Chapter 4 Neurologic Examination of the Term and Preterm Infant
The Term Infant
Fetal monitoring during the labor process is difficult, but certain variables can be studied. The fetal biophysical profile includes fetal heart rate reactivity, fetal breathing movements, gross body movements, fetal tone (flexor-extensor movements and posture), and amniotic fluid volume. Ultrasonography has enabled this type of evaluation [Manning et al., 1998]. Other methods of evaluating the fetus are also utilized in various settings [Devoe, 2008]. Fetal central nervous system malformations detected by magnetic resonance imaging (MRI), performed when suspicion of malformations exists, are of value [Herman-Sucharska et al., 2009]. Injury of the developing brain may be offset by the plasticity inherent in the nervous system during the early stages of maturation [Johnston et al., 2009]. Assessment also routinely takes place by means of electronic fetal monitoring [Volpe, 2008a]. Alterations in fetal heart rate patterns may be valuable in assessing fetal status.
The term infant is examined, when possible, immediately after birth. Apgar scores are routinely obtained for term infants at the time of birth (Apgar, 1953). The categories for scoring are described in Table 4-1. Details of the scoring and the total scores often provide useful information concerning the newborn’s status for the examiner and subsequent health-care providers. Use of the Sarnat score is of value in assessing term infants who are encephalopathic at birth (Table 4-2) [Sarnat and Sarnat, 1976].
The neurologic examination of a term infant should be conducted in a quiet and evenly lit area that is suitably warmed so that the infant remains comfortable after removal of clothing and covering. When the infant is in a stable condition, a thorough examination during the first day is customary. If possible, another examination should be performed on the second or third day of life several hours after feeding so that the infant is optimally responsive. This examination is usually performed just before discharge. In emergent situations, the infant should be evaluated after stabilization has been achieved. It is often necessary to examine the infant on several occasions to confirm the presence and monitor the evolution of abnormal findings. Many protocols for the examination of the term infant (gestational age of 38–42 weeks) have been written [Amiel-Tison, 2002; Ashwal, 1995; Brazelton, 1973; Dubowitz and Dubowitz, 1981; Paine, 1960; Peiper, 1963; Prechtl, 1977]; some investigators have addressed the subject as part of the discussion of neonatal neurology [Fenichel, 2001; Volpe, 2008b]. Estimation of gestational age is discussed later in this chapter.
It is essential that the examination of the term infant be conducted in a systematic manner. Examination of the sick neonate may be difficult because of the presence of monitoring wires, sensors, catheters, eye shields, and infusion lines; however, systematic order in the sequence and extent of examination must be maintained to provide optimal information. These sick infants often must be examined on multiple occasions for sequential monitoring purposes and to complete portions of the examination not possible at the first encounter. The examiner should bear in mind that by 6 weeks post term, neurologic signs, particularly those related to muscle tone and posture, should reflect maturation of the nervous system [Guzzetta et al., 2005]. Furthermore, prediction of long-term development may be possible with the use of a combination of multiple complementary tools, including achieved milestones, neurological examination, and assessment of the quality of motor behavior [Heineman and Hadders-Algra, 2008].
Cranial Vault Evaluation
Among the most important facets of the examination is the measurement of the occipitofrontal (head) circumference. For the most part, this measurement is a reflection of brain growth. However, undue enlargement may be associated with cephalohematoma, subdural fluid collection, hydrocephalus, hydranencephaly, macrocephaly, or megalencephaly (Chapters 21–28). Serial measurements provide an index of brain growth in sick neonates. Microcephaly may be associated with many conditions, including intrauterine infection, hereditary abnormalities, maternal substance abuse, and poor nutrition.
The measurement of the occipitofrontal circumference should be performed carefully. An assistant may be necessary to stabilize the head during measurement. The measuring tape should be moved up and down the head until the largest circumference is obtained. The shape of the head influences the measure of the circumference. The nearer the head shape approximates a perfect circle, the smaller is the head circumference compared with the circumference of a noncircular head, despite the fact that the area of a plane through the maximal circumference and the brain volumes are the same. A similar relationship exists between a perfect sphere and the volume contained within it. The occipitofrontal circumference should be plotted on a graph standardized for gender, race, and gestational age to determine if the measurement falls within the normal range (i.e., two standard deviations above or below the mean) (Figure 4-1) [Braun et al., 2004]. On average, occipitofrontal circumference increases 2 cm during the first month of life, 6 cm during the first 4 months, 7 cm during the first 6 months, and 12 cm during the first 12 months of life [Fujimura and Seryu, 1977].
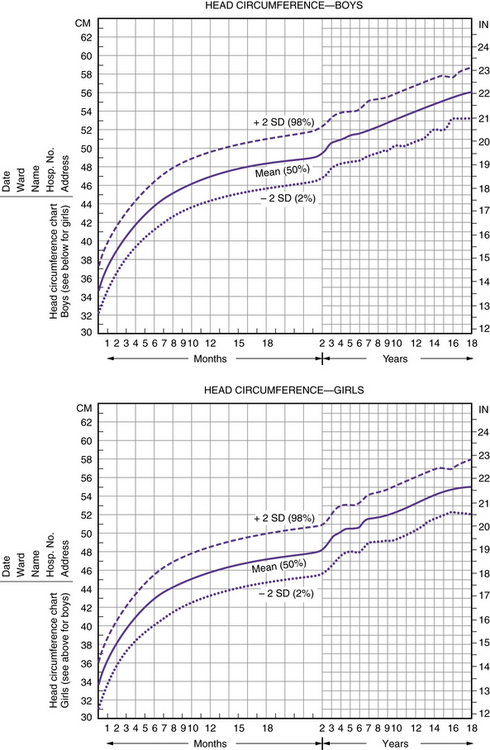
Fig. 4-1 Head circumference charts.
(From Nellhaus G. Composite international and interracial graphs. Pediatrics 1968;41:106.)
Infants delivered vaginally may have some deformity of the head because of scalp and subcutaneous edema with resulting caput succedaneum formation; vacuum extraction delivery often results in caput formation. Infants delivered by cesarean section usually have relatively round heads. The caput deformity, usually transient, produces an increased diameter and may confound accurate occipitofrontal circumference measurements. Cephalohematomas, which are delimited by the periosteum of the individual cranial bones, produce asymmetry of the head and increase the occipitofrontal circumference. Most occur over the parietal bones. A caput succedaneum, unlike a cephalohematoma, extends over two or more cranial bones and is not restricted to the subperiosteal (subgaleal) space. Subgaleal hematomas result from bleeding under the scalp aponeurosis and are often preceded by forceps or vacuum-assisted delivery space [Kilani and Wetmore, 2006]. The scalp may be edematous and boggy because of underlying blood. Although most subgaleal hematomas are benign, hypovolemic shock may ensue if a large amount of blood is sequestered in the subgaleal space.
The anterior fontanel, readily palpable at birth, is concave or flat in relation to the surrounding cranium. The fontanel should be assessed with the child held in the sitting position if there is any question of increased pressure. The fontanel may bulge during crying or in the presence of pathologic increased intracranial pressure. Unfortunately, the presence of normal conformation of the fontanel does not guarantee normal pressure; conversely, a bulging anterior fontanel strongly suggests increased intracranial pressure. The anterior fontanel varies in size but usually ranges from 1 to 3 cm in its longest dimension [Popich and Smith, 1972]. The fontanel pulsates synchronously with the infant’s pulse. The posterior fontanel in the neonate usually is open but admits only a fingertip. The presence of an enlarged posterior fontanel suggests the possibility of intrauterine increased intracranial pressure. From time to time, particularly in the presence of wormian bones, auxiliary fontanels may be palpable. A detailed discussion of the infant skull can be found in Chapter 28.
Auscultation over the infant skull, particularly the anterior fontanel and neck vessels, usually reveals a venous hum in a number of locations. Rarely, systolic-diastolic bruits, particularly those that are focal and asymmetric, indicate the presence of an arteriovenous malformation [Dodge, 1956]; however, these bruits may be heard in normal infants.
Developmental Reflexes
Developmental reflexes are primitive reflexes with complex responses, and largely reflect the integrity of the brainstem and spinal cord; the role of higher centers, although of importance, is not fully known. Many of these reflexes are present at birth and undergo modification during the first 6 months of life. Detailed discussion of these reflexes is presented in Chapter 3. Their persistence beyond the expected date of dissipation suggests maturational lag or impaired CNS function. This group includes the Moro, rooting, grasping, tonic neck, stepping, and placing reflexes. Generalized diminution of the manifestation of these reflexes suggests diffuse depression of brain function. Asymmetry indicates central or peripheral nervous system dysfunction that must be further localized. It is likely that infants born after breech presentation may have significant suppression of active movements when examined at the second and fourth days of life [Sekulic et al., 2009]. A stereotypic “elbowing” movement in newborns has been described. A curved wooden model of an ultrasonographic probe is gently used to exert pressure on the right and left subcostal regions. The newborn reacts with a particular defensive arm movement in which there is a three-phase response [Saraga et al., 2007].
Motor Function
Gentle manipulation of the infant’s limbs allows for assessment of muscle tone and strength. Tone is defined as resistance to passive movement (see Chapter 5). Tone at each large joint should be evaluated while the infant is at rest. Spontaneous movements and resistance of the infant to limb and trunk movement provide a measure of muscle strength. The examiner should recall any clues from the observation period suggesting muscle weakness and corroborating changes in tone and strength at this time. The infant should be supine with the head in the midposition while tone is evaluated so that the tonic neck reflex does not augment tone unilaterally.
Deep tendon reflexes are elicited using a reflex hammer and are often brisk in the newborn, although they may be normally absent [Critchley, 1968]. They may be inordinately enhanced by upper motor neuron abnormalities and are further facilitated by crying. CNS depression may be associated with reduced deep tendon reflexes. The examiner should confirm that the deep tendon reflexes are symmetric, because asymmetry may indicate central or peripheral nervous system impairment. If previous examination has suggested the possibility of hemiparesis, deep tendon reflexes should be carefully evaluated for asymmetry; they are usually increased on the affected side. Deep tendon reflex asymmetry in the arms may be associated with upper motor neuron abnormality, but asymmetrically absent deep tendon reflexes suggest peripheral involvement, possibly the result of brachial plexus injury. Nerve conduction studies in newborns may provide an index of neurologic maturity [Dubowitz et al., 1968].
Controversy remains over the significance of the plantar response in the newborn period in term infants. Although some investigators have reported that the Babinski sign is flexor and symmetric in the newborn period [Hogan and Milligan, 1971], this finding is more likely caused by obtaining a plantar grasp than a Babinski response if only the sole of the foot is used to elicit the response. The plantar response is extensor for at least the first month of life and usually through the first year of life. However, at all times, the response should always be bilaterally symmetric. Persistence of extensor toe-sign responses beyond infancy suggests corticospinal tract impairment and may be associated with alterations in tone and other deep tendon reflex abnormalities. Ankle clonus is frequently elicited in the newborn; rarely are there more than eight beats in normal infants. The clonus is enhanced during crying and may be facilitated during hyperexcitable states, such as those associated with metabolic abnormalities, infection, and subarachnoid hemorrhage. Sustained ankle clonus has the same significance in term newborns as in later life and suggests dysfunction of the corticospinal tracts.
A reflex akin to the plantar response has been described for the hand in term and preterm newborns. The examiner strokes the ulnar aspect of the infant’s palm with the thumb, beginning distally and stroking proximally from the small finger to the hypothenar eminence. The normal response is gradual extension of the fingers, beginning with the small finger and continuing to the middle fingers [Modanlou, 1988]. Lack of response or gross alteration of response may be observed in the presence of corticospinal tract dysfunction.
Cranial Nerve Examination
A more detailed discussion of the cranial nerve examination is found in Chapter 2. Cranial nerve I, the olfactory nerve, is infrequently tested but may be evaluated by the use of pleasant but definitive aromatic substances, such as cinnamon and cloves [Sarnat, 1978]. The infant usually manifests an arrest of activity, arousal, and sucking activity when exposed to these aromas. Virtually all neonates born after more than 32 weeks’ gestation respond [Sarnat, 1978].
Examination of the optic fundi may be difficult but is necessary. Numerous changes, including chorioretinitis (i.e., salt-and-pepper pigmentary changes), may be observed. Hemorrhages are commonly detected after vaginal delivery, even in the absence of traumatic delivery. The optic nerve may be hypoplastic, as manifested by a small, pearl-colored optic disc. The color of the optic disc in the newborn infant is grayish white. Retinal hemorrhages may be found in a large percentage of otherwise normal infants who have no history of abnormal delivery and who later prove to be neurologically normal [Besio et al., 1979]. Further discussion of funduscopic characteristics is presented in Chapter 6.
The newborn infant turns toward a light of moderate intensity and fixes on a bright object or the examiner’s face. Most often, the newborn’s eyes are symmetrically open or closed. If one eye is open and the other closed, there should be a shifting from one side to the other. Width of palpebral fissures should be equal; if not, the presence of ptosis should suggest an abnormality of cranial nerve III function, sympathetic innervation dysfunction, neuromuscular junction difficulty, weakness of the levator muscle of the lid, or abnormality of the lid connective tissue. Among the conditions to be considered are congenital myasthenia gravis, myotonic dystrophy, Horner’s syndrome (Figure 4-2), Möbius’ syndrome, congenital myopathies, and Duane’s syndrome. Occasionally, central or peripheral seventh nerve paresis may result in asymmetry of the palpebral fissure.
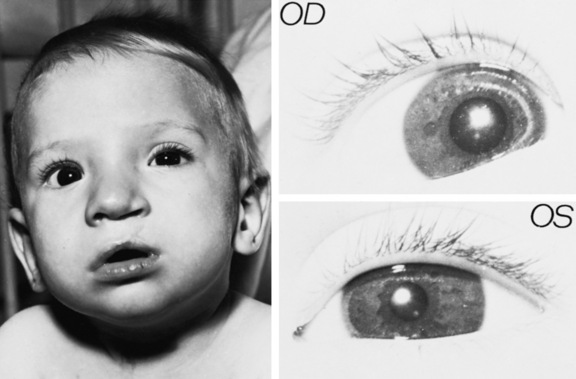
Fig. 4-2 Horner’s syndrome (left eye).
Miosis and ptosis are plainly evident.
(Courtesy of the Division of Pediatric Neurology, University of Minnesota Medical School.)
Extraocular movements should be monitored while a child is lying quietly. Slight lapses of conjugate gaze are common in the newborn period. Newborn visual acuity is difficult to assess, but black and white-patterned objects can be used. The examiner’s face is often the best “target.” The intended object of focus is moved slowly in the infant’s field of vision, less than a foot from the infant’s eyes. The infant slowly follows with eye movement, particularly in lateral directions. Prolonged gaze may occur in the newborn period [Brazelton et al., 1976]. Opticokinetic nystagmus may be elicited by using a striped, rotating drum or striped cloth strip, which is slowly pulled across the infant’s visual field in the vertical and horizontal directions. The response is the same as in older children (see Chapter 2).
Cranial nerve VII involvement may be the result of the position of the infant in the maternal pelvis and delivery by pressure incurred during forceps delivery, or by agenesis of the motor nucleus of cranial nerve VII. Facial movements are readily observed during crying; an asymmetry of mouth movement may indicate cranial nerve VII involvement. During crying, the angle of the mouth is depressed on the normal side. The syndrome referred to as asymmetric crying facies may manifest this way [Nelson and Eng, 1972]. This syndrome results from weakness of the lower lip caused by hypoplasia of the depressor muscle of the mouth angle. This phenomenon is a congenital abnormality and does not signal cranial nerve VII involvement. This condition also may be associated with somatic atrophy, vertebral and rib abnormalities, renal dysgenesis, and most importantly, cardiac defects (i.e., atrial or ventricular septal defect; cardiofacial syndrome) [Pape and Pickering, 1972].