35 Nontraumatic Intracerebral and Subarachnoid Hemorrhage
Intracerebral Hemorrhage
Spontaneous (nontraumatic) intracerebral hemorrhage (ICH) accounts for approximately 10% of all strokes in North America and about 20% to 30% in East Asia. It is associated with greater mortality and more severe neurologic deficits than any other stroke subtype.1–3 Nearly half of all patients die within the first 30 days; survivors often have significant residual disability.4
Pathophysiology
The pathophysiologic mechanisms of brain injury due to ICH are complex. The primary injury is one of local tissue destruction as rupture of a cerebral blood vessel introduces a sudden stream of blood into the brain parenchyma. In over one third of patients, continued bleeding or rebleeding results in hematoma enlargement and further mechanical injury within the first few hours after onset.5 The mass of the hematoma produces tissue shifts within the intracranial cavity.
Experimental models of ICH consistently suggest that ischemia is an important part of the pathophysiology of ICH.6,7 In clinical studies, peri-clot and ipsilateral hemispheric hypoperfusion have been demonstrated,8–10 but the hypoperfusion does not appear to represent ischemia.11,12 Positron emission tomography (PET) studies in humans performed 5 to 22 hours after symptom onset showed that perihematomal cerebral metabolism was reduced to a greater degree than cerebral blood flow (CBF), suggesting that the hypoperfusion reflects reduced metabolic demand of the damaged tissue surrounding the hematoma rather than ongoing ischemia.12 Magnetic resonance imaging (MRI) studies within 6 hours after symptom onset demonstrate hypoperfusion without restricted diffusion, findings that are inconsistent with ischemia.13
Cerebral edema has been demonstrated to occur within hours of experimental ICH, variably thought to result from the toxic effects of blood-derived enzymes, from increased osmotic pressure exerted by clot-derived serum proteins, or from ischemia.14–16 The presence, time course, and importance of edema formation in humans are debated, however. Signal changes on radiographic studies after ICH indicate increased water content in the area surrounding the clot, but the clinical and pathophysiologic significance of this is not known. Edema does not appear to contribute to early increases in mass effect17 and is not associated with worsened functional outcome or increased mortality.18,19
Causes and Risk Factors
The leading risk factor for ICH, occurring in over half of all cases, is chronic hypertension.20 Long-term adequate treatment of chronic hypertension significantly reduces this risk.21 Increasing age is another risk factor, with a doubling of the rate of hemorrhage with each decade of life until age 80, when the incidence plateaus at nearly 25 times that of the previous decade.22 In the United States, ICH is 2 to 3 times more common in African Americans and Hispanics than in Caucasians (incidence rates of 32, 35, and 10 to 15 per 100,000 population, respectively).23,24 The incidence in Asian countries is considerably greater (61 per 100,000 population).25
Low serum cholesterol has been implicated in a number of studies,26 and use of high-dose statins appears to increase the risk of ICH, particularly in those with prior history of ICH.27 The impact of smoking,28 alcohol abuse,29,30 and diabetes31,32 on the risk of ICH is disputed.
Hypertensive Hemorrhage
Hypertensive ICH predominantly occurs deep in the cerebral hemispheres, most often in the putamen33 (Figure 35-1). Other frequently involved sites include the thalamus, lobar white matter, cerebellum, and pons. The common link between these sites is that they are all supplied by small penetrating arteries,34 perpendicular branches directly off major arteries that are subject to high sheer stress and that have no collaterals. These features make them vulnerable to the effects of increased blood pressure. Chronic hypertension damages the tunica media, resulting in lipohyalinosis, fibrinoid necrosis, and microaneurysms (Charcot-Bouchard aneurysms). Although Charcot-Bouchard aneurysms have been demonstrated in the weakened vessel walls of patients with ICH, their pathogenetic role in vascular rupture is uncertain.35 The occurrence of ICH in an atypical location, in multiple locations, or in association with subarachnoid hemorrhage raises the suspicion of a non-hypertensive etiology, such as a cerebral vascular anomaly, blood dyscrasia, or trauma.
Intracranial Aneurysms and Vascular Malformations
Approximately half of intracranial AVMs in adults present with hemorrhage.36 In 60% of cases, the hemorrhage is parenchymal, involving virtually any location within the cerebrum, brainstem, or cerebellum.37 The majority of AVMs become symptomatic by age 40; thus hemorrhage due to AVM occurs in a younger population than that due to aneurysms or hypertension. Multiple calcified vascular channels may be seen within the hematoma on CT scan, suggesting the presence of an AVM. MRI and four-vessel cerebral angiography are useful adjuncts in the diagnosis of these lesions.
Other Causes
Cerebral amyloid angiopathy (CAA) is an important cause of predominantly lobar, often recurrent, ICH in the elderly. Histopathologic studies in CAA demonstrate the deposition of beta-amyloid protein in the media and adventitia of small meningeal and cortical vessels; deposition in the typical sites for hypertensive hemorrhage is rare but has been reported in the cerebellum.38 The prevalence of amyloid in cerebral vessels increases dramatically with age39,40 and may partially account for the exponential rise in the risk for ICH with increasing age. There is an overrepresentation of the apolipoprotein E ε2 and ε4 genotypes in CAA-related hemorrhage, and these alleles are associated with an earlier age of onset of first hemorrhage and a higher risk of early recurrence.41,42 Although neuropathologic examination remains the only means of definitively diagnosing CAA, the presence of multiple or recurrent lobar ICH (including asymptomatic microhemorrhages detected on gradient-echo MRI) in individuals 55 years or older without other known causes of hemorrhage strongly suggests the diagnosis.43 Recent PET studies with 11C-Pittsburgh compound B (PIB) suggest an occipital predominant increase in amyloid in such patients.44 Neuropathologic correlation remains to be demonstrated, but PIB-PET appears to be a promising tool for in vivo diagnosis of CAA.
Hematologic causes of ICH include the use of antithrombotic and thrombolytic agents as well as systemic disease (e.g., thrombocytopenia, leukemia, and hepatic and renal failure) and congenital or acquired factor deficiencies. The incidence of oral anticoagulant (OAC)-associated ICH has been increasing in parallel with the increased use of warfarin following pivotal trials in atrial fibrillation in the 1990s. A recent population-based study in the greater Cincinnati area identified a fivefold increased incidence of OAC-associated ICH between 1988 and 1999, such that this condition now accounts for 17% of all ICH cases.45 The incidence is anticipated to rise further in the coming years as the population ages. Although the risk of ICH is greater in the setting of very elevated international normalized ratio (INR), a significant number of hemorrhages occur when the INR is within the therapeutic range.46 Hematoma expansion may be more common in OAC-associated ICH and occur over a longer time frame because of persistent coagulopathy, contributing to the doubling of mortality rate compared to spontaneous ICH.47
The relationship between antiplatelet agent use and hematoma size, hematoma expansion, and outcome in ICH are active areas of investigation. Most studies suggest that antiplatelet use at ICH onset is not associated with larger hematoma size, hematoma growth, or poor clinical outcome48; however, an association has been demonstrated between reduced platelet activity and hematoma growth and poor outcome.49 Whether platelet transfusion is beneficial in this situation is unknown.
Hemorrhage from an underlying neoplasm is rare but occasionally occurs with malignant primary CNS tumors such as glioblastoma multiforme and lymphoma and with metastatic tumors such as melanoma, choriocarcinoma, renal cell carcinoma, and bronchogenic carcinoma.50 Benign tumors are almost never associated with ICH.
ICH may also occur in association with infection (e.g., infiltration of vessel wall by fungal organisms,51 necrotizing hemorrhagic encephalitis with herpes simplex,52 vasculitis,53 venous sinus occlusion,54 in a delayed fashion after head trauma,55 following reperfusion (e.g., after carotid endarterectomy or acute thrombolysis),56 and with the use of various drugs, particularly sympathomimetics (e.g., cocaine, amphetamines, pseudoephedrine, and phenylpropanolamine).57 Finally, some degree of hemorrhagic transformation of acute cerebral infarcts is common,58 though symptomatic ICH in this setting is rare in the absence of anticoagulation or thrombolytic therapy.
Clinical Features
The clinical presentation of ICH is often indistinguishable from that of ischemic stroke but more commonly includes altered level of consciousness, headache, and vomiting, reflecting the presence of increased intracranial pressure (ICP).33 Blood pressure is elevated in the majority of patients (see later discussion). Seizures occur in nearly one-third of patients at onset or within the first few days, particularly in those with lobar hemorrhages or underlying vascular or neoplastic lesions, and may be purely electrographic.59 Symptoms are maximal at onset or develop over minutes to hours. Neurologic deterioration within 48 hours after hospital admission has been reported to occur in 22% of patients with ICH.60 The cause for clinical worsening is not always evident, but it is predicted by clinical and biological markers of inflammation on admission and commonly associated with increased hematoma size and intraventricular bleeding.
Diagnostic Studies
Noncontrast computed tomography (CT) scanning has been the traditional gold standard for diagnosis of acute ICH. The typical CT appearance of an acute hematoma consists of a well-defined area of increased density surrounded by a rim of decreased density. Over time, the borders of both the high- and low-attenuation regions become increasingly indistinct such that the hematoma is isodense with adjacent brain parenchyma by 2 to 6 weeks.61 Peripheral contrast enhancement can often be seen at this time.62 By 2 to 6 months, there may be no CT evidence of previous hemorrhage or there may be an area of hypodensity or a slit-like scar.63
Recent studies have suggested that MRI has high sensitivity and specificity for the diagnosis of acute ICH.64–66 These studies have been criticized, however, for major methodological limitations including the basing of sensitivity estimates on only a small fraction of patients investigated, lack of CT comparator in many patients, and both incorporation and spectrum bias (highly selected patient sample) that may have overestimated diagnostic accuracy of MRI.67 In addition, MRI may not be feasible in a substantial number of patients with acute ICH because of impaired consciousness, hemodynamic compromise, or vomiting.68 The benefits of MRI over CT are its superior performance in the identification of associated vascular malformations, greater accuracy in determining the approximate age of a hematoma (because each hemoglobin oxidation state during evolution of the hematoma produces a predictable pattern of MR signal intensity),69 and its utility in demonstrating the iron-containing deposits of previous asymptomatic hemorrhages.70
Angiography is useful in evaluating the cause of ICH if an underlying aneurysm or vascular malformation is suspected, but the yield of such studies is extremely low when the patient has chronic hypertension and the hemorrhage is in one of the typical sites associated with hypertensive hemorrhage.71 Multidetector CT angiography is evolving as an alternative to conventional angiography. In a retrospective review of 623 patients, multidetector CT angiography identified a vascular etiology for ICH in 91 (15%), with a sensitivity of 96% and a specificity of 99%. The yield was higher in patients who were younger than 46 (47%), had lobar (20%) or infratentorial (16%) ICH locations, had lobar hemorrhages with intraventricular extension (25%), and had neither hypertension nor impaired coagulation (33%).72 In another study of 78 patients, CT angiography identified all but one of the 22 lesions seen on conventional angiography, with a sensitivity of 96% and a specificity of 100%.73
Multidetector CT angiography can also be used to identify the presence of active contrast extravasation into the hematoma, an indicator of active hemorrhage. Termed the “spot sign,” these foci of intralesional enhancement are seen in up to one third of patients with acute ICH74 and are associated with an increased risk of hematoma expansion, in-hospital mortality, and poor outcome in survivors.75
Treatment
Initial Stabilization
Acute ICH is a medical emergency requiring considerable attention to airway and respiratory management, hemodynamic status, and correction of any underlying coagulopathy. As many as half of all patients with ICH undergo mechanical ventilation.76 Blood pressure is often elevated at presentation, sometimes markedly so. Finally, given the frequency of hematoma enlargement over the first few hours, aggressive correction of coagulopathies might be helpful.
Airway and Respiratory Management
Initial airway management includes proper positioning, frequent suctioning, and placement of an oral or nasal airway. Frequent assessments for sonorous respiration, inability to manage oral secretions, or decreased oxygen saturation are necessary. If conservative measures are ineffective, intubation may be necessary. Intubation of patients with ICH requires adequate sedation and jaw relaxation as well as prevention of elevation of ICP. Several factors may conspire to raise ICP during intubation: hypoxia, hypercarbia, and direct tracheal stimulation causing systemic and intracranial hypertension. Intravenous (IV) lidocaine (1-1.5 mg/kg) has been recommended to block this response,77 although data supporting its use are lacking.78 Short-acting IV anesthetic agents (thiopental, 1-5 mg/kg; or etomidate, 0.1-0.5 mg/kg) also block this response79 and additionally suppress brain metabolic rate,80 theoretically improving tolerance of a transient fall in cerebral perfusion pressure (CPP) should it occur. Etomidate is generally preferred over thiopental, since it is less likely to lower blood pressure. Paralytic agents are usually unnecessary but, if needed, short-acting agents should be used.
Hemodynamics
Arterial blood pressure is elevated on admission in the majority of patients with ICH, even in the absence of a history of hypertension.33 Mean arterial pressure (MAP) is greater than 120 mm Hg in over two-thirds of patients and greater than 140 mm Hg in over one-third.81 Although this acute increase in blood pressure is often implicated as the cause of the hemorrhage, it may simply be a reflection of chronic hypertension, the brain’s attempt to maintain CPP in response to the sudden increase in ICP, pain and anxiety, and sympathetic activation. Even without pharmacologic intervention, blood pressure tends to decline to premorbid levels during the first 7 to 10 days after hemorrhage.82
There is substantial controversy over if and when to lower blood pressure after acute ICH and how aggressive any intervention should be.83 Proponents of rapid treatment of acute hypertension argue that high blood pressure may predispose to hematoma enlargement and may exacerbate vasogenic edema by increasing capillary hydrostatic pressure, especially in areas with a damaged blood-brain barrier. Yet, an association between hypertension and edema has never been demonstrated, and data on the effect of hypertension on hematoma enlargement have been inconsistent.84,85 Another potential reason to lower blood pressure is that hypertension during the acute phase of ICH has been shown to correlate with a poor prognosis in some studies.86,87 One compelling reason to consider lowering blood pressure in ICH patients with moderate to severe hypertension is the potential for end-organ damage. Such patients are at risk for systemic complications of elevated blood pressure, including myocardial ischemia, congestive heart failure, and acute renal failure.
The major argument against the treatment of elevated blood pressure is that lowering blood pressure might exacerbate ischemic damage in the tissue surrounding the hematoma by impairing blood flow.88 Chronic hypertension shifts the cerebral autoregulatory curve to the right such that a higher CPP is required to maintain adequate CBF.89,90 Lowering the blood pressure to “normal” levels in these patients might thus lead to inadequate CBF. Similarly, since CPP is equal to the difference between MAP and ICP, lowering blood pressure may reduce CPP below the autoregulatory limit in patients in whom ICP is elevated due to a large space-occupying clot or hydrocephalus.
Seeking to address the issue of whether lowering blood pressure produces cerebral ischemia in acute ICH, several studies of CBF autoregulation in patients with recent ICH and elevated blood pressure (MAP > 130-140 mm Hg) have been carried out.91–93 Taken together, these studies demonstrate that regional and global autoregulation are preserved after ICH down to a lower MAP limit that averages 110 mm Hg or about 80% of the admission MAP.
These observations set the stage for the INTERACT trial,94 a prospective trial of blood pressure management beginning within 6 hours of symptom onset in 404 patients with spontaneous ICH and elevated systolic blood pressure (150-220 mm Hg). Patients were randomized to an early intensive blood pressure–lowering strategy that targeted a reduction in systolic blood pressure to below 140 mm Hg within 1 hour, or control, with a target systolic pressure of less than 180 mm Hg. The primary efficacy endpoint was hematoma growth at 24 hours. After the first hour of treatment, mean blood pressure was 13.3 mm Hg lower (95% confidence interval [CI] 8.9-17.6 mm Hg; P <0.0001) in the intensive blood pressure–lowering group. Hematoma growth at 24 hours was 36.3% in the control group and 13.7% in the intensive treatment group, a 22.6% difference (95% CI 0.6%-44.5%; P=0.04). After adjusting for initial hematoma volume and time from onset to CT, median hematoma growth at 24 hours differed by 1.7 mL (95% CI 0.5-3.9, P=0.13). Intensive blood pressure–lowering treatment did not increase the risk of adverse events or improve 90-day clinical outcome. A much larger follow-on trial is currently underway.
Prevention of Hemorrhage Extension
Because hemorrhage extension occurs within the first few hours after symptom onset in about one-third of patients (Figure 35-2), it seems appropriate that any coagulopathy should be corrected as rapidly as possible. Patients taking warfarin should receive IV vitamin K and enough fresh frozen plasma (FFP) to normalize the coagulation profile. Prothrombin complex concentrate may be a useful alternative. Care must be taken not to precipitate congestive heart failure, however, and diuretics may be required. Additionally there is risk of transfusion-related acute lung injury with the administration of fresh frozen plasma, which can complicate the process considerably. Correcting coagulopathy associated with thrombolytic-induced ICH is discussed later.
Even in those patients without coagulopathy, promoting early hemostasis might limit ongoing bleeding and decrease hematoma volume. Factor VIIa is a coagulation factor that interacts with tissue factor exposed in the wall of a damaged blood vessel to drive a burst of thrombin that initiates platelet aggregation and accelerates formation of a stable fibrin clot. A phase IIb placebo-controlled dose-ranging proof-of-concept study found that treatment with recombinant factor VIIa (rFVIIa) given as a single IV bolus within 4 hours of ICH onset decreased hematoma growth and improved clinical outcome despite a small increase in thromboembolic events. A much larger phase III trial comparing placebo to 20 and 80 µg/kg of rFVIIa followed. This study confirmed the ability of rFVIIa to reduce hematoma growth; however, at 90 days there was no difference in clinical outcome.95 A post hoc exploratory analysis suggested that a subgroup of younger patients who present earlier and have no significant intraventricular hemorrhage might benefit from rFVIIa, but this has not been tested.96
Intraventricular Hemorrhage and Hydrocephalus
In approximately 40% of patients with ICH, blood extends into the ventricular system (intraventricular hemorrhage [IVH]).97 Mortality in these patients is high.98,99 IVH may contribute to poor outcome by blocking cerebrospinal fluid (CSF) pathways, with resultant hydrocephalus and increased ICP. In addition, intraventricular blood and/or its breakdown products may exert direct chemical irritative effects on periventricular structures. Hydrocephalus may develop after ICH either in association with IVH or because of direct mass effect on a ventricle (e.g., on the third ventricle with a thalamic hemorrhage) (Figure 35-3). External ventricular drainage (ventriculostomy) is frequently used to treat hydrocephalus and IVH, but its efficacy has never been established. Ventriculostomy in the setting of IVH is difficult to manage because the catheter frequently becomes obstructed with thrombus, interrupting drainage and raising ICP. Flushing the system helps remove thrombus from the catheter but increases the risk of ventriculitis. Recently, investigators have attempted to facilitate removal of blood from the ventricles via direct intraventricular administration of thrombolytic agents. Preliminary studies have been promising,100 and a multicenter randomized trial is currently underway.
Intracranial Hypertension
The incidence, impact, and appropriate management of intracranial hypertension in ICH are not well understood. Factors likely to contribute to elevated ICP in this population include large hematoma size, minimal degree of underlying cerebral atrophy, hydrocephalus, and edema, but the true incidence of intracranial hypertension is unclear, since routine ICP monitoring is not performed. Because the hematoma is localized and the increase in volume it produces can be compensated for to some degree by reduction in the size of the ventricles and subarachnoid space, a global increase in ICP may not be seen unless the hemorrhage is very large or is associated with marked hydrocephalus. However, mass effect from the hematoma and local tissue shifts can compress the brainstem or result in herniation in the absence of a global increase in ICP.101,102 Thus the utility of ICP monitoring has never been established.
Elevated ICP is often treated with osmotic agents (mannitol, hypertonic saline) and, if the ventricles are enlarged, CSF drainage. A recent case series suggested that rapid reversal of clinical transtentorial herniation (decreased level of consciousness and dilated pupil) with hyperventilation and osmotic agents improved long-term outcome.103 There are only a few small clinical trials of osmotic agents in ICH, which do not provide sufficient data to support their routine use.104 The best available data on corticosteroids in ICH indicate that they do not provide any benefit and increase the rate of complications.105
Surgical Evacuation
The rationale for surgical evacuation of a hematoma is that reducing mass effect and removing neurotoxic clot constituents should minimize injury to adjacent brain tissue and hence improve outcome. Unfortunately, several randomized controlled trials of surgery for supratentorial ICH dating back to 1961 have all failed to show a benefit of the intervention.106–109 A meta-analysis of three of these trials reported that patients undergoing surgical evacuation via open craniotomy had a higher rate of death or dependency at 6 months compared to those managed medically (83% versus 70%).110 Criticisms of these trials are that the surgical techniques used were outdated, patient selection was inadequate, and surgery was delayed too long.
Because open craniotomy is complicated by tissue damage sustained during the approach to the hematoma, a variety of new techniques for clot removal have been proposed, including an Archimedes screw, ultrasonic aspirator, modified endoscope, modified nucleotome, double track aspirator, intraoperative CT monitoring, and instillation of thrombolytics. However, the recurrence of bleeding due to the loss of tamponade effect on adjacent tissue that occurs in 10% of patients treated with open craniotomy remains an issue with the newer techniques. In addition, because the newer techniques involve limited surgical exposure, concern exists that rebleeding will be more difficult to control than with open craniotomy. One study comparing endoscopic aspiration to medical management found a better outcome in the surgical group (74% death or disability compared to 90%), but the benefit was limited to patients with lobar hematomas.111 Three studies addressed the feasibility of early craniotomy for ICH. In one,112 34 patients were treated within 12 hours of ICH. Mortality was 18% in the surgical group and 23% in the medical group. In another study,113 20 patients were randomized, with a median time to surgery of 8.5 hours from onset. Good outcome (Glasgow Outcome Scale score > 3) was 56% with surgery and 36% in the medically treated group (P = NS). The third, a study of ultra-early surgery (<4 hours), found a disturbingly high rate of postoperative rebleeding.114
A lack of benefit of surgery in ICH was also shown in a recently completed multicenter trial in which 1033 patients were randomized within 72 hours of ICH onset to surgical hematoma evacuation (open craniotomy or stereotactic aspiration, at surgeon’s discretion) or initial conservative management. Favorable outcome occurred in 26.1% in the surgery group and 23.8% in the initial conservative treatment group, a nonsignificant difference (odds ratio 0.89; 95% CI 0.66-1.19). There was also no difference in mortality (surgery 62.6% versus conservative treatment 63.7%). Subgroup analysis suggested a possible benefit of surgery in patients with superficial hematomas (less than 1 cm from cortical surface).115 A trial comparing surgical and medical management of superficial hematomas is currently underway.
Cerebellar hemorrhages were excluded from the randomized trials of surgery, but nonrandomized case series report good outcomes for surgically treated patients with cerebellar hemorrhages that are large or associated with brainstem compression or obstruction of the fourth ventricle. Recommended criteria for when to evacuate a cerebellar hematoma have thus included diminished level of consciousness, large size of the hematoma (>3 cm3), midline location, compression of basal cisterns and/or brainstem, and presence of hydrocephalus (Figure 35-4).116–118 Patient selection is important as many patients with smaller hemorrhages do well with medical management.119
Management of Thrombolytic-Induced Ich
Associated with considerable morbidity and mortality, symptomatic ICH is a feared complication of thrombolytic therapy. Symptomatic ICH occurs after thrombolytic treatment of acute ischemic stroke in approximately 6% of patients.120 It is substantially less common after thrombolytic treatment of extracerebral thrombosis (myocardial infarction, pulmonary embolism, deep venous thrombosis, and arterial and graft occlusion)121 but results in a similarly poor outcome.122 Factors that increase the risk of symptomatic ICH include intraarterial versus IV route of administration, early ischemic changes on pretreatment CT, greater symptom severity, and elevated serum glucose or history of diabetes mellitus.123,124
In the setting of thrombolytic therapy, any new neurologic deficit, especially with a decline in consciousness, should be assumed to be due to hemorrhage. Management of a suspected ICH should begin with stopping the thrombolytic infusion, reassessing the patient’s airway, and obtaining an emergent CT scan. Blood studies (prothrombin time, partial thromboplastin time, thrombin, and fibrinogen levels) should be performed to assess fibrinolytic state. Preparations for giving FFP, cryoprecipitate, and platelets should be initiated at the first suspicion of hemorrhage so that they will be ready if needed; however, no reliable data are available to guide the choice of blood product. The NINDS rt-PA study125 stipulated 6 to 8 units of cryoprecipitate or FFP and 6 to 8 units of platelets, but only rarely was this amount of blood product given to an individual patient during the study. Although neurosurgical consultation was frequently obtained in patients with symptomatic ICH in the NINDS trial, only one patient in the study underwent surgery, and that patient died.
Management of Seizures
Although seizures may theoretically exacerbate ICH, they have not been demonstrated to alter outcome. Prophylactic anticonvulsants may reduce the risk of early seizures in patients with lobar ICH but do not affect the risk of developing epilepsy.126 Thus reasonable approaches include either a brief period of prophylaxis or treating only if seizures occur. As for any hospitalized patient, the treatment of clinical seizures typically begins with an IV benzodiazepine such as lorazepam followed by an IV agent such as fosphenytoin.
Supportive Care
Patients with ICH are prone to the same medical complications seen in patients with ischemic stroke, including fever, deep venous thrombosis (DVT), pulmonary embolism, and pneumonia.127,128 Given the association between fever and worsened outcome in experimental models of brain injury, it is reasonable for antipyretic medications to be administered in febrile patients with ICH. The use of pneumatic sequential compression devices and elastic stockings has been shown to significantly decrease the incidence of DVT in patients with acute ICH relative to elastic stockings alone.129 Subcutaneous heparin at a dose of 5000 U three times daily when initiated on day 2 after hemorrhage has been shown to significantly reduce the frequency of DVT relative to treatment begun on day 4 or 10, with no concomitant increase in hematoma expansion.130 In another study, subcutaneous enoxaparin (40 mg daily) initiated at 48 hours after ICH was also safe. A benefit of enoxaparin over compression stockings could not been detected because of the low incidence of DVT in both treatment groups.131
Prognostic Factors and Causes Of Mortality
Mortality following ICH is high (25%-50%), with over half of the deaths occurring in the first 48 hours. Although patients who have small hemorrhages and mild deficits may recover completely, the majority of ICH survivors have significant residual disability.4,132,133 A variety of clinical, laboratory, and radiographic predictors of poor outcome have been identified, the most consistent being impaired level of consciousness and large hematoma size on admission. Other predictive clinical features variably include increasing age, elevated admission blood pressure on admission, rapid decline in blood pressure over the first 24 hours, history of diabetes, antecedent OAC use, male gender, and in-hospital neurologic deterioration. Laboratory parameters identified in at least one study include hyperglycemia, elevated troponin level, elevated plasma S100B level, elevated plasma D-dimer level, elevated INR, low serum cholesterol and triglyceride levels, and apolipoprotein E ε2 or ε4 allele. Radiographic features include infratentorial hematoma location, intraventricular spread of blood, midline shift, hydrocephalus, hematoma growth, and presence of the spot sign on CT angiography. A number of prognostic models of varying complexity have been developed to allow risk stratification upon presentation with ICH. One easy to use model is the ICH score,134 which is based on point assignments for Glasgow Coma Scale score, ICH volume, presence of intraventricular hemorrhage, infratentorial location, and patient age and has been validated to accurately predict 30-day mortality. It has been demonstrated, however, that withdrawal of support in patients felt likely to have a poor outcome biases predictive models in ICH and negates the predictive value of all other variables.135 Thus, the most frequent cause of death after ICH is withdrawal of care, followed by early (within 48 hours) transtentorial herniation with progression to brain death. Medical complications of immobility (pulmonary embolism, pneumonia, sepsis) account for most of the other deaths.133 For survivors of ICH, the risk of recurrent stroke is approximately 4% per year. Recurrent ICH occurs about twice as often as ischemic stroke, especially in those with previous lobar hemorrhage.136
Subarachnoid Hemorrhage
Although it is the least common form of stroke, subarachnoid hemorrhage (SAH) has great impact on its sufferers. One-quarter of patients die before reaching medical attention,137 and because of the consequences of secondary insults—rebleeding, hydrocephalus, and delayed ischemia due to vasospasm—more than half of those that reach medical attention either die or are left with neurologic deficits.
Pathophysiology
In SAH, the primary site of bleeding is within the subarachnoid space, but may also involve hemorrhage into the brain parenchyma, ventricular system, or subdural space. Rupture of an intracranial saccular aneurysm (Figure 35-5) is by far the most common cause of spontaneous SAH. Saccular or berry aneurysms are small, rounded protrusions of the arterial wall occurring predominantly at bifurcations of the large arteries of the circle of Willis at the base of the brain. The most common sites of ruptured aneurysms are the distal internal carotid artery and its posterior communicating artery junction (41%); anterior communicating artery/anterior cerebral artery (34%); middle cerebral artery (20%); and vertebrobasilar arteries (4%).138 About 20% of patients have multiple aneurysms.
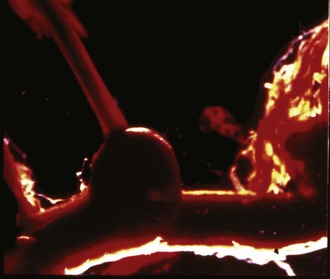
Figure 35-5 Autopsy specimen of intracranial aneurysm filled with pressurized blood to simulate subarachnoid hemorrhage.
The pathogenesis of aneurysms remains controversial, especially in regard to the relative important of developmental versus acquired factors. Proponents of the congenital theory suggest that aneurysms arise at sites of faulty fusion between muscular segments within the arterial wall. Supporters of the acquired-degenerative theory focus on the role of vascular damage caused by hemodynamic stress.139 The third possibility is that aneurysms develop at sites harboring congenital defects with superimposed degenerative changes.
Causes and Risk Factors
Genetic conditions that predispose to aneurysm formation include polycystic kidney disease, connective tissue disorders, and coarctation of the aorta. Recently it has become clear that in some patient populations, genetic factors play a role in aneurysm formation, without other associated conditions.140 There is a familial form as well.141 Other types of aneurysms that less commonly cause SAH include atherosclerotic, mycotic, and traumatic aneurysms.
The risk of SAH increases with age, peaking at 55 to 60 years. There is a slight male predominance in younger age groups and a slight female predominance among older patients.142 Potentially reversible risk factors for SAH include cigarette smoking, oral contraceptive use, alcohol abuse, and hypertension.143 Prospective cohort studies have reported a relative risk of SAH as high as 5.7 for female and 4.7 for male smokers,144