Chapter 97 Pathogenesis of Proliferative Vitreoretinopathy
Introduction
PVR is reported to have an incidence of 5–10% of all rhegmatogenous retinal detachments (RRD),1 and it is believed to be the most common cause of ultimate failure of a surgery for RRD. A high rate of PVR has also been reported following posterior segment procedures or disorders such as giant retinal tears, macular relocation surgery, endoresection of tumors and chip implantation. In human immunodeficiency, virus-infected patients PVR has been developed in 29% of cytomegalovirus retinitis-related retinal detachment.2 In children, postoperative PVR occurs in higher incidence and is characterized by a rapid and aggressive development.3 PVR is also a common complication following a variety of ocular injuries with the highest frequency after perforating and penetrating wounds, in which the incidence is 10–45%.
Soon after the development of vitrectomy, PVR became a specific indication for vitreous surgery. The changing understanding of PVR pathogenesis is reflected in the different classification systems. In the beginning, it was assumed to be primarily due to changes in the vitreous gel (“massive vitreous retraction”, “massive preretinal retraction”). Then the involvement of cells was recognized, and the condition was re-termed “massive periretinal proliferation.”4 Later, a distinct biomicroscopic classification was developed in an attempt to categorize the severity of the disease (Retina Society Classification 1983). A major problem of the latter system was that it did not reflect prognosis and surgical difficulty. The Cologne classification5 and the Silicone Oil Study classification6 took these aspects into account by separating anterior and posterior PVR. The updated Retina Society classification is a compromise, which describes severity (A, B, C), localization, updated grade, and updated contraction type.7 However, this classification still does not consider many aspects of the disorder. Therefore, a classification that provides additional relevant clinical information, such as the evolutionary stages of the disease (biologic activity) and the degree of surgical difficulty is desirable.8
Clinically, early stages of PVR are characterized by an increased reflectance and a cellophane appearance of the inner retinal surface. Additionally, tortuosity of both small and larger vessels is regularly observed. The pathological hallmarks of the advanced PVR include periretinal membrane formation, causing development of surface wrinkling and single or multifocal star-folds (Fig. 97.1). In the final stages, multidirectional tractional forces produced by posterior and/or anterior PVR form a narrow or closed funnel of the detached retina.9
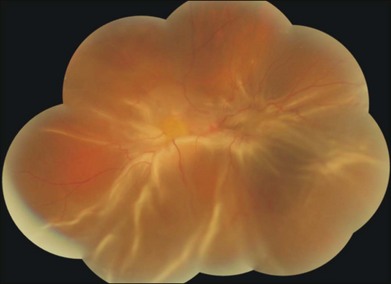
Fig. 97.1 Human proliferative vitreoretinopathy showing typical surface wrinkling and multifocal star-folds.
A retinal break is a prerequisite for the development of PVR. Almost all risk factors for the PVR are associated with intravitreal dispersion of retinal pigment epithelial (RPE) cells or the breakdown of the blood–retinal barrier (BRB).10,11 The size of breaks, the extent of detachment, the presence of preoperative inflammation or low-grade PVR, and iatrogenic complications are important factors in the pathogenesis of severe PVR after the surgery for retinal detachment.12,13
Several investigators have underlined the parallels between PVR and the general wound-healing process.14,15 PVR develops in a sequence of three overlapping phases: inflammation, cellular proliferation, and extracellular matrix remodeling. The time course of PVR development is poorly investigated. Data which were accumulated from clinical analysis could reveal that on average it takes 4–8 weeks for PVR development after surgery.8,16 Detailed PVR chronobiology was evaluated in the experimental animal model of PVR induced by macrophages. The inflammation phase is initiated immediately in response to macrophage injection. Cellular proliferation can be detected as early as days 4 ± 7 and peaks on days 10 ± 14. The scarring-induced retinal detachment occurs during the 2nd and 3rd weeks after macrophage injection.17
Cellular basis of PVR
Composition of membranes
The composition of the membranes changes with time, from early cellular to late paucicellular and rather fibrotic membranes.18 In fibroproliferative membranes, glial cells, RPE cells, and fibrocytes transdifferentiate into contractile myofibrocytes,19 which generate tractional forces through the expression of the contractile protein α-smooth-muscle actin. Fibroblastic transdifferentiation of cells causes a reduction in cell-specific proteins such as glial fibrillary acidic protein (GFAP; glial cells) and cytokeratins (RPE cells), while proteins involved in motility and proliferation such as α-smooth-muscle actin (which is normally not expressed by the cells) are upregulated.19–21 This cellular transdifferentiation may be the reason for the paradox that relatively few glial and pigment epithelial cells can be detected in periretinal membranes with the commonly used immunocytochemical markers.
RPE cells
EMT is an important process during normal development in which epithelial cells transform to mesenchymal cells, and show alterations in polarized organization, intercellular adhesion and become migratory and invasive. The critical role of EMT has also been recognized in numerous pathological conditions including tumor progression and fibrosis. EMT appears to play a critical role in PVR. During retinal detachment, RPE cells gain access through retinal breaks into the vitreous cavity, adhere to the detached retina, and undergo EMT to a fibrocyte phenotype. Studies on EMT show that various factors such as cytokines, modified growth factor signaling, loss of normal matrix adhesion, and changes in cell–cell adhesion profile may initiate this process. Recent studies suggest that disruption and loss of cell–cell contacts initiate EMT and proliferation of RPE cells.22,23 During EMT, RPE cells lose their epithelial phenotypes and acquire mesenchymal, fibroblast-like properties. Characteristic features of this process are the upregulation of α-smooth-muscle actin and vimentin, the loss of the zona occludens protein ZO-1, the reduced intercellular adhesion and the increased motility and enhanced migration24 which is supported by the enhanced matrix metalloproteinases (MMPs) activity.
RPE cell migration is a complex process that includes changes in cell attachment, spreading, and cytoskeletal reorganization, and it is regulated by cell matrix, matrix-dependent enzymes, cytokines, and growth factors. RPE cell migration is also mediated by cell membrane-associated signaling.25
Glial cells
Whereas the role of RPE cells has been widely discussed in the literature over the last 30 years, the impact of glial cells on the pathogenesis of PVR was frequently overlooked. Glial cells and, in particular, Müller cells, play a central role in retinal physiology. They support the neuronal activity, the integrity of the BRB, and maintain the ionic and osmotic homeostasis. Virtually all retinal diseases are associated with reactive gliosis, which is characterized by cellular hypertrophy and upregulation of the intermediate filaments vimentin and GFAP. Moreover, Müller cell gliosis is associated with proliferation and PVR is a key example of massive and long-lasting cellular proliferation.26 Reactive gliosis is observed within minutes after experimental retinal detachment and proceeds so long as the retina remains detached.27 In the course of PVR, Müller cells proliferate and migrate out of the retina where they are a constant part of fibrocellular membranes. Fibroproliferative membranes are focally connected to the retina via hypertrophied processes of Müller cells, rising from the retinal tissue into the membranes.1,28,29
The proliferation of Müller cells is accompanied by alterations in their membrane conductance. Müller cells of patients with PVR, and those from animal models of PVR, display a severe downregulation of the potassium conductance mediated by inwardly rectifying potassium (Kir) channels.30–33 The downregulation of functional Kir channels causes a depolarization of the cells which is a prerequisite for the reentry of the cells into the proliferation cycle.34 Moreover, the impaired retinal potassium homeostasis may favor neuronal hyperexcitation and glutamate toxicity. Therefore, the depolarization of Müller cells may contribute to an impairment of the regular glial–neuronal interactions in the retina and, thus, to the neuronal degeneration observed in PVR.35 Furthermore, activated Müller cells may act as modulators of immune and inflammatory processes, for example by producing proinflammatory cytokines.36 Reactive gliosis is a limiting factor in the recovery of vision after retinal reattachment.29 In the healthy retina, Müller cells serve as soft, compliant embedding for neurons and also as a soft substrate required for neurite growth and facilitating neuronal plasticity.37 The hypertrophied and proliferating glial cells fill the spaces left by dying neurons and degenerated axons and generate so-called glial scars. Reactive gliosis is associated with an increased stiffness of Müller cells.38 Such rigid glial scars may constitute a mechanical obstacle for regenerative axon growth. Therefore, attempts to reduce Müller cell gliosis may be a promising tool to inhibit retinal degeneration and to support neuroregeneration after retinal reattachment.29,35
Blood-borne cells
Inflammation is an important step in the pathogenesis of PVR and is associated with influx of blood-derived cells into the retinal tissue and vitreous. Blood-borne cells such as macrophages and fibrocytes are frequently found in PVR membranes. Macrophage-like cells might be also derived from RPE cells which have undergone EMT. Circulating fibrocytes and macrophages may function as precursors of myofibroblasts in PVR membranes and may contribute to the fibrocellular membranes directly.39,40 Hyalocytes (cells in the cortical vitreous) belong to the monocyte/macrophage lineage and play a significant role in the synthesis of ECM, modulation of immune reaction, and modulation of inflammation. The strong contractile properties of hyalocytes suggest also a critical role in the genesis of PVR.41
Stimulation of cellular proliferation and migration
There is a great deal of evidence supporting the idea that soluble factors including growth factors and cytokines play a central role in the pathogenesis of PVR, inducing key cellular responses such as chemotaxis, proliferation, migration, and extracellular matrix remodeling.42 Growth factors are proteins that bind to receptors on the cell surface, with the primary result of activating cellular proliferation and/or differentiation. Cytokines specifically denote signaling proteins that are used extensively in cellular communication. They often exhibit growth factor activity and can exert autocrine, paracrine and endocrine effects as do the hormones. Interleukins are cytokines specifically directed to leucocytes. A number of soluble factors contribute to PVR, but the evidence accumulated so far is greatest for the factors below.
Blood components
A breakdown of the blood–retinal barrier is a characteristic and essential event in the pathogenesis of PVR. Serum, which enters the retina and vitreous seems to play an important role in the transmission of mitogenic signals to both Müller cells and RPE cells. Thrombin, a serum component, stimulates the proliferation of retinal glial cells and RPE cells.43,44 Fibronectin stimulates the migration of glial cells43 and plays an important role in ECM remodeling (see below). The vitreous of experimental PVR animals and retinal surgery patients contains the serum-derived protease plasmin. It is responsible for the generation of active platelet-derived growth factor-C (PDGF-C), which is the major PDGF isoform involved in the development of PVR45 (see below).
Platelet-derived growth factor (PDGF)
PDGF, a wound-healing cytokine, seems to play a critical role in the development of PVR. Both PDGF and PDGF receptor (PDGFR) are present in PVR membranes.46 Vitreal PDGF could be detected in eight out of nine PVR patients, but only in one out of 16 patients with other vitreoretinal diseases.45 RPE and retinal glial cells produce PDGFs and possess their receptors.46 PDGF is a potent chemoattractant and mitogen for many cell types, including RPE and glial cells.47,48
Among the four PDGF family members, PDGF-C was the predominant isoform in PVR specimens.45 Plasmin is suggested to be the major PDGF-C processing protease.49 Analysis of epiretinal PVR membranes indicated that a greater percentage of the PDGFRα receptors is activated as compared to PDGFRβ and that both retinal pigment epithelial and glial cells express activated PDGFRα receptors.50 Abundant binding of PDGF-C to PDGFRα explains why PDGFRα activation is more important in the pathogenesis of PVR than PDGFRβ activation.45 In a rabbit model of PVR, delivery of retrovirus containing a dominant negative PDGFRα suppresses the development of retinal detachment.51 Furthermore, oral administration of tyrosine kinase inhibitors, which block signaling through PDGF receptors, reduce PDGF-induced epiretinal membrane formation and retinal detachment in transgenic mice.52 It has been shown recently that PDGFRα can also be activated by other growth factors besides PDGF.53 This indirect activation of PDGFRα involves the formation of intracellular reactive oxygen species (ROS). Initial studies have shown that vitreal injection of the antioxidant N-acetyl cysteine prevents the development of experimental PVR.54
Transforming growth factor-β
TGF-β is implicated in the tissue contraction in different fibrous diseases including proliferative eye diseases such as proliferative diabetic retinopathy and PVR.55 The vitreous of PVR patients contains high levels of TGF-β.56 TGF-β was also localized to subretinal strands of PVR patients.57 Müller cells are one source of TGF-β in the retina.58 Experimental retinal detachment increases the expression of TGF-β and TGF receptor II in Müller cells and in hypertrophied Müller cells processes that contribute to the formation of periretinal membranes.59 TGF-β-treated RPE cells differentiate along a myofibroblast pathway.24 Furthermore, gene transfer of a soluble TGF-β type II receptor (which traps TGF-β) suppresses the progression of experimental PVR.60 These experimental and clinical studies indicate that inhibition of TGF-β signaling could be a therapeutic approach to prevent the progression of PVR. Intravitreal injection of the cicatricial contraction inhibitor simvastatin could prevent PVR progression in a dose-dependent manner.61 TGF-β likely mediates cicatricial contraction via activation of the Rho-kinase (ROCK) pathway; this pathway is also activated by various other growth factors implicated in cell adhesion, proliferation and migration. It makes it an interesting therapeutic target for treating PVR.62,63
Monocyte chemotactic protein-1 (MCP1)
The chemokine MCP-1 has a role during the initial stage of PVR. In experimental retinal detachment, the expression of MCP-1 increases within one hour, and the MCP-1 protein level increases within six hours of detachment.64 MCP-1 stimulates the migration of RPE cells; this effect is inhibited by dexamethasone.65 MCP-1 was detected in a substantial percent of vitreous samples of patients with PVR.66