Nutrition Alterations and Management
Assessing Nutritional Status
In the United States, The Joint Commission mandates nutrition screening be conducted on every patient within 24 hours of admission to an acute care center.1 A brief questionnaire to be completed by the patient or significant other, the nursing admission form, or the physician’s admission note usually provides enough information to determine whether the patient is at nutritional risk (Box 8-1). Any patient judged to be nutritionally at risk needs a more thorough nutrition assessment. The nutrition assessment process is continuous, with reassessments as a part of the overall nutrition care plan, as shown in Figure 8-1.2
Nutrition assessment involves collection of four types of information: 1) anthropometric measurements; 2) biochemical (laboratory) data; 3) clinical signs (physical examination); and 4) diet and pertinent health history. This information provides a basis for 1) identifying patients who are malnourished or at risk of malnutrition; 2) determining the nutritional needs of individual patients; and 3) selecting the most appropriate methods of nutrition support for patients with or at risk of developing nutritional deficits. Nutrition support is the provision of specially formulated or delivered oral, enteral, or parenteral nutrients to maintain or restore optimal nutrition status.3 The nutrition assessment can be performed by or under the supervision of a registered dietitian or by a nutrition care specialist (e.g., nurse with specialized expertise in nutrition). Figure 8-2 shows the route of administration of specialized nutrition support.
Anthropometric Measurements
< ?xml:namespace prefix = "mml" />

Weight is measured in kilograms and height in meters. BMI values are independent of age and gender and are used for assessing health risk. BMI can be classified as shown in Table 8-1.4,5 Evidence that the associations between BMI, percent of body fat, and body fat distribution differ across populations suggests the possible need for developing different BMI cut-off values for different ethnic groups.6 For example, alternate BMI classification cut-off values exist for Asians, who are at risk for obesity-related co-morbidities at lower BMI.6
TABLE 8-1
CLASSIFICATION | BMI (kg/m2) |
Underweight | <18.5 |
Normal | 18.5-24.99 |
Overweight: | ≥25 |
Pre-obese | 25-29.99 |
Obese class I | 30-34.99 |
Obese class II | 35-39.99 |
Obese class III | ≥40 |
From World Health Organization. Obesity: preventing and managing the global epidemic. Report of a WHO Consultation. WHO Technical Report Series 894. Geneva: World Health Organization; 2000.
It may be impossible to measure the height of some patients accurately. Total height can be estimated from arm span length or knee height.7,8 To measure knee height, bend the knee 90 degrees, and measure from the base of the heel to the anterior surface of the thigh.


In addition to height and weight data, other measurements such as arm muscle circumference, skin fold thickness, and body composition (proportion of fat and lean tissue, determined by bioelectric impedance or other methods) are sometimes performed, but these measurements are of limited use in assessing critically ill patients.9
Biochemical Data
A wide range of laboratory tests can provide information about nutritional status. Those most often used in the clinical setting are described in Table 8-2. No diagnostic tests for evaluation of nutrition are perfect, and care must be taken in interpreting the results of the tests.10
TABLE 8-2
COMMON BLOOD AND URINE TESTS USED IN NUTRITION ASSESSMENT
TEST | COMMENTS AND LIMITATIONS |
Serum Proteins | |
Albumin or prealbumin | Levels decrease with protein deficiency and in liver failure. Albumin levels are slow to change in response to malnutrition and repletion. Prealbumin levels fall in response to trauma and infection. |
Hematologic Values | |
Anemia | |
Normocytic (normal MCV, MCHC) | Common with protein deficiency |
Microcytic (decreased MCV, MCH, MCHC) | Indicative of iron deficiency (can be from blood loss) |
Macrocytic (increased MCV) | Common in folate and vitamin B12 deficiency |
Lymphocytopenia | Common in protein deficiency |
Clinical or Physical Manifestations
A thorough physical examination is an essential part of nutrition assessment. Box 8-2 lists some of the more common findings that may indicate an altered nutritional state. It is especially important for the nurse to check for signs of muscle wasting, loss of subcutaneous fat, skin or hair changes, and impairment of wound healing.
Diet and Health History
Information about dietary intake and significant variations in weight is a vital part of the history. Dietary intake can be evaluated in several ways, including a diet record, a 24-hour recall, and a diet history. The diet record, a listing of the type and amount of all foods and beverages consumed for some period (usually 3 days), is useful for evaluating the patient’s intake in the critical care setting if the adequacy of intake is questionable. However, such a record reveals little about the patient’s habitual intake before the illness or injury. The 24-hour recall of all food and beverage intake is easily and quickly performed, but it also may not reflect the patient’s usual intake and has limited usefulness. The diet history consists of a detailed interview about the patient’s usual intake, along with social, familial, cultural, economic, educational, and health-related factors that may affect intake. Although the diet history is time consuming to perform and may be too stressful for the acutely ill patient, it does provide a wealth of information about food habits over a prolonged period and a basis for planning individualized nutrition education if changes in eating habits are desirable. Other information to include in a nutrition history is listed in Box 8-3.
Determining Nutritional Needs
A variety of methods can be used in clinical practice to estimate caloric requirements. Indirect calorimetry, a method by which energy expenditure is calculated from oxygen consumption (Vo2) and carbon dioxide production (Vco2), is the most accurate method for determining caloric needs.11,12 Indirect calorimetry is useful in those patients suspected to have a high metabolic rate. This test can also analyze substrate use, which can be extrapolated from VO2 and VCO2 during a steady state of respiration. The respiratory quotient (RQ) is equal to the VCO2 divided by the Vo2. Fat, protein, and carbohydrates each have a unique RQ, thus RQ identifies which substrate is being preferentially metabolized and may provide target goals for calorie replacement.12 For example, the RQ for the metabolism of fats is about 0.7 while the RQ for the metabolism of carbohydrates is 1.0. A mixed fuel diet results in an RQ of approximately 0.8.12 The test can be performed on spontaneously breathing patients and on those who require mechanical ventilation. Some ventilators are constructed so that they can perform indirect calorimetry. However, for most patients, indirect calorimetry requires the use of a metabolic cart, which is not available in all institutions. To maintain accuracy and reliability of measurement, several testing criteria must be met.12 Information received from the metabolic cart is limited; measurements are conducted over a relatively brief period (often 20 to 30 minutes) and may not be representative of energy expenditure over the whole day.
Calorie and protein needs of patients are often estimated using formulas that provide allowances for increased nutrient use associated with injury and healing. Although indirect calorimetry is considered the most accurate method to determine energy expenditure, estimates using formulas have demonstrated reasonable accuracy.13–15 Commonly used formulas for critically ill patients can be found in Appendix B. Some rules of thumb are available to provide a rough estimate of caloric needs so that nurses and other caregivers can quickly determine if patients are being seriously overfed or underfed (Table 8-3).
TABLE 8-3
CATEGORY | DESCRIPTION | CALORIES/kg | CALORIES/lb |
Obese | More than 40% over ideal body weight or BMI >30 | 21 | 9.5 |
Sedentary | Relatively inactive individual without regular aerobic exercise; hospitalized patient without severe injury or sepsis | 25-30 | 11-13.5 |
Moderate activity or injury | Individual obtaining regular aerobic exercise plus routine activities; patient with trauma or sepsis | 30-35 | 13.5-16 |
Very active or severe injury | Manual laborer or athlete in very active training; patient with major burns or trauma | 40 | 18 |
The goal of nutrition assessment is to obtain the most accurate estimate of nutritional requirements. Underfeeding and overfeeding must be avoided during critical illness. Overfeeding results in excessive production of carbon dioxide, which can be a burden in the person with pulmonary compromise. Overfeeding increases fat stores, which can contribute to insulin resistance and hyperglycemia. Hyperglycemia increases the risk of postoperative infections in diabetic and nondiabetic individuals.16
Implications of Undernutrition for the Sick or Stressed Patient
As many as 40% of hospitalized patients are at risk for malnutrition.17–20 Although illness or injury is the major factor contributing to development of malnutrition, other possible contributing factors are lack of communication among the nurses, physicians, and dietitians responsible for the care of these patients; frequent diagnostic testing and procedures, which lead to interruption in feeding; medications and other therapies that cause anorexia, nausea, or vomiting and thereby interfere with food intake; insufficient monitoring of nutrient intake; and inadequate use of supplements, tube feedings, or parenteral nutrition to maintain the nutritional status of these patients.
Nutritional status tends to deteriorate during hospitalization unless appropriate nutrition support is started early and continually reassessed. Malnutrition in hospitalized patients is associated with a wide variety of adverse outcomes. Wound dehiscence, pressure ulcers, sepsis, infections, respiratory failure requiring ventilation, longer hospital stays, and death are more common among malnourished patients.21–23 Decline in nutritional status during hospitalization is associated with higher incidences of complications, increased mortality rates, increased length of stay, and higher hospital costs.
Nutrition Support
Nursing Management of Nutrition Support
Nutrition support is an important aspect of the care of critically ill patients. Maintenance of optimal nutritional status may prevent or reduce the complications associated with critical illness and promote positive clinical outcomes.3 Critical care nurses play a key role in the delivery of nutrition support and must work closely with dietitians and physicians in promoting the best possible outcomes for their patients.
Nutrition support is the provision of oral, enteral, or parenteral nutrients. It is an essential adjunct in the prevention and management of malnutrition in critically ill patients.3 The goal of nutrition support therapy is to provide enough support for body requirements, to minimize complications, and to promote rapid recovery. Critical care nurses must have a broad understanding of nutrition support, including the indications, prevention, and management of associated complications.
Oral Supplementation
1. Collaborate with the dietitian to choose appropriate products and allow the patient to participate in the selection process, if possible. Milk shakes and instant breakfast preparations are often more palatable and economical than commercial supplements. However, lactose intolerance is common among adults. Many disease processes (e.g., Crohn’s disease, radiation enteritis, human immunodeficiency virus [HIV] infection, severe gastroenteritis) can cause lactose intolerance. Individuals with this problem require commercial lactose-free supplements or milk treated with lactase enzyme.
2. Offer to serve commercial supplements well chilled or on ice, because this improves flavor.
3. Advise patients to sip formulas slowly, consuming no more than 240 mL over 30 to 45 minutes. These products contain easily digestible carbohydrates. If formulas are consumed too quickly, rapid hydrolysis of the carbohydrate in the duodenum can contribute to dumping syndrome, characterized by abdominal cramping, weakness, tachycardia, and diarrhea.
4. Record all supplement intake separately on the intake-and-output sheet so that it can be differentiated from intake of water and other liquids.
Enteral Nutrition
Enteral nutrition or tube feedings are used for patients who have at least some digestive and absorptive capability but are unable or unwilling to consume enough by mouth. When possible, the enteral route is the preferred method of feeding over total parenteral nutrition (TPN). The proposed advantages of enteral nutrition over TPN include lower cost, better maintenance of gut integrity, and decreased infection and hospital length of stay.3 A review of the literature comparing enteral nutrition and TPN indicates that enteral nutrition is less expensive than TPN and is associated with a lower risk of infection.3,24
The gastrointestinal (GI) tract plays an important role in maintaining immunologic defenses, which is why nutrition by the enteral route is thought to be more physiologically beneficial than TPN. Some of the barriers to infection in the GI tract include neutrophils; the normal acidic gastric pH; motility, which limits GI tract colonization by pathogenic bacteria; the normal gut microflora, which inhibit growth of or destroy some pathogenic organisms; rapid desquamation and regeneration of intestinal epithelial cells; the layer of mucus secreted by GI tract cells; and bile, which detoxifies endotoxin in the intestine and delivers immunoglobulin A (IgA) to the intestine. A second line of defense against invasion of intestinal bacteria is the gut-associated lymphoid tissue (GALT).25 The systemic immune defenses in the GI tract are stimulated by the presence of food within it. In animal models, resting the GI tract by providing TPN contributes to bacterial translocation, whereby bacteria normally found in the GI tract cross the intestinal barrier, are found in the regional mesenteric lymph nodes, and give rise to generalized sepsis. However, there is insufficient evidence in humans that TPN causes atrophy of the intestinal mucosa or that enteral nutrition prevents bacterial translocation.26,27
Patients who are experiencing severe stress that greatly increases their nutritional needs (caused by major surgery, burns, or trauma) often benefit from tube feedings. Table 8-4 lists different enteral formula types and the nutritional indications for using each one. Individuals who require elemental formulas because of impaired digestion or absorption or the specialized formulas for altered metabolic conditions usually require tube feeding because the unpleasant flavors of the free amino acids, peptides, or protein hydrolysates used in these formulas are very difficult to mask if taken in orally.
TABLE 8-4
FORMULA TYPE | NUTRITIONAL USES | CLINICAL EXAMPLES | EXAMPLES OF COMMERCIAL PRODUCTS (MANUFACTURER) |
Formulas Used When GI Tract Is Fully Functional | |||
Polymeric (standard): Contains whole proteins (10%-15% of calories), long-chain triglycerides (25%-40% of calories), and glucose polymers or oligosaccharides (50%-60% of calories); most provide 1 calorie/mL | Inability to ingest food Inability to consume enough to meet needs |
Oral or esophageal cancer Coma, stroke Anorexia resulting from chronic illness Burns or trauma |
Sepsis
Neurosurgery
COPD
Liver disease
Radiation enteritis
Inflammatory bowel disease
Dialysis
Hemodialysis or peritoneal dialysis
Sepsis
*These diets may be beneficial for selected patients; costs and benefits must be considered.
Immune-enhancing formulas (IEFs) have emerged as a means to protect and stimulate the immune system. Some of the enterally delivered nutrients that may benefit critically ill patients include fiber, the amino acids glutamine and arginine, the omega-3 (n-3) fatty acids, and the nucleotide ribonucleic acid (RNA).28 Fiber is not digested by humans but can be metabolized by gut bacteria to yield short-chain fatty acids, the primary fuel of the colon cells. Glutamine is the major fuel of the small intestinal cells. It is considered a nonessential amino acid, but it becomes conditionally essential in illness. It has been shown to improve mortality and infectious morbidity in critically ill patients.29–31 Arginine is involved in protein synthesis and is a precursor of nitric oxide, a molecule that stimulates vasodilation in the GI tract and heart and mediates hepatic protein synthesis during sepsis.28 The omega-3 fatty acids, derived primarily from fish oils, are involved in synthesis of eicosanoids (molecules with hormone-like activity)—prostaglandins, prostacyclin, and leukotrienes—and may modulate the inflammatory response.
There are a variety of commercial enteral feeding products, some of which are designed to meet the specialized needs of the critically ill. Products designed for the stressed patient with trauma or sepsis are usually rich in glutamine, arginine, branched amino acids (a major fuel source, especially for muscle), and antioxidant nutrients, such as selenium and vitamins C, E, and A.32 The antioxidants help to reduce oxidative injury to the tissues (e.g., from reperfusion injury). Despite the fact that IEFs may reduce the incidence of infectious complications, the efficacy and safety of these formulas in critically ill patients have not been clearly demonstrated.33–36
Early enteral nutrition, administered with the first 24 to 48 hours of critical illness, has been advocated as a way to reduce septic complications and improve feeding tolerance in critically ill patients. Although studies37 have shown a lower risk of infection and decreased length of stay with early enteral nutrition, the benefit of early enteral nutrition compared with enteral nutrition delayed a few days remains controversial.3,38 Current guidelines support the initiation of nutrition support in critically ill patients who will be unable to meet their nutrient needs orally for a period of 5 to 10 days.3 To avoid complications associated with intestinal ischemia and infarction, enteral nutrition must be initiated only after fluid resuscitation and adequate perfusion have been achieved.39,40
Critically ill patients may not tolerate early enteral feeding because of impaired gastric motility, ileus, or medications administered in the early phase of illness. This is particularly true for patients receiving gastric enteral feeding.41 The assessment of enteral feeding tolerance is an important aspect of nursing care. Monitoring of gastric residual volume is a method used to assess enteral feeding tolerance. However, evidence suggests that gastric residuals are insensitive and unreliable markers of tolerance to tube feeding.42 There is little evidence to support a correlation between gastric residual volumes and tolerance to feedings, gastric emptying, and potential aspiration. Except in selected high-risk patients, there is little evidence to support holding tube feedings in patients with gastric residual volumes less than 400 mL.42 The gastric residual volume should be evaluated within the context of other gastrointestinal symptoms. Prokinetic agents, including metoclopramide and erythromycin, have been used to improve gastric motility and promote early enteral nutrition in critically ill patients.43–45
Enteral Feeding Access.
Achievement of enteral access is the cornerstone of enteral nutrition therapy. Several techniques can be used to facilitate enteral access. These include surgical methods, bedside methods, fluoroscopy, endoscopy, air insufflation, and prokinetic agents.9 Placement of feeding tubes beyond the stomach (postpyloric) eliminates some of the problems associated with gastric feeding intolerance. However, placement of postpyloric feeding tubes is time-consuming and may be costly. Tubes with weights on the proximal end are available; they were originally designed for postpyloric feeding in the belief that that they would be more likely than unweighted tubes to pass spontaneously through the pyloric sphincter. However, randomized trials with the two types of tubes have shown that unweighted tubes are more likely to migrate through the pylorus than weighted tubes.46 The weights sometimes cause discomfort while being inserted through the nares. Unweighted tubes therefore may be preferable.
After the tube is placed, correct location must be confirmed before feedings are started and regularly throughout the course of enteral feedings. Radiographs are the most accurate way of assessing tube placement, but repeated radiographs are costly and can expose the patient to excessive radiation. After correct placement has been confirmed, marking the exit site of the tube to check for movement is helpful. Alternative methods for confirming tube placement have been researched that attempt to verify placement in the stomach or small intestine. An inexpensive and relatively accurate alternative method involves assessing the pH of fluid removed from the feeding tube; some tubes are equipped with pH monitoring systems. Assessing the pH and the bilirubin concentration in fluid aspirated from the feeding tube is a newer method for confirming tube placement.47
Location and Type of Feeding Tube.
Decisions regarding enteral access should be determined based on gastrointestinal anatomy, gastric emptying, and aspiration risk.3 Nasal intubation is the simplest and most commonly used route for enteral access. This method allows access to the stomach, duodenum, or jejunum. Tube enterostomy—a gastrostomy or jejunostomy—is used primarily for long-term feedings (6 to 12 weeks or more) and when obstruction makes the nasoenteral route inaccessible. Tube enterostomies may also be used for the patient who is at risk for tube dislodgment because of severe agitation or confusion. A conventional gastrostomy or jejunostomy is often performed at the time of other abdominal surgery. The percutaneous endoscopic gastrostomy (PEG) tube has become extremely popular because it can be inserted at the bedside without the use of general anesthetics. Percutaneous endoscopic jejunostomy (PEJ) tubes are also used.
Postpyloric feedings through nasoduodenal, nasojejunal, or jejunostomy tubes are commonly used when there is a high risk of pulmonary aspiration, because the pyloric sphincter theoretically provides a barrier that lessens the risk of regurgitation and aspiration.48 However, some studies have demonstrated that gastric feeding is safe and not associated with an increased risk of aspiration.49–51 Postpyloric feedings have an advantage over intragastric feedings for patients with delayed gastric emptying, such as those with head injury, gastroparesis associated with uremia or diabetes, or postoperative ileus. Delivery of enteral nutrition into the small bowel is associated with improved tolerance,52 higher calorie and protein intake,53 and fewer gastrointestinal complications.41 Small bowel motility returns more quickly than gastric motility after surgery, and it is often possible to deliver transpyloric feedings within a few hours of injury or surgery.48 Figure 8-3 shows the locations of tube feeding sites.
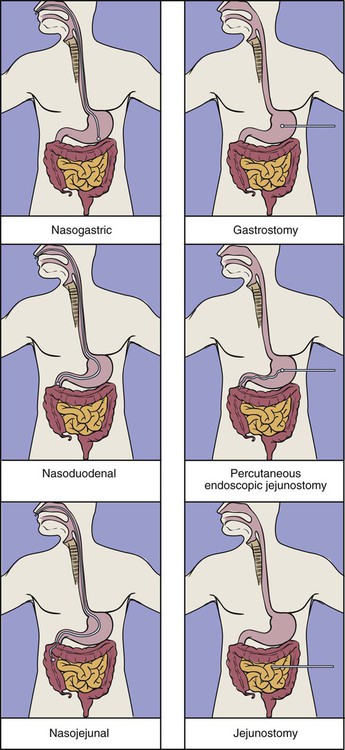
Assessment and Prevention of Feeding Tube Complications.
Nursing care of patients receiving enteral nutrition involves prevention and management of complications associated with the use of feeding tubes. Nursing management of these problems is summarized in Table 8-5. The skin around the feeding tube should be cleaned at least daily and the tape around the tube replaced whenever loosened or soiled. Secure taping helps to prevent movement of the tube, which may irritate the nares or oral mucosa or result in accidental dislodgment. The tube must be taped in the dependent position to prevent unnecessary pressure and prevent necrosis. Commercially available attachment devices may be used to avoid inadvertent dislodgment.
TABLE 8-5
NURSING MANAGEMENT OF ENTERAL TUBE FEEDING COMPLICATIONS
COMPLICATION | CONTRIBUTING FACTORS | PREVENTION OR CORRECTION |
Pulmonary aspiration (signs and symptoms include tachypnea, shortness of breath, hypoxia, and infiltrate on chest radiographs) | Feeding tube positioned in esophagus or respiratory tract | Confirm proper tube placement before administering any feeding; check tube placement at least every 4-8 hr during continuous feedings; consider intermittent feedings. |
Regurgitation of formula | Elevate head to 30-45 degrees during feedings unless contraindicated; if the head cannot be raised, position the patient in lateral (especially right lateral, which facilitates gastric emptying) or prone position to improve drainage of vomitus from the mouth. Consider giving feeding into small bowel rather than stomach in high-risk patients. | |
Metoclopramide may improve gastric emptying and decrease the risk of regurgitation. | ||
Evaluate feeding tolerance every 2 hr initially, then less frequently as condition becomes stable. Intolerance may be manifested by bloating, abdominal distention and pain, lack of stool and flatus, diminished or absent bowel sounds, tense abdomen, increased tympany, nausea and vomiting, residual volume >200 mL aspirated from an NG tube or >100 mL aspirated from a gastrostomy tube, although a high residual volume in the absence of other abnormal findings may not be grounds for stopping feedings (measuring residual volumes is a controversial practice; see the section on tube occlusion that follows below in this Table 8-5). If intolerance is suspected, abdominal radiographs may be done to check for distended gastric bubble, distended loops of bowel, or air-fluid levels. | ||
Diarrhea | Medications with GI side effects (e.g., antibiotics, digitalis, laxatives, magnesium-containing antacids, quinidine, caffeine, many others) | Evaluate the patient’s medications to determine their potential for causing diarrhea, and consult the pharmacist if necessary. |
Predisposing illness (e.g., short bowel syndrome, inflammatory bowel disease) | Use continuous feedings; consider a formula with MCT and/or soluble fiber. | |
Hypertonic formula or medications (e.g., oral suspensions of antibiotics, potassium, other electrolytes), which can cause dumping syndrome | Evaluate formula administration procedures to ensure that feedings are not being given by bolus infusion; administer the formula continuously or by slow intermittent infusion. Dilute enteral medications well. | |
Bacterial contamination of the formula | Use scrupulously clean technique in administering tube feedings; prepare formula with sterile water if there are any concerns about the safety of the water supply or if the patient is seriously immunocompromised; keep opened containers of formula refrigerated, and discard them within 24 hr; discard enteral feeding containers and administration sets every 24 hr; hang formula no more than 4-8 hr unless it comes prepackaged in sterile administration sets. | |
Fecal impaction with seepage of liquid stool around the impaction | Perform a digital rectal examination to rule out impaction; see guidelines for prevention of constipation that follow below in this table (Table 8-5). | |
Lactose intolerance | Use lactose-free formula. | |
Constipation | Low-residue formula, creating little fecal bulk, lack of fiber | Consider using a fiber-containing formula; ensure fluid intake is adequate; stool softeners may be beneficial. |
Tube occlusion | Medications administered by tube that physically plug the tube or coagulate the formula, causing it to clog the tube | If medications must be given by tube, avoid use of crushed tablets; consult with the pharmacist to determine whether medications can be dispensed as elixirs or suspensions. Irrigate tube with water before and after administering any medication; never add any medication to the formula unless the two are known to be compatible. |
Sedimentation of formula | Irrigate tube every 4-8 hr during continuous feedings and after every intermittent feeding. If residuals are measured, flush the tube thoroughly after returning the formula to the stomach, since gastric juices left in tube may cause precipitation of formula. Instilling pancreatic enzymes into the tube can remove or prevent some occlusions. | |
Gastric retention | Delayed gastric emptying related to head trauma, sepsis, diabetic or uremic gastroparesis, electrolyte balance, or other illness | The cause must be corrected if possible. Consult with the physician about use of postpyloric feedings or prokinetic agents to stimulate gastric emptying. Encourage the patient to lie in the right lateral position frequently, unless contraindicated. |
GI, Gastrointestinal; NG, nasogastric.
Modified from Moore MC. Pocket Guide to Nutritional Assessment and Care. 6th ed. St. Louis: Mosby; 2009.
Dressings are used initially around gastrostomy insertion sites. The dressing is changed daily and the skin cleansed with soap and water. If leakage of gastric fluid occurs around a gastrostomy tube, the integrity of the gastrostomy balloon should be evaluated.54 Karaya powder can be used to protect the peristomal skin from leakage. Fever, redness, purulent drainage, foul odor, or pain at the insertion site may indicate infection. Treatment may include application of a topical antibiotic ointment and daily cleansing with soap and water. Buried bumper syndrome may occur when the gastrostomy device disk, or bumper, is pulled tight against the abdominal wall. To prevent this, it is necessary to minimize tension on the disk. The tube may also become dislodged. A new gastrostomy tube needs to be reinserted within hours to prevent closure of the stoma.
Feeding Tube Occlusion.
Regular irrigation helps to prevent feeding tube occlusion. Usually, 20 to 30 mL of warm water every 3 to 4 hours during continuous feedings and before and after intermittent feedings and medication administration can maintain patency.3 The volume of irrigant may have to be reduced for fluid restriction. Automatic enteral flush pumps are also available. Although cranberry juice or cola beverages are sometimes used in an effort to reduce the incidence of tube occlusion, water is the preferred irrigant because it has been shown to be superior in maintaining tube patency.55 Tube occlusion may occur as a result of stagnant formula, inadequately crushed pills, or medication interactions with formula. Enteral infusion pumps should be used, and tubes should be flushed before feeding infusions are paused. Liquid medications or elixirs should be used when possible to avoid tube occlusion with pill fragments. The use of pancreatic enzymes in the feeding tube appears to reduce the risk of tube clogging and may be successful in removing a clog after it has formed.55,56
Aspiration.
Pulmonary aspiration of enteral formulas and subsequent pneumonia is a serious complication of enteral feeding in critically ill patients. Risk factors for aspiration of enteral feeding include decreased level of consciousness, supine position, and swallowing disorders.57 Figure 8-4 shows the risk factors for aspiration in patients on tube feeds. To reduce the risk of pulmonary aspiration of formula during enteral feeding, the nurse must keep the head of the bed elevated unless contraindicated; temporarily stop feedings when the patient must be supine for prolonged periods; position the patient in the right lateral decubitus position when possible to encourage gastric emptying; use postpyloric feeding methods; keep the cuff of the endotracheal tube inflated as much as possible during enteral feeding, if applicable; and be alert to any increase in abdominal distention.
Two bedside methods have been used in the past to detect pulmonary aspiration of enteral feeding. One is the addition of blue dye to the enteral formula and observation of the patient for any dye-tinged tracheal secretions, and the other is glucose testing of tracheal secretions to detect the presence of the glucose-containing enteral formula. The glucose oxidase method may cause false-positive reactions if blood is present. It has a low sensitivity when low-glucose formulas are used, and it has questionable specificity.58 There is no established protocol for blue dye testing. Although it has been used routinely in clinical practice for several years, there is no evidence to support its efficacy or safety. It lacks sensitivity and specificity in ruling out aspiration. Numerous clinical reports of systemic absorption of blue dye and adverse outcomes have been described.59,60 Glucose oxidase testing and blue food coloring are not recommended as appropriate methods for detecting aspiration of enteral feedings.59,61
Gastrointestinal Complications.
Diarrhea is common in patients receiving enteral nutrition, with an incidence of 2% to 70%.62 No single definition has been established for diarrhea. Current definitions include various stool frequencies, volumes, and weights.63 Diarrhea in enterally fed critically ill patients has many factors. Common causes include medications, malabsorption, formula contamination, or low-fiber formulas. While the cause of diarrhea is being determined, nurses must provide adequate fluid and electrolyte replacement, maintain skin integrity, and administer antidiarrheal agents. To prevent complications, stool must be checked for infection, especially Clostridium difficile, before antidiarrheal medications can be administered.64 Constipation is a complication of enteral feeding that may result from dehydration, bed rest, opioid administration, or lack of adequate fiber in enteral formulas. Pasty stools are normal in enterally fed patients. Bowel movements should be assessed daily. The nurse must ensure adequate fluid and fiber intake, promote optimal mobility, and administer laxatives and stool softeners as necessary.65
Adequacy of Enteral Nutrition.
Critically ill patients have so many needs for care that it is easy to overlook the importance of nutrition. Many studies have shown that critically ill patients receive considerably less enteral nutrition than required.66–69 This is a complication unique to enteral nutrition not observed with TPN. The discrepancy in nutritional intake has a variety of causes, including patient factors (e.g., high residual volumes, emesis, abdominal distention), tube-related factors (e.g., occlusion, malposition), and treatment-related factors (e.g., interruptions caused by procedures, airway management, and medications).67 Inadequate enteral nutrition delivery is also related to physicians’ prescribing practices in the critical care unit.69 The enteral delivery practices in the critical care unit and clinicians’ concerns about aspiration may lead to inappropriate and prolonged interruptions in enteral feeding. Chest physiotherapy, suspicion of formula in the tracheobronchial secretions, and excessive gastric retention of formula are examples of appropriate reasons for stopping feedings. Enteral nutrition delivery practices that are evidence-based are necessary for optimal nutritional outcomes in critically ill patients.
Tubing and Catheter Misconnections.
Tubing and catheter or Luer connector (small device used in the connection of many medical components and accessories) misconnection errors have gain recent attention with The Joint Commission issuing a Sentinel Event Alert in April 2006.70 Examples of misconnection errors include misconnecting an enteric feeding tube into an intravenous catheter or injection of an intravenous fluid into a tracheostomy cuff inflation tube. These misconnection errors are potentially life threatening, and increased awareness as well as precautions can improve patient safety. The following strategies should be followed to minimize risk for tubing and catheter misconnections71:
• Label all tubes and catheters, especially arterial, epidural, and intrathecal catheters.
• Trace lines back to their origins when initiating any new device or infusion.
• Standardize a line reconciliation process with patient handoffs.
• Consider routing tubes and catheters with different purposes in different, standardized directions.
• Never use a standard Luer syringe for oral medications or enteric feedings.
Total Parenteral Nutrition
Types of Parenteral Nutrition.
TPN involves administration of highly concentrated dextrose (25% to 70%), providing a rich source of calories. These highly concentrated dextrose solutions are hyperosmolar, as much as 1800 mOsm/L, and therefore must be delivered through a central vein.72 Peripheral parenteral nutrition (PPN) has a glucose concentration of 5% to 10% and may be delivered safely through a peripheral vein. PPN solution delivers nutrition support in a large volume that cannot be tolerated by patients who require fluid restriction. It provides short-term nutrition support for a few days to less than 2 weeks.
Nursing Management of Potential Complications.
Nursing management of the patient receiving TPN includes catheter care, administration of solutions, prevention or correction of complications, and evaluation of patient responses to intravenous feedings. Table 8-6 describes nursing management of TPN complications. Evaluation of the patient’s response is discussed later in this chapter.
TABLE 8-6
NURSING MANAGEMENT OF TOTAL PARENTERAL NUTRITION COMPLICATIONS
COMPLICATION | CLINICAL MANIFESTATIONS | PREVENTION OR CORRECTION |
Catheter-related sepsis | Fever, chills, glucose intolerance, positive blood culture | Use aseptic technique when handling catheter, IV tubing, and TPN solutions. Hang a bottle of TPN no longer than 24 hr, lipid emulsion no longer than 12-24 hr. Use an in-line 0.22-micron filter with TPN to remove microorganisms. Avoid drawing blood, infusing blood or blood products, piggybacking other IV solutions into TPN IV tubing, or attaching manometers or transducers through the TPN infusion line, if possible. |
If catheter-related sepsis is suspected, remove the catheter or assist in changing the catheter over a guidewire and administer antibiotics as ordered. | ||
Air embolism | Dyspnea, cyanosis, apnea, tachycardia, hypotension, “millwheel” heart murmur; mortality estimated at 50% (depends on quantity of air entering) | Use Luer-Lok connections; use an in-line air-eliminating filter. Have the patient perform a Valsalva maneuver during tubing changes; if the patient is on a ventilator, change tubing quickly at end expiration. Maintain occlusive dressing over catheter site for at least 24 hr after removing catheter to prevent air entry through catheter tract. If an air embolism is suspected, place the patient in left lateral decubitus and Trendelenburg positions (to trap air in the apex of the right ventricle, away from the outflow tract) and administer oxygen and CPR as needed; immediately notify physician, who may attempt to aspirate air from the heart. |
Pneumothorax | Chest pain, dyspnea, hypoxemia, hypotension, radiographic evidence, needle aspiration of air from pleural space | Thoroughly explain the catheter insertion procedure to the patient, because when a patient moves or breathes erratically, he or she is more likely to sustain pleural damage. Perform x-ray examination after insertion or insertion attempt. |
If pneumothorax is suspected, assist with needle aspiration or chest tube insertion, if necessary. | ||
Central venous thrombosis | Edema of neck, shoulder, and arm on same side as catheter; development of collateral circulation on chest; pain in insertion site; drainage of TPN from the insertion site; positive findings on venography | Follow measures to prevent sepsis; repeated or traumatic catheterizations are most likely to result in thrombosis. If thrombosis is confirmed, remove the catheter, and administer anticoagulants and antibiotics, as ordered. |
Catheter occlusion or semi-occlusion | No flow or a sluggish flow through the catheter | If the infusion is stopped temporarily, flush the catheter with saline or heparinized saline. |
If the catheter appears to be occluded, attempt to aspirate the clot; if this is ineffective, the physician may order a thrombolytic agent such as streptokinase or alteplase (tPA) instilled in the catheter. | ||
Hypoglycemia | Diaphoresis, shakiness, confusion, loss of consciousness | Infuse TPN within 10% of the ordered rate; monitor blood glucose until stable. If hypoglycemia is present, administer oral carbohydrate; if the patient is unconscious or oral intake is contraindicated, the physician may order an IV bolus of dextrose. |
Hyperglycemia | Thirst, headache, lethargy, increased urinary output | Administer TPN within 10% of the ordered rate; monitor blood glucose level at least daily until stable. The patient may require insulin added to the TPN if hyperglycemia is persistent; sudden appearance of hyperglycemia in a patient who was previously tolerating the same glucose load may indicate the onset of sepsis. |
Hypertriglyceridemia | Serum triglyceride concentrations elevated (especially serious if >400 mg/dL); serum may appear turbid | Monitor serum triglycerides at baseline, 6 hr after lipid infusion, and at least 3 times weekly until stable in patients receiving lipid emulsions; reduce lipid infusion rate or administer low-dose heparin with lipid emulsions as ordered if elevated levels are observed. |
CPR, cardiopulmonary resuscitation; IV, intravenous; TPN, total parenteral nutrition.
Modified from Moore MC. Pocket Guide to Nutritional Assessment and Care. 6th ed. St. Louis: Mosby; 2009.
Some clinical studies have reported that catheter-related sepsis is higher with multilumen catheters; others have found no difference compared with single-lumen catheters.73 Patients requiring multilumen catheters are likely to be very ill and immunocompromised, and scrupulous aseptic technique is essential in maintaining their multilumen catheters. The manipulation involved in frequent changes of intravenous fluid and obtaining blood specimens through these catheters increases the risk of catheter contamination. Peripherally inserted central catheters (PICC) allow central venous access through long catheters inserted in peripheral sites. This reduces the risk of complications associated with percutaneous cannulation of the subclavian vein and provides an alternative to PPN.74
The indwelling central venous catheter provides an excellent nidus for infection. Catheter-related infections arise from endogenous skin flora, contamination of the catheter hub, seeding of the catheter by organisms carried in the bloodstream from another site, or contamination of the infusate. Good hand washing and scrupulous aseptic technique in all aspects of catheter care and TPN delivery are the primary steps for prevention of catheter-related infections. Other measures to reduce the incidence of catheter-related infections include using maximal barrier precautions (e.g., cap, mask, sterile gloves, sterile drape) at the time of insertion, tunneling the catheter underneath the skin, use of a 2% chlorhexidine preparation for skin cleansing, no routine replacement of the central venous catheter for prevention of infection, and use of antiseptic- or antibiotic-impregnated central venous catheters.75
Metabolic complications associated with parenteral nutrition include glucose intolerance and electrolyte imbalance. Slow advancement of the rate of TPN (25 mL/hr) to goal rate allows pancreatic adjustment to the dextrose load. Capillary blood glucose should be monitored every 4 to 6 hours. Insulin can be added to the TPN solution or can be infused as a separate drip to control glucose levels. Rapid cessation of TPN may not lead to hypoglycemia; however, tapering the infusion over 2 to 4 hours is recommended.76
Serum electrolytes are obtained on starting TPN. During critical illness, levels should be monitored and corrected daily and then weekly or twice weekly after the patient is more stable. The refeeding syndrome is a potentially lethal condition characterized by generalized fluid and electrolyte imbalance. It occurs as a potential complication after initiation of oral, enteral, or parenteral nutrition in malnourished patients. During chronic starvation, several compensatory metabolic changes occur. The reintroduction of carbohydrates and amino acids leads to increased insulin production. This creates an anabolic environment that increases intracellular demand for phosphorus, potassium, magnesium, vitamins, and minerals.77 These metabolic demands result in severe shifts from the extracellular compartment. Increased insulin levels also result in fluid retention. Severe hypophosphatemia, hypokalemia, and hypomagnesemia result in altered cardiac, gastrointestinal, and neurologic function. In particular, hypophosphatemia causes a decrease in 2,3-diphosphosoglycerate (2,3-DPG) and limits the many reactions that require ATP. Hypophosphatemia and other electrolyte deficiencies may lead to respiratory failure, congestive heart failure, and dysrhythmias.
It is important to anticipate refeeding syndrome in patients who may be at risk. Patients with chronic malnutrition or underfeeding, chronic alcoholism, or anorexia nervosa or those maintained NPO for several days with evidence of stress are at risk for refeeding syndrome.78 In high-risk patients, nutrition support should be started cautiously at 25% to 50% of required calories and slowly advanced over 3 to 4 days as tolerated. Close monitoring of serum electrolytes before and during feeding is essential. Normal values do not always reflect total body stores. Correction of pre-existing electrolyte imbalances are necessary before initiation of feeding. Continued monitoring and supplementation with electrolytes and vitamins are necessary throughout the first week of nutrition support.78
Lipid Emulsion.
Lipids or fat emulsions provide calories for energy and prevent essential fatty acid depletion. In contrast to dextrose–amino acid solutions, intravenous lipid emulsions provide a rich environment for the growth of bacteria and fungi, including Candida albicans. Lipid emulsions cannot be filtered through an in-line 0.22-micron filter, because some particles in the emulsions have larger diameters than this. Lipids may be infused into the TPN line downstream from the filter. No other medications should be infused into a line containing lipids or TPN. Lipid emulsions are handled with strict asepsis, and they must be discarded within 12 to 24 hours of hanging. There is a trend toward mixing lipid emulsions with dextrose–amino acid TPN solutions; these are called 3-in-1 solutions or total nutrient admixtures (TNAs). Consolidating the nutrients in one container is more economical and saves nursing time, although TNA solutions may be less stable.72
Monitoring and Evaluation of Nutrition Support
Recently, the Society of Critical Care Medicine (SCCM) and the American Society for Parenteral and Enteral Nutrition (A.S.P.E.N.) published The Guidelines for the Provision and Assessment of Nutrition Support Therapy in the Adult Critically Ill Patient. The publication is based on an extensive review of 307 articles and is intended for the care of critically ill adults who require a stay of greater than 3 days in the critical care area. All practitioners who care for this target population are encouraged to become familiar with these evidence-based guidelines.3
Nutrition and Cardiovascular Alterations
Nutrition Assessment in Cardiovascular Alterations
A nutrition assessment provides the nurse and other members of the health care team the information necessary to plan the patient’s nutrition care and education. Common findings in the nutrition assessment of the cardiovascular patient are summarized in Box 8-4. The major nutritional concerns relate to appropriateness of body weight and the levels of serum lipids and blood pressure.
Nutrition Intervention and Education in Cardiovascular Alterations
Myocardial Infarction
Long-Term Changes
For the individual who is overweight or obese, gradual loss of weight (0.45 to 0.9 kg [1 to 2 lb] per week) is the goal.79 Weight loss can be achieved through moderate exercise (with the physician’s approval) and reduction of dietary intake by 500 to 1000 kcal/day. Dietary guidelines for the National Cholesterol Education Program (NCEP) Adult Treatment Panel III (ATP III) support lipid-lowering therapy through adoption of a low-saturated-fat and low-cholesterol diet, maintenance of a healthy weight, and regular physical activity.80 Recommendations include low-density lipoprotein (LDL) cholesterol levels of 100 mg/dL or less as optimal and increased focus on the metabolic syndrome.81,82 Metabolic syndrome is a group of risk factors (Table 8-7) associated with increased risk for cardiovascular disease and diabetes. The primary target for patients with metabolic syndrome is weight reduction reinforced with increased physical activity, as it is expected that lipid parameters and insulin resistance will be improved with a decrease in obesity.83,84
TABLE 8-7
METABOLIC SYNDROME* DEFINITION
RISK FACTOR | DEFINING LEVEL |
Elevated waist circumference | |
Men | ≥40 in (≥102 cm) |
Women | ≥35 in (≥88 cm) |
Elevated triglycerides | ≥150 mg/dL (≥1.7 mmol/L) |
or on medication treatment for elevated triglycerides | |
Reduced HDL cholesterol | |
Men | <40 mg/dL (<1.03 mmol/L) |
Women | <50 mg/dL (<1.3 mmol/L) |
or on medication treatment for reduced HDL cholesterol | |
Elevated blood pressure | ≥130 mm Hg systolic or ≥85 mm Hg diastolic |
or on antihypertensive medication treatment in a patient with a history of hypertension | |
Elevated fasting glucose | ≥100 mg/dL (≥5.6 mmol/L) |
or on medication treatment for elevated glucose |
*Metabolic Syndrome diagnosed when 3 of 5 of the listed risk factors are present.
Adapted from Grundy SM, et al. Diagnosis and management of the metabolic syndrome: an American Heart Association/National Heart, Lung, and Blood Institute scientific statement. Circulation. 2005;112:2735.
Elevated plasma levels of homocysteine, derived from the essential amino acid methionine, are a risk factor for heart disease. Homocysteine can damage the endothelium of the blood vessels, cause proliferation of smooth muscle in the vessel walls, and activate platelets and the coagulation cascade, contributing to thrombus formation. Adequate amounts of folic acid and vitamins B6 and B12 are known to reduce homocysteine levels. Although elevated homocysteine levels remain a risk marker for heart disease, current research does not support treatment with folic acid, B6 and B12 in lowering homocysteine levels to prevent risk of death from cardiovascular disease.85
For hypertensive cardiac disease, sodium chloride restriction is recommended. Some individuals have been shown to be more salt sensitive than others, and this salt sensitivity contributes to hypertension. Adoption of a healthy lifestyle is critical for the prevention of high blood pressure and is an indispensable part of the management of those with hypertension.86 Weight loss of as little as 10 pounds reduces blood pressure. Adoption of the Dietary Approaches to Stop Hypertension (DASH)87 eating plan also benefits blood pressure. DASH comprises a diet rich in fruits, vegetables, and low-fat dairy products with a reduced content of dietary cholesterol and reduced levels of saturated and total fat. It is rich in potassium and calcium content. Dietary sodium should be reduced to no more than 100 mmol per day (2.4 g of sodium). Alcohol intake should be limited to no more than 1 ounce (30 mL) of ethanol per day in men and no more than 0.5 ounce of ethanol in women.
Heart Failure
Nutrition intervention in heart failure is designed to reduce fluid retained within the body and therefore reduce the preload. Because fluid accompanies sodium, limitation of sodium is necessary to reduce fluid retention. Specific interventions include limiting salt intake, usually to 2 g a day or less, and limiting fluid intake as appropriate.88 If fluid is restricted, the daily fluid allowance is usually 1.5 to 2 L/day, which includes fluids in the diet and those given with medications and for other purposes (see “Heart Failure” in Chapter 15).
Cardiac Cachexia
Malnutrition is common in patients with heart failure. The term cachexia is derived from the Greek words kakos, meaning “bad,” and hexis, meaning “condition.” It is characterized by weight loss, anorexia, weakness, early satiety, and edema.89 Cachexia is seen in a variety of disorders, including cancer and heart failure. It is well recognized as an independent predictor of higher mortality in patients with heart failure.90 Sodium and fluid restriction are appropriate interventions. It is important to concentrate nutrients into as small a volume as possible and to serve small amounts frequently, rather than three large meals daily, which may overwhelm the patient. The individual should be encouraged to consume calorie-dense foods and supplements.
Nutrition and Pulmonary Alterations
Malnutrition has extremely adverse effects on respiratory function, decreasing surfactant production, diaphragmatic mass, vital capacity, and immunocompetence. Patients with acute respiratory disorders find it difficult to consume adequate oral nutrients and can rapidly become malnourished. Individuals who have an acute illness superimposed on chronic respiratory problems are also at high risk. Nearly three fourths of patients with chronic obstructive pulmonary disease (COPD) have had weight loss.91 Patients with undernutrition and end-stage COPD, however, often cannot tolerate the increase in metabolic demand that occurs during refeeding. They also are at significant risk for development of cor pulmonale and may fail to tolerate the fluid required for delivery of enteral or parenteral nutrition support. Prevention of severe nutritional deficits, rather than correction of deficits after they have occurred, is important in nutritional management of these patients (see “Acute Respiratory Failure” and “Long-Term Mechanical Ventilator Dependence” in Chapter 20).
Nutrition Assessment in Pulmonary Alterations
Common findings in nutrition assessment related to pulmonary alterations are summarized in Box 8-5. The patient with respiratory compromise is especially vulnerable to the effects of fluid volume excess and must be assessed continually for this complication, particularly during enteral and parenteral feeding.
Nutrition Intervention and Education in Pulmonary Alterations
Prevent or Correct Undernutrition and Underweight
Avoid Overfeeding
Overfeeding of total calories or of carbohydrate or lipid alone can impair pulmonary function. The production of carbon dioxide (Vco2) increases when carbohydrate is relied on as the primary energy source. This is unlikely to be significant in the patient who is eating foods. Instead, it is an iatrogenic complication of TPN, in which glucose is often the predominant calorie source, or occasionally of tube feeding in a patient with a very high carbohydrate formula. Excessive calorie intake can raise Paco2 sufficiently to make it difficult to wean a patient from the ventilator. A balanced regimen with lipids and carbohydrates providing the nonprotein calories is optimal for the patient with respiratory compromise, and the patient needs to be reassessed continually to ensure that caloric intake is not excessive.3
Excessive lipid intake can impair capillary gas exchange in the lungs, although this is not usually sufficient to produce an increase in Paco2 or decrease in Pao2.92 However, the patient with severe respiratory alteration may be further compromised by lipid overdose. If lipid intake is maintained at no more than 2 g/kg/day, lipid excess is rarely a problem. Serum triglyceride levels greater than 400 mg/dL may indicate inadequate lipid clearance and a need to decrease the lipid dosage.
Nutrition and Neurologic Alterations
Nutrition Intervention and Education in Neurologic Alterations
Prevention or Correction of Nutritional Deficits
Oral Feedings.
Patients with dysphagia or weakness of the swallowing musculature often experience the greatest difficulty in swallowing foods that are dry or thin liquids, such as water, that are difficult to control. For these patients, the nurse, the dietitian, and the speech therapist can work together to plan suitable meals and evaluate patient acceptance and tolerance (see “Stroke” in Chapter 24).
Tube Feedings or Total Parenteral Nutrition.
Patients with neurologic deficits are at increased risk for certain complications (particularly pulmonary aspiration) during tube feeding and therefore require especially careful nursing management. Patients of most concern are 1) those with an impaired gag reflex, such as some patients with cerebral vascular accident; 2) those with delayed gastric emptying, such as patients in the early period after spinal cord injury and patients with head injury treated with barbiturate coma; and 3) patients likely to experience seizures. To help prevent pulmonary aspiration, the patient’s head is kept elevated at an angle of 30 to 45 degrees, if not contraindicated; when elevation of the head is not possible, administering feedings with the patient in the prone or lateral position allows free drainage of emesis from the mouth and decreases the risk of aspiration (see “Aspiration Pneumonitis” in Chapter 20).
Administering phenytoin with enteral formulas decreases the absorption of the medication and the peak serum level achieved. A problem arises when a patient is receiving continuous enteral feedings and requires anticonvulsant therapy. One way to deal with the problem is stop the feeding for 1 to 2 hours before and after phenytoin administration.93 Even when this practice is followed, the patient may require a higher phenytoin dosage than normal to maintain therapeutic serum concentrations. When continuous feedings are discontinued and the patient resumes eating meals or receives intermittent enteral feedings, the phenytoin dosage must be adjusted appropriately. Phenytoin levels should be monitored carefully in patients receiving enteral feedings. The infusion rate may need to be increased to account for the time that the enteral feeding is held for phenytoin administration.
Prompt use of nutrition support is especially important for patients with head injuries because head injury causes marked catabolism, even in patients who receive barbiturates, which should decrease metabolic demands. Head-injured patients rapidly exhaust glycogen stores and begin to use body proteins to meet energy needs, a process that can quickly lead to PCM. The catabolic response is partly a result of corticosteroid therapy in head-injured patients. However, the hypermetabolism and hypercatabolism are also caused by dramatic hormonal responses to this type of injury.94 Levels of cortisol, epinephrine, and norepinephrine increase as much as seven times normal. These hormones increase the metabolic rate and caloric demands, causing mobilization of body fat and proteins to meet the increased energy needs. Head-injured patients undergo an inflammatory response and may be febrile, creating increased needs for protein and calories. Improvement in outcome and reduction in complications have been observed in head-injured patients who receive adequate nutrition support early in the hospital course94,95 (see Traumatic Brain Injuries in Chapter 34).
Prevention of Overweight and Obesity.
Many stable patients with neurologic disorders are less active than their healthy counterparts and require fewer calories. They may become overweight or obese if given normal amounts of calories for their age and gender. Within 1 or 2 months after spinal cord injury, substantial amounts of muscle atrophy and loss of body mass begin to occur as a result of denervation and disuse. Consequently, body weight and caloric needs decline. Ideal body weights for paraplegics and quadriplegics are less than those for healthy adults of the same height.96 Stable, rehabilitating paraplegics need approximately 27.9 calories/kg/day, and quadriplegics need approximately 22.7 calories/kg/day.96,97 Patients with dysphagia or extreme swallowing musculature weakness may rely on very soft, easy-to-chew foods that are usually more dense in calories than are bulky, high-fiber foods. They also may gain unneeded weight that will hamper their care and impede mobility. For these reasons, nutrition education of the patient and family coping with a spinal cord injury should include instruction about prevention of undesirable weight gain. Decreased use of high-fat foods (e.g., milk—such as shakes, ice cream, butter, margarine, pastries) can help to reduce calorie intake. Fruits and vegetables without added fat or sauces are good choices because they are generally low in fat and supply fiber needed to help maintain regular bowel habits.
Nutrition and Renal Alterations
Nutrition Intervention and Education in Renal Alterations
Protein
The kidney is responsible for excreting nitrogen from amino acids or proteins in the form of urea. When urinary excretion of urea is impaired in renal failure, BUN rises. Excessive protein intake may worsen uremia. However, the patient with renal failure often has other physiologic stresses that increase protein or amino acid needs: losses because of dialysis, wounds, and fistulas; use of corticosteroid medications that exert a catabolic effect; increased endogenous secretion of catecholamines, corticosteroids, and glucagon, all of which can cause or aggravate catabolism; metabolic acidosis, which stimulates protein breakdown; and catabolic conditions, such as trauma, surgery, and sepsis.98 Patients with acute kidney injury need adequate amounts of protein to avoid catabolism of body tissues.
Patients with stable acute kidney injury without evidence of fluid overload or electrolyte or acid-base disturbances can often be managed conservatively without dialysis. However, when renal function worsens, some form of renal replacement therapy (RRT) is required to maintain homeostasis and prevent metabolic complications. Types of RRT include peritoneal dialysis, intermittent hemodialysis, and continuous arteriovenous (AV) hemofiltration.98 During hemodialysis, amino acids are freely filtered and lost, but proteins such as albumin and immunoglobulin are not. Proteins and amino acids are removed during peritoneal dialysis, creating a greater nutritional requirement for protein.99,100 Protein needs may be higher, depending on the level of stress.101 To limit catabolism, patients with acute kidney injury on dialysis therapy should receive approximately 1.5 to 2.0 g of protein/kg/day (with a maximum of 2.5 g of protein/kg/day), depending on catabolic rate, renal function, and dialysis losses.3,102
Fluid
The patient with renal insufficiency usually does not require a fluid restriction until urine output begins to diminish. Patients receiving hemodialysis are limited to a fluid intake resulting in a gain of no more than 0.45 kg (1 lb) per day on the days between dialysis. This generally means a daily intake of 500 to 750 mL plus the volume lost in urine. With the use of continuous peritoneal dialysis, hemofiltration, or hemodialysis, the fluid intake can be liberalized.103 This more liberal fluid allowance permits more adequate nutrient delivery by oral, tube, or parenteral feedings. Enteral formulas containing 1.5 to 2 calories/mL or more provide a concentrated source of calories for tube-fed patients who require fluid restriction. Intravenous lipids, particularly 20% emulsions, can be used to supply concentrated calories for the TPN patient. Intradialytic TPN can be given during hemodialysis sessions to some malnourished patients; it supplies an additional source of nutrients at a time when the fluid can be rapidly removed in dialysis.99
Energy (Calories)
Energy needs are not increased by renal failure, but adequate calories must be provided to avoid catabolism.98,103 It is essential that the renal patient receive an adequate number of calories to prevent catabolism of body tissues to meet energy needs. Catabolism reduces the mass of muscle and other functional body tissues, and it releases nitrogen that must be excreted by the kidney. Adults with renal insufficiency need about 30 to 35 calories/kg/day to prevent catabolism and ensure that all protein consumed is used for anabolism rather than to meet energy needs.103 After renal transplantation, when the patient usually receives large doses of corticosteroids, it is especially important to ensure that caloric intake is adequate (usually 25 to 35 calories/kg/day) to prevent undue catabolism.

Other Nutrients
Certain nutrients such as potassium and phosphorus are restricted because they are excreted by the kidney. The patient has no specific requirement for the fat-soluble vitamins A, E, and K, because they are not removed in appreciable amounts by dialysis and restriction generally prevents development of toxicity. Patients with end-stage renal disease may have decreased clearance of vitamin A, and levels should be monitored.102 The needs for several water-soluble vitamins and trace minerals are increased in the dialysis patient because they are small enough to pass freely through the dialysis filter. Vitamin and minerals should be supplemented as necessary.103
Nutrition and Gastrointestinal Alterations
Nutrition Intervention and Education in Gastrointestinal Alterations
Hepatic Failure
The liver is the most important metabolic organ, and it is responsible for carbohydrate, fat and protein metabolism, vitamin storage and activation, and detoxification of waste products. Liver failure is associated with a wide spectrum of metabolic alterations. Because the diseased liver has impaired ability to deactivate hormones, levels of circulating glucagon, epinephrine, and cortisol are elevated. These hormones promote catabolism of body tissues and cause glycogen stores to be exhausted. Release of lipids from their storage depots is accelerated, but the liver has decreased ability to metabolize them for energy. Moreover, inadequate production of bile salts by the liver results in malabsorption of fat from the diet. Body proteins are used for energy sources, producing tissue wasting. The branched-chain amino acids (BCAAs)—leucine, isoleucine, and valine—are especially well used for energy, and their levels in the blood decline. Conversely, levels of the aromatic amino acids (AAAs)—phenylalanine, tyrosine, and tryptophan—increase as a result of tissue catabolism and impaired ability of the liver to clear them from the blood. BCAAs are not as dependent on liver metabolism as the AAAs are.104 The AAAs are precursors for neurotransmitters (serotonin and dopamine) within the central nervous system. Rising levels of AAAs cause encephalopathy by promoting synthesis of false neurotransmitters that compete with endogenous neurotransmitters. The damaged liver cannot clear ammonia from the circulation adequately, and ammonia accumulates in the brain. The ammonia may contribute to the encephalopathic symptoms and to brain edema.3,104
Monitoring Fluid and Electrolyte Status.
Ascites and edema are caused by a combination of factors. Colloid osmotic pressure in the plasma decreases because of the reduction of production of albumin and other plasma proteins by the diseased liver, increased portal pressure caused by obstruction, and renal sodium retention from secondary hyperaldosteronism. To control the fluid retention, restriction of sodium (usually 2000 mg) and fluid (1500 mL or less daily) usually is necessary in conjunction with the administration of diuretics. Patients are weighed daily to evaluate the success of treatment. Physical status and laboratory data must be closely monitored for deficiencies of potassium, phosphorus, zinc, and vitamins A, D, E, and K.3
Provision of a Nutritious Diet and Evaluation of Response to Dietary Protein.
PCM and nutritional deficiencies are common in patients with liver failure. The causes of malnutrition are complex and usually are related to decreased intake, malabsorption, maldigestion, and abnormal nutrient metabolism. Nutrition intervention is individualized and based on these metabolic changes. A diet with adequate protein helps to suppress catabolism and promote liver regeneration. Stable patients with cirrhosis usually tolerate 0.8 to 1 g of protein/kg/day. Patients with severe stress or nutritional deficits have increased needs—as much as 1.2 to 2 g of protein/kg/day.104 Aggressive treatment with medications, including lactulose, neomycin, or metronidazole, is considered first-line therapy in the management of acute hepatic encephalopathy. Chronic protein restriction, which could lead to PCM, is not recommended as a long-term management strategy for patients with liver disease.3,104
Anorexia may interfere with oral intake, and the nurse may need to provide much encouragement to the patient to ensure intake of an adequate diet. Prospective calorie counts may need to be instituted to provide objective evidence of oral intake. Small, frequent feedings are usually better tolerated by the anorexic patient than are three large meals daily. Soft foods are preferred because the patient may have esophageal varices that may be irritated by high-fiber foods. If patients are unable to meet their caloric needs, they may require oral supplements or enteral feeding. Small-bore nasoenteric feeding tubes can be used safely without increasing risk of variceal bleeding.104 TPN should be reserved for patients who are absolutely unable to tolerate enteral feeding.3 Diarrhea from concurrent administration of lactulose should not be confused with feeding intolerance.
A diet adequate in calories (at least 30 calories/kg daily) is provided to help prevent catabolism and to prevent the use of dietary protein for energy needs.105 In cases of malabsorption, medium-chain triglycerides (MCTs) may be used to meet caloric needs. Pancreatic enzymes may also be considered for malabsorption problems.
BCAA-enriched products have been developed for enteral and parenteral nutrition of patients with hepatic disease. These products may be used in patients with acute hepatic encephalopathy who do not tolerate standard diets or enteral formulas or who are unresponsive to lactulose. However, no substantial evidence exists showing BCAAs are superior to standard formulas in regard to nitrogen balance or as treatment for encephalopathy.3,104 The patient who undergoes successful liver transplantation is usually able to tolerate a regular diet with few restrictions. Intake during the postoperative period must be adequate to support nutritional repletion and healing; 1 to 1.2 g of protein/kg/day and approximately 30 calories/kg/day are usually sufficient. Immunosuppressant therapy (corticosteroids and cyclosporine or tacrolimus) contributes to glucose intolerance. Dietary measures to control glucose intolerance include 1) obtaining approximately 30% of dietary calories from fat; 2) emphasizing complex sources of carbohydrates; and 3) eating several small meals daily. Moderate exercise often helps to improve glucose tolerance.
Pancreatitis
The pancreas is an exocrine and endocrine gland required for normal digestion and metabolism of proteins, carbohydrates, and fats. Acute pancreatitis is an inflammatory process that occurs as a result of autodigestion of the pancreas by enzymes normally secreted by that organ. Food intake stimulates pancreatic secretion, increasing the damage to the pancreas and the pain associated with the disorder. Patients usually present with abdominal pain and tenderness and with elevations of pancreatic enzymes. A mild form of acute pancreatitis occurs in 80% of patients requiring hospitalization, and a severe form of acute pancreatitis occurs in the other 20%.106 Patients with the mild form of acute pancreatitis do not require nutrition support and generally resume oral feeding within 7 days. Chronic pancreatitis may develop, and it is characterized by fibrosis of pancreatic cells. This results in loss of exocrine and endocrine function because of the destruction of acinar and islet cells. The loss of exocrine function leads to malabsorption and steatorrhea. In chronic pancreatitis, the loss of endocrine function results in impaired glucose intolerance.106
Prevention of Further Damage to the Pancreas and Preventing Nutritional Deficits.
Effective nutritional management is a key treatment for patients with acute pancreatitis or exacerbations of chronic pancreatitis. The concern that feeding may stimulate the production of digestive enzymes and perpetuate tissue damage has led to the widespread use of TPN and bowel rest. Recent data suggest that for patients with severe pancreatitis, providing enteral nutrition support is more beneficial than prolonged bowel rest and provision of TPN.3,107 Enteral nutrition infused into the distal jejunum bypasses the stimulatory effect of feeding on pancreatic secretion and is associated with fewer infectious and metabolic complications compared with TPN.108,109
The results of randomized studies comparing TPN with total enteral nutrition (TEN, or enteral tube feeding) indicate that TEN is preferable to TPN in patients with severe acute pancreatitis, reducing costs and the risk of sepsis and improving clinical outcome.108–110 Patients unable to tolerate TEN should receive TPN, and some patients may require a combination of TEN and TPN to meet nutritional requirements.111,112 Low-fat enteral formulas and those with fat provided by MCTs are more readily absorbed than formulas that are high in long-chain triglycerides (e.g., corn oil, sunflower oil).
When oral intake is possible, small, frequent feedings of low-fat foods are least likely to cause discomfort,109 though the level of fat restriction should depend on the level of steatorrhea and abdominal pain the patient experiences.107 For patients with chronic pancreatitis, pancreatic enzyme replacement therapy may be indicated.107 Guidelines for the treatment of diabetes (discussed later) are appropriate for the care of the person with glucose intolerance or diabetes related to pancreatitis.
Nutrition and Endocrine Alterations
Nutrition Intervention in Endocrine Alterations
Nutrition Support and Blood Glucose Control
Patients with insulin-dependent diabetes mellitus (type 1 diabetes) or endocrine dysfunction caused by pancreatitis often have weight loss and malnutrition as a result of tissue catabolism because they cannot use dietary carbohydrates to meet energy needs. Although patients with type 2 diabetes are more likely to be overweight than underweight, they also may become malnourished as a result of chronic or acute infections, trauma, major surgery, or other illnesses.113 Nutrition support should not be neglected simply because a patient is obese, because PCM can develop in these patients. When a patient is not expected to be able to eat for at least 5 to 7 days or inadequate intake persists for that period, initiation of tube feedings or TPN is indicated. No disease process benefits from starvation, and development or progression of nutritional deficits may contribute to complications, such as pressure ulcers, pulmonary or urinary tract infections, and sepsis, which prolong hospitalization, increase the costs of care, and may even result in death.
Blood glucose control is especially important in the care of surgical patients. Poorly controlled diabetes reduces immune function by impairing granulocyte adherence, chemotaxis, and phagocytosis.114,115 In surveys of critically ill patients undergoing a variety of elective operations and coronary artery surgery,114 glucose levels of 206 to 220 mg/dL or higher during the first 24 to 36 postoperative hours were associated with higher rates of nosocomial infection than lower glucose levels.114,116 To maintain tight control of blood glucose, glucose levels are monitored regularly, usually several times a day, until the patient is stable. Patients unable to tolerate oral diets or enteral feeding may require TPN to meet nutritional requirements during acute illness.117 Regular insulin added to the solution is a common method of managing hyperglycemia in the patient receiving TPN. The dosage required may be larger than the patient’s usual dose because some of the insulin adheres to glass bottles and plastic bags or administration sets. Multiple injections or, preferably, a continuous infusion of regular insulin may be used to maintain tight control of blood glucose in the enterally fed patient.118 Although further study is needed, the following glucose goals are recommended: 80 mg/dL to 120 mg/dL in critically ill patients in the critical care unit and 100 mg/dL to 150 mg/dL for stable patients on the wards.117
In patients receiving enteral tube feedings, the postpyloric route (through a nasoduodenal, nasojejunal, or jejunostomy tube) may be the most effective, because gastroparesis may limit tolerance of intragastric tube feedings.119 Postpyloric feedings are given continuously because dumping syndrome and poor absorption may occur if feedings are given rapidly into the small bowel. Continuous enteral infusions are associated with improved control of blood glucose. Fiber-enriched formulas may slow the absorption of the carbohydrate, producing a more delayed and sustained glycemic response. Most standard formulas contain balanced proportions of carbohydrate, protein, and fats appropriate for diabetic patients. Specialized diabetic formulas have not shown improved outcomes compared with standard formulas.118
Severe Vomiting or Diarrhea in the Patient with Type 1 Diabetes Mellitus
When insulin-dependent patients experience vomiting and diarrhea severe enough to interfere significantly with oral intake or result in excessive fluid and electrolyte losses, adequate carbohydrates and fluids must be supplied. Nausea and vomiting should be treated with antiemetic medication.119 Delayed gastric emptying is common in diabetes and may improve with administration of prokinetic agents.119 Small amounts of food or liquids taken every 15 to 20 minutes usually are the best tolerated by the patient with nausea and vomiting. Foods and beverages containing approximately 15 g of carbohydrate include cup of regular gelatin,
cup of custard,
cup of regular ginger ale,
cup of a regular soft drink, and
cup of orange or apple juice. Blood glucose levels should be monitored at least every 2 to 4 hours.
Nutrition Education in Diabetes
Optimal control of blood glucose in type 1 and type 2 diabetes is associated with a decreased risk of development of retinopathy, neuropathy, and other long-term complications.113 Self-monitoring of blood glucose is essential in maintaining diabetic control, and nutrition is considered the most critical component of diabetes care in achieving blood glucose goals.120 Meals are based on heart-healthy diet principles, according to which saturated fat and cholesterol are limited and protein accounts for 15% to 20% of total calories. Most carbohydrate foods should be whole grains, fruits, vegetables, and low-fat milk.121 Evidence-based medical nutrition therapy supports hospitals implementing a consistent carbohydrate meal plan for diabetic patients.122 The meal plan is based on the amount of carbohydrate that is consistent from meal to meal each day. Although exact calorie levels are not specified, a typical daily menu provides approximately 1500 to 2000 calories with a range of three to five carbohydrate foods at each meal, each containing 15 g of carbohydrate.121
Careful monitoring of dietary intake and blood glucose levels is essential during critical illness to meet nutritional needs and maintain glucose control. Avoidance of overfeeding limits hyperglycemia and associated complications. Insulin can be adjusted to maintain blood glucose control based on frequent monitoring. Intensive insulin therapy has been shown to reduce mortality rates for critically ill surgical patients.114 It is vital for the dietitian to work closely with the interdisciplinary team to determine feeding methods, appropriate enteral formulas, and the amounts of protein, lipid, and carbohydrate supplied in parenteral nutrition.122
Summary
Nutrient Metabolism
• The major purposes of metabolism of the energy-yielding nutrients are the production of energy and the formation and preservation of lean body mass.
Implications of Undernutrition for the Sick or Stressed Patient
• Malnutrition can be related to any essential nutrient or nutrients, but a serious type of malnutrition found frequently among hospitalized patients is protein-calorie malnutrition (PCM). It is usually the result of the combined effects of starvation and hypermetabolism.
• The hypermetabolic process results from increased catabolic changes caused by a stressful event.
• Malnutrition is associated with a variety of adverse outcomes: wound dehiscence, pressure ulcers, infections, respiratory failure requiring ventilation, longer hospital stays, and death.
Nursing Management of Nutrition Support
• Whenever possible, the enteral route is the preferred method of feeding because of lower cost, better maintenance of gut integrity, and decreased infection and hospital stay.
• Oral supplementation may be necessary for patients who can eat and have normal digestion and absorption.
• Early enteral nutrition (within the first 24 to 48 hours of critical illness) reduces septic complications and improves feeding tolerance in critically ill patients.
• It is essential to check tube placement before feedings and regularly throughout the course of enteral feedings.
• Continued oral care is provided during the course of the enteral feedings.
Nutrition and Cardiovascular Alterations
• In the early period after an MI, interventions are aimed at reducing angina, cardiac workload, and the risk of dysrhythmia; meal size, caffeine intake, and food temperatures are monitored closely.
• Interventions in heart failure are designed to reduce fluid retention, thereby reducing the preload.
• Calorie-dense foods and supplements are provided to the patient with cardiac cachexia.
Nutrition and Pulmonary Alterations
• Malnutrition has adverse effects on respiratory function, decreasing surfactant production, diaphragmatic mass, vital capacity, and immunocompetence.
• Because of anorexia, dyspnea, debilitation, or the need for ventilatory support, many patients require tube feeding or TPN.
• It is especially important to be alert to the risks of pulmonary aspiration in the patient with an artificial airway, keeping the hob elevated 45 degrees during feedings, unless contraindicated, and keeping the cuff of the artificial airway inflated during feedings.
Nutrition and Neurologic Alterations
• Patients with dysphagia or weakness of the swallowing musculature often experience the greatest difficulty in swallowing foods that are dry or thin liquids, such as water, which are difficult to control.
• Beverages may be thickened with commercial thickening products, infant cereal, or yogurt; fruit nectars may be better tolerated than fruit juices.
• Prompt use of nutrition support is important for patients with head injuries because head injuries cause marked catabolism, and patients undergo an inflammatory response and may be febrile, increasing the need for proteins and calories.
Nutrition and Renal Alterations
• The goal of intervention is to balance adequate calories, protein, vitamins, and minerals while avoiding excesses of proteins, fluid, electrolytes, and other nutrients with potential toxicities.
• Patients with acute kidney injury need adequate amounts of protein to avoid catabolism of body tissues.
• With the use of continuous peritoneal dialysis, hemofiltration, or hemodialysis, the fluid intake can be liberalized. This allows for more adequate nutrient delivery through oral, tube, or parenteral feedings.
• The need for several water-soluble vitamins and trace minerals is increased in dialysis patients because these nutrients are small enough to pass through the dialysis filter.
Nutrition and Gastrointestinal Alterations
• Ascites and edema in the patient with liver failure have many causes. There is decreased colloid osmotic pressure in the plasma because of reduced production of albumin and other plasma proteins; increased portal pressure caused by obstruction; and renal sodium retention from secondary hyperaldosteronism.
• Causes of malnutrition in liver failure are complex and related to decreased intake, malabsorption, maldigestion, and abnormal nutrient metabolism.
• In the patient with pancreatitis, concern that feeding may stimulate the production of digestive enzymes and perpetuate liver damage has led to the use of TPN and bowel rest.