.W., Wilkin G.P., Turnbull M.J., et al. Are baclofen-sensitive GABAB receptors present on primary afferent terminals of the spinal cord? Nature. 1984;307(5946):71-74.
Priebe M.M., Sherwood A.M., Graves D.E., et al. Effectiveness of gabapentin in controlling spasticity: a quantitative study. Spinal Cord. 1997;35(3):171-175.
Renshaw T.S., Green N.E., Griffin P.P., et al. Cerebral palsy: orthopaedic management. Instr Course Lect. 1996;45:475-490.
Rice S.A., Blackman J.A., Braun S., et al. Rehabilitation of children with traumatic brain injury: descriptive analysis of a nationwide sample using the WeeFIM. Arch Phys Med Rehabil. 2005;86(4):834-836.
Rose J., Gamble J.G., Burgos A., et al. Energy expenditure index of walking for normal children and for children with cerebral palsy. Dev Med Child Neurol. 1990;32(4):333-340.
Russo R.N., O’Flaherty S. Bromocriptine for the management of autonomic dysfunction after severe traumatic brain injury. J Paediatr Child Health. 2000;36(3):283-285.
Sadato N., Pascaul-Leone A., Grafman J., et al. Activation of primary visual cortex by Braille reading in blind subjects. Nature. 1996;380:526-528.
Sanger T.D., Delgado M.R., Gaebler-Spira D., et al. Task Force on Childhood Motor Disorders. Classification and definition of disorders causing hypertonia in childhood. Pediatrics. 2003;111(1):e89-e97.
Saxena S., Caroni P. Mechanisms of axon degeneration: from development to disease. Prog Neurobiol. 2007;83(3):174-191.
Selzer M.E. Neurological rehabilitation. Ann Neurol. 1992;32:695-699.
Shehab D., Elgazzar A., Collier B. Heterotopic ossification. J Nucl Med. 2002;43(3):346-353.
Shreiber D.I., Bain A.C., Ross D.T., et al. Experimental investigation of cerebral contusion: histopathological and immunohistochemical evaluation of dynamic cortical deformation. J Neuropathol Exp Neurol. 1999;58(2):153-164.
Sobus K.M., Alexander M.A., Harcke H.T. Undetected musculoskeletal trauma in children with traumatic brain injury or spinal cord injury. Arch Phys Med Rehabil. 1993;74:902-904.
Steenbeek D., Meester-Delver A., Becher J.G., et al. The effect of botulinum toxin type A treatment of the lower extremity on the level of functional abilities in children with cerebral palsy: evaluation with goal attainment scaling. Clin Rehabil. 2005;19:274-282.
Suz P., Vavilala M.S., Souter M., et al. Clinical features of fever associated with poor outcome in severe pediatric traumatic brain injury. J Neurosurg Anesthesiol. 2006;18(1):5-10.
Taub E., Ramey S.L., DeLuca S., et al. Efficacy of constraint-induced movement therapy for children with cerebral palsy with asymmetric motor impairment. Pediatrics. 2004;113:305-312.
Taub E., Uswatte G., Elbert T. New treatments in neurorehabilitation founded on basic research. Nat Rev Neurosci. 2002;3:228-236.
Taylor D.A. Neurorehabilitation in child neurology. J Child Neurol. 1991;6:97-100.
Thomas-Stonell N., Johnson P., Rumney P., et al. An evaluation of the responsiveness of a comprehensive set of outcome measures for children and adolescents with traumatic brain injuries. Pediatr Rehabil. 2006;9(1):14-23.
Tilton A. Management of spasticity in children with cerebral palsy. Semin Pediatr Neurol. 2009;16:82-89.
Tilton A.H. Injectable neuromuscular blockade in the treatment of spasticity and movement disorders. J Child Neurol. 2003;18:S50-S66.
Turner M.S. Early use of intrathecal baclofen in brain injury in pediatric patients. Acta Neurochir Suppl. 2003;87:81-83.
Van Schaeybroeck P., Nuttin B., Lagae L., et al. Intrathecal baclofen for intractable cerebral spasticity: A prospective placebo-controlled, double-blind study. Neurosurgery. 2000;46:603-609.
Villablanca J.R., Hovda D.A. Developmental neuroplasticity in a model of cerebral hemispherectomy and stroke. Neurosci. 2000;95:625-637.
Ward C., McIntosh S. The rehabilitation process: a neurological perspective. In: Greenwood R., Barnes M.P., McMillan T.M., et al, editors. Neurological rehabilitation. Edinburgh: Churchill Livingston; 2003:15-29.
Waxman S.G. Functional recovery in diseases of the nervous system. Adv Neurol. 1988;47:1-7.
Whyte J., Hart T., Vaccaro M., et al. Effects of methylphenidate on attention deficits after traumatic brain injury: a multidimensional, randomized, controlled trial. Am J Phys Med Rehabil. 2004;83(6):401-420.
Whyte J., Robinson K.M. Pharmacologic management. In: Glenn M.B., Whyte J., editors. The practical management of spasticity in children and adults. Philadelphia: Lea & Febiger; 1990:201-226.
Williams S.E. Amantadine treatment following traumatic brain injury in children. Brain Inj. 2007;21(9):885-889.
World Health Organization. The international classification of impairments, activities and participation (ICIDH-2). WHO; 1997.
Wright F., Sheil E.M., Drake J.M., et al. Evaluation of selective dorsal rhizotomy for the reduction of spasticity in cerebral palsy: a randomized control study. Dev Med Child Neurol. 1998;40:239-247.
Yarkony G.M. Spinal cord injury rehabilitation. In: Lazar R.B., editor. Principles of neurologic rehabilitation. New York: McGraw-Hill; 1997:121-134.
Ylivsaker M., Chorazy A.J.L., Feeney T.J., et al. Traumatic brain injury in children and adolescents: assessment and rehabilitation. In: Rosenthal M., Griffith E.R., Kreutzer J.S., et al, editors. Rehabilitation of the adult and child with traumatic brain injury. ed 3. Philadelphia: FA Davis Company; 1999:356-392.
Zhang R.L., Zhang Z.G., Chopp M. Neurogenesis in the adult ischemic brain: generation, migration, survival, and restorative therapy. Neuroscientist. 2005;11:408-416.
Zidek K., Srinuvasan R. Rehabilitation of a child with spinal cord injury. Semin Pediatr Neurol. 2003;10(2):140-150.