Pediatric breathing circuits
Mapleson circuits
By the 1950s, several types of semiclosed circuits were used to deliver anesthetic gases. Semiclosed circuits under optimal conditions prevent rebreathing of alveolar gases. In 1954, the physicist William W. Mapleson analyzed five of these circuits and proposed optimal conditions that would prevent rebreathing. The efficiency of a nonrebreather is determined by the amount of fresh-gas flow, as well as by the positions of the inflow of fresh gas, the expiratory valve, and the reservoir bag. Mapleson labeled these circuits A, B, C, D, and E (Figure 193-1); subsequently, these circuits have been referred to as the Mapleson circuits, and Mapleson’s theoretical analyses have been verified empirically by others.
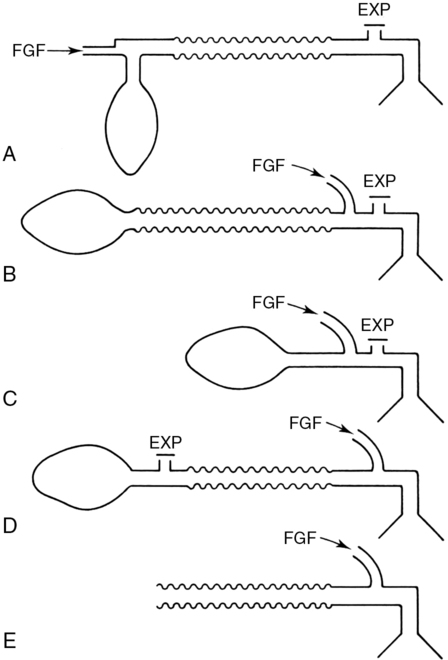
Mapleson A circuit
Immediately before the inspiratory phase of spontaneous ventilation occurs, continuous fresh gas flows into the reservoir bag and the circuit (Figure 193-2). As the patient inhales, the reservoir bag begins to empty. The lower the fresh-gas flow, or the higher the tidal volume, the emptier the reservoir bag becomes. During the expiratory phase, the reservoir bag completely fills with fresh gas, and, when the fresh-gas flow exceeds 70% of minute ventilation, enough pressure develops to vent alveolar and fresh gas through the APL valve. At the last stage of the expiratory phase, a pause occurs before the initiation of the next cycle. During the expiratory pause, fresh-gas flow further drives alveolar gas through the APL valve and virtually eliminates rebreathing.