CHAPTER 348 Neurovascular Imaging
Moniz performed the first cerebral angiogram in 1927.1,2 Since that time, neurovascular imaging has undergone rapid evolution. The introduction of computed tomography (CT) and magnetic resonance imaging (MRI) offered viable noninvasive means of imaging the neurovascular tree. More recently, improvements in technology and technique have enabled the acquisition of direct and indirect hemodynamic measurements and functional and physiologic data, as well as visualization of intravascular pathology.
Computed Tomography–Based Techniques
Since the advent of CT in the 1970s, serial advances in imaging technique and computer software have provided increasingly detailed imaging of cerebrovascular anatomy, as well as the neurophysiologic properties of the brain in both the normal and pathologic states. For a comprehensive review of the available literature, please see the American Heart Association scientific statement on guidelines and recommendations for perfusion imaging in cerebral ischemia.3
Computed Tomographic Angiography
Technique
After the initial acquisition of non–contrast-enhanced head CT, 100 to 120 mL of nonionic intravenous contrast agent is administered at a rate of 3 to 4 mL/sec. A scanning delay is built in based on either detection of contrast material within the aortic arch or an empirical delay of 25 seconds. The area of the aortic arch to the circle of Willis is then imaged in 2.5-mm-thick slices. Postprocessing is generally performed at a computer workstation to generate reformatted and three-dimensional (3D) maximum intensity projection images.3–5
Clinical Applications
Cerebral Aneurysm
One of the most clinically useful applications of CTA is detection (Fig. 348-1A and B) and follow-up of cerebral aneurysms. Although digital subtraction angiography (DSA) has traditionally been the “gold standard” for detection of cerebral aneurysms, CTA has proved to have a sensitivity of greater than 90% for detection of aneurysms less than 2 mm in diameter.4–6 Because of the availability and speed of CTA, it has become the first-line imaging choice for subarachnoid hemorrhage (SAH) in many institutions. However, negative CTA findings necessitate subsequent DSA for evaluation of possible small aneurysms.
Because DSA is invasive and expensive, noninvasive modalities such as CTA are used to monitor aneurysms after treatment. CTA has been shown to be feasible for monitoring coiled aneurysms,6 but clipped aneurysms produce too much artifact to be well visualized on CTA, so specialized protocols have been developed to optimize the visualization of clipped aneurysms with CTA. Brown and coauthors reported on a technique in which CTA is performed with the patient’s head tilted so that the axial plane through the aneurysm clip projects away from the remainder of the circle of Willis. In their hands, this technique, when combined with other artifact-reducing methods (i.e., thin collimation), provides improved resolution of previously clipped aneurysms and surrounding structures.7
Acute Ischemia
The speed and ease of CTA have made it part of the stroke protocol at many institutions. Excellent views of the carotid (Fig. 348-2) and vertebral arteries in the neck can be inspected for evidence of stenosis, dissection, or thrombus. In addition, the primary intracerebral branches (i.e., the M1 division of the middle cerebral artery) may also be well visualized (see Fig. 348-1) and may confirm the vascular territory of the infarct and provide guidance for subsequent intra-arterial chemical or mechanical thrombolysis.
Chronic Ischemia/Carotid Stenosis
CTA is increasingly being used as a stand-alone diagnostic test to identify carotid stenosis (see Fig. 348-2). Because it is noninvasive, CTA is frequently preferred over DSA for outpatients or for those who cannot otherwise tolerate DSA.
Other
Another major advantage of CTA over DSA or MRA is that it provides excellent visualization of bony anatomy as it relates to vascular structures. For example, many rely on visualizing the optic strut on CTA to determine whether an ophthalmic aneurysm is intradural or extradural.8 CTA can also provide visualization of intraluminal thrombus.
Despite improvements, CTA does not always provide the same anatomic detail as either MRI or DSA. Information about flow rates or collateralization is not available on standard CTA sequences. As discussed later, CT perfusion can provide reliable relative cerebral blood flow (CBF) data.9
Computed Tomographic Perfusion
There have been attempts to use CT scans to provide functional and physiologic data since its advent in the 1970s. Only recently, however, have technologic advances allowed CT perfusion to be performed in timely fashion on standard helical scanners, which has consequently made it part of the stroke protocol at many institutions.10–13
Technique
The most commonly used technique is called dynamic contrast-enhanced CT. After the acquisition of unenhanced images on a helical scanner, two to four sections are chosen for analysis based on the non–contrast-enhanced CT scans. The area of the basal ganglia is frequently used as one of these sections because it includes vascular territories from the anterior, middle, and posterior cerebral arteries. In this technique, the first pass of an iodinated contrast bolus through the cerebral circulation is monitored. The contrast material causes a transient increase in attenuation that is used to generate time-attenuation curves. Mathematical modeling and deconvolution analysis are then used to generate the key measurements in the identification of ischemic and infarcted brain tissue.14 Perfused blood volume mapping is a separate technique that requires only subtraction of the unenhanced CT images from subsequent CTA images to obtain maps of the blood volume present. In contrast to the dynamic contrast-enhanced technique, perfused blood volume mapping can image the entire brain volume. However, it cannot evaluate CBF or mean transit time (MTT) and thus cannot be used to identify a stroke penumbra and consequently is used infrequently.3
Clinical Applications
Acute Stroke
The key benefit in using CT perfusion is its ability to determine cerebral blood volume (CBV), CBF, MTT, and time to peak enhancement. These measurements are most useful for identification of the stroke “penumbra” of ischemic but not yet infarcted brain tissue that can potentially be saved by either intravenous or intra-arterial thrombolysis. Srinivasan and colleagues noted that “the clinical application of CT perfusion imaging in acute stroke is based on the hypothesis that the penumbra shows either (1) increased MTT with moderately decreased CBF (>60%) and normal or increased CBV (80% to 100% or higher) secondary to autoregulatory mechanisms or (2) increased MTT with markedly reduced CBF (>30%) and moderately reduced CBV (>60%), whereas infarcted tissue shows severely decreased CBF (<30%) and CBV (<40%) with increased MTT.”5 Complex deconvolution algorithms can then be used to produce color maps on which areas of a potential penumbra can quickly be identified. A large penumbra may prompt further attempts at clot lysis or retrieval, whereas a small or absent penumbra may be evidence that any new intervention will just incur new risks without any additional advantage in preserving brain tissue.
CT perfusion has been shown to be comparable to Xe-CT for evaluation of regional CBF.15 However, CT perfusion is faster and easier to use. Helical/spiral CT scanners are readily available in most institutions, and CT perfusion can usually be combined with CTA to provide data about the site of the obstructed vessel. Images can be acquired and easily conceptualized maps generated with enough speed to be of use in an acute stroke protocol.14,16
Early reports suggested that CTA with or without CT perfusion may offer a noninvasive way of evaluating vasospasm.17,18 A study of CTA alone had a sensitivity and specificity of 86.8% and 96.8%, respectively, for mild to moderate vasospasm and 76.5% and 99.5%, respectively, for severe vasospasm, whereas CT perfusion had a sensitivity and specificity of 20% and 100%, respectively, for mild to moderate vasospasm and 90% and 100%, respectively, for severe vasospasm.18 This and other studies suggest that in the near future, the combination of these two modalities may provide high sensitivity and specificity for the noninvasive evaluation of vasospasm.19
Xenon-Enhanced Computed Tomography
Xe-CT has been in use for more than 30 years20,21 and relies on application of the Kety-Schmidt equation to calculate CBF. Xenon is a small, biologically inert and inhalable molecule that serves as the “contrast agent.” Its easily crosses the blood-brain barrier and has a short half-life (40 seconds).16 Thus, it is an ideal tracer for the evaluation of cerebral perfusion. Xenon causes x-ray attenuation similar to iodine and hence can quickly be identified with CT-based technology. By measuring the xenon tissue concentration, both arterial input and tissue uptake can be determined.21
Technique
A stable (nonradioactive) xenon and oxygen (28% Xe, 21% to 60% O2) mixture is inhaled over the course of 4.33 minutes. A nonenhanced CT scan is obtained, and four to six contiguous, 10-mm-thick levels are selected for study.16 Each level is scanned six times across the inhalation time, which requires exact table relocation. As xenon gas builds up in cerebral tissue, a mild sedative effect, respiratory depression, or nausea has been reported.16 These effects usually resolve over a few minutes after the procedure.
The CT scanner subtracts the baseline x-ray attenuation coefficients from those obtained during xenon accumulation. A modified Kety-Schmidt equation is used to calculate CBF for each voxel based on the rate of change in the concentration of xenon in the blood and brain and on the blood-brain partition coefficient for xenon. This yields flow data that are most accurate for regions of interest greater than 100 voxels (1 voxel = 10 mm3). These flow data can be overlaid on the standard CT image to create an anatomic quantitative flow map.3,22
Clinical Applications
Acute Stroke
Many studies have demonstrated the accuracy of Xe-CT in determining CBF values, which can then be used to identify areas of reversible and irreversible ischemia.16,20,21,23 Flow values determined with Xe-CT have also been used to identify volumes of eventual infarction and areas at increased risk for hemorrhage and edema.
Chronic Ischemia
Xe-CT–determined CBF has been used in conjunction with hypotensive or acetazolamide challenge to identify the subset of patients at higher risk for future infarction. For a detailed review of cerebral hemodynamic impairment, methods of measurement, and the association with stroke risk, please see Derdeyn and associates.23 These patients may benefit from either surgical (e.g., extracranial-to-intracranial bypass) or endovascular flow augmentation (Fig. 348-3).
Balloon Occlusion Testing
Xe-CT provides a well-studied method for obtaining quantitative values for CBF. Indeed, early work from Yonas’ group and others provides the basis for much of our understanding of cerebral hemodynamics.16,23,24 It can be used in conjunction with other CT studies such as CTA. Moreover, because it is so well studied, the results are standardized between different scanners and different centers.
Single-Photon Emission Computed Tomography
Technique
SPECT uses a radioisotope such as technetium 99m attached to a delivery compound such as hexamethylpropyleneamine oxime or ethylcysteinate dimer to cross the blood-brain barrier after intravenous injection. The radioisotope is metabolized and trapped in the tissue, with its uptake being proportional to CBF at the time of injection. Imaging can then be performed for several hours after the injection.3
Direct quantification of CBF is not generally possible without an indwelling arterial line, and therefore a semiquantitative approach is frequently used. In this method, the relative counts of the radioisotope are compared between the area of interest (i.e., the area of presumed infarction) and another control area. One drawback of the technique is that patients with acute stroke frequently have underlying chronic ischemia, which may make interpretation of relative uptake difficult. In addition, acute changes in CBF can be seen in “unaffected” control areas, which again will obfuscate direct comparison of radioisotope uptake in different regions.3
Clinical Applications
Ischemia
SPECT imaging has been used in patients with both acute and chronic ischemia to determine areas of infarction, as well as areas of ischemia that are at risk for infarction.3 Although the technique frequently provides only semiquantitative or relative data comparison, it has still been shown to have a good degree of sensitivity and specificity for stroke detection,25 as well as for correlation with stroke volume, response to thrombolytic therapy, and clinical outcome.26,27
Vasospasm
SPECT evidence of hypoperfusion has been shown to correlate with delayed neurological deficits in the setting of SAH.28 It may be able to provide noninvasive evidence of vasospasm when used in conjunction with the transcranial Doppler method.
Balloon Occlusion Testing
SPECT (Fig. 348-4) can be used as an adjunct to angiographic, electroencephalographic, and neurological determination of a patient’s ability to tolerate hypoperfusion in a given vascular territory.
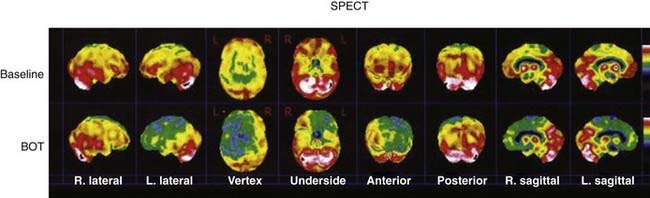
FIGURE 348-4 A 78-year-old woman with a history of a previously coiled left carotid-ophthalmic, internal carotid artery aneurysm was found to have significant recurrence on magnetic resonance angiography (MRA) and digital subtraction angiography (DSA). In preparation for possible vessel sacrifice, multiple planar and tomographic images of the cerebral hemispheres were obtained 30 minutes after the injection of 30.1 mCi of radiolabeled cerebral perfusion agent and balloon inflation. Single-photon emission computed tomography showed that when compared with the baseline study, there is a dramatic change in the relative perfusion of the left cerebral hemisphere. Markedly decreased activity is noted throughout the left frontal, temporal, and parts of the posterior parietal region in the left hemisphere. In addition, the medial temporal lobe and basal ganglion on the left show decreased activity in comparison to baseline. BOT, balloon occlusion test. (For related DSA and MRA images, please see Fig. 348-7.)
Magnetic Resonance Imaging–Based Techniques
Magnetic Resonance Angiography
Technique
MRI utilizes radiofrequency (RF) excitation/pulses and specific magnetic localizing fields to enable visualization of variations in proton spin.29,30 Currently, there are a variety of methods by which MRI can be optimized to acquire angiographic data. However, a detailed and exhaustive discussion is beyond the scope of this chapter. For the purposes of introduction, there are three general approaches to MRA: time-of-flight (TOF), phase-contrast (PC), and contrast-enhanced (CE) MRA. In general, direct visualization of blood vessels with TOF or PC techniques is achieved by taking advantage of the dynamic nature of flowing blood in comparison to the static surrounding tissue to create “angiographic contrast” without the use of intravenous agents. CE MRA, however, relies on intravenous agents to provide contrast.
Time of Flight
TOF MRA is based on the principle known as flow-related enhancement (FRE). Briefly, MRI relies on the application of RF excitation pulses to a given section or slice.29,31 Repeated pulses without adequate recovery time can saturate the section and reduce signal intensity. Stationary tissue is subject to repeated pulses and thus has attenuated intensity. Conversely, moving tissue (flowing blood) will not be subject to the saturation effects of repeated pulses because it washes in and out of the imaging plane. As a result, flowing blood appears brighter than the static surrounding tissue. FRE is increased as the vessel approaches a perpendicular orientation with respect to the slice plane. Simply stated, as blood flow velocity increases in the plane perpendicular to the slice, FRE increases. Unfortunately, based on the same principles, imaging of slow flow and in-plane flow can be challenging with the TOF technique.31 As discussed later, use of contrast agents, which helps increase intensity, can partially mitigate this problem.
The typical TOF technique uses gradient-recalled echoes.29,30