Neonatal and Pediatric Respiratory Disorders
After reading this chapter you will be able to:
Discuss the clinical findings, radiographic abnormalities, and treatment of patients with respiratory distress syndrome.
Describe the clinical manifestations and treatment of patients with transient tachypnea of the newborn.
Describe the pathophysiology, presentation, and treatment of meconium aspiration syndrome.
Identify the clinical signs and symptoms associated with bronchopulmonary dysplasia and the approaches used to manage these infants.
State the etiology and treatment of apnea of prematurity.
Describe the pathophysiology, diagnosis, and treatment of persistent pulmonary hypertension of the newborn.
Discuss the pathophysiology, diagnosis, and treatment of congenital diaphragmatic hernia.
Identify the anatomic defects associated with tetralogy of Fallot.
Describe the clinical presentation of a ventricular septal defect.
Define the epidemiologic factors associated with increased risk of sudden infant death syndrome.
Identify the respiratory problems associated with gastroesophageal reflux disease.
State the clinical findings commonly observed in patients with bronchiolitis.
Describe the clinical features and treatment of children with epiglottitis.
Describe the clinical manifestations and treatment of cystic fibrosis.
Neonatal Respiratory Disorders
Lung Parenchymal Disease
Respiratory Distress Syndrome
Pathophysiology
Figure 31-1 outlines the pathophysiologic events associated with RDS. A qualitative decrease in surfactant increases alveolar surface tension forces. This process causes alveoli to become unstable and collapse and leads to atelectasis and increased work of breathing. At the same time, the increased surface tension draws fluid from the pulmonary capillaries into the alveoli. In combination, these factors impair oxygen (O2) exchange and cause severe hypoxemia. The severe hypoxemia and acidosis increase pulmonary vascular resistance (PVR). As pulmonary arterial pressure increases, extrapulmonary right-to-left shunting increases, and hypoxemia worsens. Hypoxia and acidosis also impair further surfactant production. Antenatal steroids have been shown to mature surfactant function.
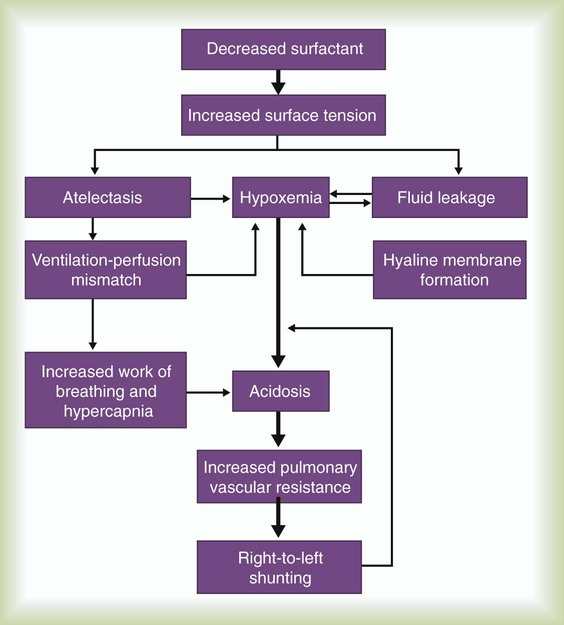
Clinical Manifestations
A definitive diagnosis of RDS usually is made with chest radiography (Figure 31-2). Diffuse, hazy, reticulogranular densities with the presence of air bronchograms with low lung volumes are typical of RDS. The reticulogranular pattern is caused by aeration of respiratory bronchioles and collapse of the alveoli. Air bronchograms appear as aerated, dark, major bronchi surrounded by the collapsed or consolidated lung tissue.
Treatment
Continuous positive airway pressure (CPAP) and positive end expiratory pressure (PEEP) are the traditional support modes used to manage RDS. Surfactant replacement therapy and high-frequency ventilation (HFV) have been added to these traditional approaches.1–5 Unless the infant’s condition is severe, a trial of nasal CPAP is indicated (4 to 6 cm H2O).6,7 Because of the hazards of endotracheal tubes, nasal prongs are preferred. If the infant’s clinical condition deteriorates rapidly, a more aggressive approach is required. Endotracheal intubation should be performed under controlled conditions as an elective procedure. Mechanical ventilation with PEEP should be initiated if oxygenation does not improve with CPAP or if the patient is apneic or acidotic. There is significant interest in an approach comprising intubation, delivery of surfactant, extubation, and then nasal CPAP.8 However, more research is needed to understand the risks and benefits of this approach.
Three surfactant preparations are used in the United States for management of neonatal RDS: beractant (Survanta; Abbott Laboratories, North Chicago, IL), calfactant (Infasurf; ONY, Inc, Amherst, NY), and poractant alfa (Curosurf; Chiesi, Cheadle, United Kingdom).4,5,7–9 Beractant and calfactant are natural bovine surfactant extracts. Poractant alfa is a natural porcine surfactant extract. Each of these three natural surfactants has surfactant proteins B and C as part of the formulation. These surfactant proteins are important for decreasing alveolar surface tension. All of these preparations are liquid suspensions that are instilled directly into the trachea. The current standard of care is to deliver replacement surfactant to all infants with RDS. An additional artificial surfactant, lucinactant, is being actively studied.9 The ability to nebulize with this new surfactant is an exciting possibility. At the present time, no evidence supports the use of a particular brand of surfactant.
Surfactant replacement therapy also is used as both a rescue treatment (of infants who already have RDS) and a prophylactic therapy (in the care of infants delivered prematurely).10–13 Some centers use prophylactic surfactant replacement therapy in the care of all very small infants (<1500 g). Therapies aimed at decreasing pulmonary edema, improving cardiac output, and weaning from O2 and high ventilator pressures are essential in the successful treatment of infants receiving surfactant.
All surfactants are delivered via the endotracheal tube. Animal studies suggest that surfactant is rapidly distributed throughout the lung.14 Each specific surfactant has different dosing volumes and intervals (Table 31-1). The surfactant product insert describes the positioning of the infant for surfactant delivery. Basically, the infant is positioned with different sections of the lung dependent so that the surfactant enters that section of the lung with gravity flow. If the infant is very sick and cannot be repositioned, surfactant can be administered with the infant in a supine position.
TABLE 31-1
Beractant (Survanta) | Calfactant (Infasurf) | Poractant alfa (Curosurf) | |
Dose mg/kg of phospholipid | 100 | 100 | 100-200 |
ml/kg | 4 | 3 | 1.25-2.5 |
Administration | ![]() |
![]() |
Whole or ![]() |
Dosing interval | Every 6 hr or more often | Every 12 hr or more often | Every 12 hr or more often |
Transient Tachypnea of the Newborn
Background
Transient tachypnea of the newborn (TTN), often called type II RDS, is probably the most common respiratory disorder of newborns. The cause of TTN is unclear, but it is most likely related to delayed clearance of fetal lung liquid.15–29 During most births, approximately two-thirds of this fluid is expelled by thoracic squeeze in the birth canal; the rest is reabsorbed through the lymphatic vessels during initial breathing. These mechanisms are impaired in infants born by cesarean section or infants with incomplete development of the lymphatic vessels (preterm or small-for-gestational-age infants). The residual lung fluid causes an increase in airway resistance and an overall decrease in lung compliance. Because compliance is low, the infant must generate more negative pleural pressure to breathe. This process can result in hyperinflation of some areas and air trapping in others. Most infants with TTN are born at term without any specific predisposing factors in common. Mothers of neonates who have TTN tend to have longer labor intervals and a higher incidence of failure to progress in labor, which leads to cesarean delivery. In many cases, however, maternal history and labor and delivery are normal.
Meconium Aspiration Syndrome
Pathophysiology
Amniotic fluid consists mainly of fetal lung fluid, fetal urine, and transudate from the uterine wall. Meconium, the contents of the fetal intestine, occasionally is expelled from the fetus into the surrounding amniotic fluid. Meconium consists of mucopolysaccharides, cholesterol, bile acids and salts, intestinal enzymes, and other substances. Meconium normally is not passed until after delivery.30 Infants who have marked perinatal depression or perinatal asphyxia may pass meconium in utero. The pathophysiologic control mechanisms for the passage of meconium in utero are not completely understood. It is widely accepted that infants can have meconium aspiration in utero. Amniotic fluid stained with meconium is found in approximately 12% of all births.30 Meconium-stained amniotic fluid is rare among infants of less than 37 weeks’ gestational age. The clinical syndrome develops in 2 of every 1000 infants. Of infants with inhaled meconium, 95% clear their lungs spontaneously.30Amniotic fluid infusion into the uterus before the delivery of infants with meconium-stained fluid has been shown to improve neonatal outcomes.31,32
For many years, the aspirated meconium itself was considered the primary cause of MAS. More recent evidence suggests that the real causative agent is fetal asphyxia that precedes aspiration.23 Fetal asphyxia causes pulmonary vasospasm and hyperreactivity of the vasculature, which lead to persistent pulmonary hypertension.
MAS involves three primary problems: pulmonary obstruction, lung tissue damage, and pulmonary hypertension.33 Obstruction occurs because of plugging of the airways with particulate meconium. This obstruction often is of the ball-valve type, which allows gas entry but prevents gas exit. Ball-valve obstruction causes air trapping and can lead to volutrauma (Figure 31-3). The lung tissue injury caused by MAS is chemical pneumonitis. Additionally, there are various chemical effects, inflammatory responses, cytokine and chemokine activations, complement activation, and phospholipase A2 activation.33–37 Persistent pulmonary hypertension with intracardiac and extracardiac right-to-left shunting frequently complicates MAS.30
Clinical Manifestations
Before birth, thick meconium, fetal tachycardia, and absent fetal cardiac accelerations during labor are evidence that the fetus is at high risk of MAS.38 After delivery, if the infant has a low umbilical artery pH, an Apgar score less than 5, and meconium aspirated from the trachea, intensive care and close observation for MAS are warranted. Infants with MAS typically have gasping respirations, tachypnea, grunting, and retractions. The chest radiograph usually shows irregular pulmonary densities, which represent areas of atelectasis, and hyperlucent areas, which represent hyperinflation secondary to air trapping (Figure 31-4). Arterial blood gases typically show hypoxemia with mixed respiratory and metabolic acidosis. In the most severe cases, there is right-to-left shunting and persistent pulmonary hypertension.30
Treatment
It is no longer recommended that vigorous infants with meconium-stained fluid be intubated and suctioned.31,39–41 However, it is important that an endotracheal tube be inserted immediately in severely depressed infants with thick meconium, and suction should be applied directly to the endotracheal tube.40 The endotracheal tube is removed and inspected for meconium. If meconium is present, the procedure is repeated with a new endotracheal tube until no further meconium is aspirated or until two to four aspirations have been performed. The endotracheal tube should be left in place, and mechanical ventilation should be started. For prevention of hypoxemia, a flow of warmed 100% O2 should be blown across the infant’s face during the aspiration efforts. No evidence suggests an improved outcome because of endotracheal suctioning in the care of infants who have meconium and are vigorous and would not otherwise require intubation.42,43 There is evidence that tracheal lavage with dilute surfactant improves the clinical course and outcome of infants with MAS.44–46
If the infant’s condition worsens, CPAP or mechanical ventilation may be indicated. CPAP is indicated if the primary problem is hypoxemia. By distending the small airways, CPAP can sometimes overcome the ball-valve obstruction and improve both oxygenation and ventilation. If respiratory acidosis is severe or clinical assessment indicates excessive work in breathing, mechanical ventilation should be started. Figure 31-3 shows the ball-valve effect. At rest, the airway lumen is partially obstructed. With inspiration, negative intrathoracic pressure opens the airway and relieves the obstruction. Gas enters and expands the alveoli. With expiration, intrathoracic pressure changes to a positive force, which narrows the airway and causes total occlusion. Gas cannot be expelled and is trapped within the alveoli. It is difficult to provide ventilation to infants with severe MAS. These infants often retain CO2 and need increased ventilator support. Because of high airways resistance, the lungs have a long time constant. High ventilator rates and pressures increase the risk of air trapping and volutrauma.
Evidence suggests that both HFV and synchronous intermittent mechanical ventilation decrease the risk of air leak.47 Various studies have shown improvement in MAS with the use of HFV and surfactant.48 Nitric oxide has become a major adjunct in the management of persistent pulmonary hypertension.49 Corticosteroids have not yet been shown to improve outcomes for infants with MAS.50 High mean airway pressures may worsen pulmonary hypertension and aggravate right-to-left cardiac shunting.38
Bronchopulmonary Dysplasia
Background
Infants, especially preterm infants, with severe respiratory failure in the first few weeks of life may develop a chronic pulmonary condition called bronchopulmonary dysplasia (BPD). BPD is a complex disease that is poorly defined.51–54 Historical definitions have included radiographic patterns and the requirement for supplemental O2 at fixed time points in the infant’s life. Immaturity, genetics, malnutrition, O2 toxicity, and mechanical ventilation all have been implicated in the origin of BPD.51,55–62
Pathophysiology
The development of BPD is complex and involves many pathways. The initiating factors are related to atelectrauma (lung collapse) and volutrauma (large tidal volume [VT]). Factors such as hyperoxia and hypoxia, mechanical forces, vascular maldevelopment, inflammation, nutrition, and genetics contribute to the abnormal development of the lung and lead to BPD.56,59,63–67 Atelectrauma is a term coined to describe loss of alveolar volume that is both a consequence and a cause of lung injury. Volutrauma is the term used to describe local overinflation (and stretch) of airways and alveoli. Atelectrauma leads to derecuitment (e.g., areas of alveolar collapse) of the lung. Volutrauma leads to damage to airways, pulmonary capillary endothelium, alveolar and airway epithelium, and basement membranes. The combination of atelectrauma and volutrauma synergistically increases lung injury.
The response of the lungs to the combination of trauma and O2 toxicity is the production and release of soluble mediators. These mediators probably are released from granulocytes residing in the lung. The release of these mediators can injure the alveolar-capillary barrier and induce an inflammatory response.62 A “new” BPD is being described that shows decreased alveolarization rather than the prominent airway damage of the “old” BPD. This change in the pathologic characteristics of BPD is thought to be related to improvements in ventilator management, the use of surfactant, and processes that interrupt alveolar development (e.g., postnatal steroid therapy).54,68–70 Some authors speculate that the “new” BPD and “old” BPD are the same disease. The difference is that clinicians are better at performing mechanical ventilation in infants and do less damage to the airway compared with 20 years ago.71
Clinical Manifestations
BPD has various clinical manifestations. Some very immature infants may start with little or no O2 requirement and little or no mechanical ventilation requirement. Progressive respiratory distress develops at approximately 2 to 3 weeks of life, and then the infant needs O2 and mechanical ventilation. Other immature infants may begin with pneumonia or sepsis and need very high levels of O2 and mechanical ventilation. In either of these scenarios, progressive vascular leakage and areas of atelectasis and emphysema develop in the lungs, and progressive pulmonary damage occurs. The chest radiograph in severe disease shows areas of atelectasis, emphysema, and fibrosis diffusely intermixed throughout the lung (Figure 31-5).67,72 Arterial blood gas measurements reveal varying degrees of hypoxemia and hypercapnia secondary to airway obstruction, air trapping, pulmonary fibrosis, and atelectasis. There is a marked increase in airway resistance with an overall decrease in lung compliance.
Treatment
Multiple pharmacologic treatments have been advocated for infants with BPD.73 Diuretics are given as needed to decrease pulmonary edema; antibiotics are given to manage existing pulmonary infection.74 Chest physical therapy may help mobilize secretions and prevent further atelectasis. Bronchodilator therapy may be useful in decreasing airway resistance.75 Steroid therapy with dexamethasone can produce substantial short-term improvement in lung function, often allowing rapid weaning from ventilatory support. However, steroid therapy has little effect on long-term outcome such as mortality and duration of O2 therapy.76,77 Steroid therapy also has been implicated in decreased alveolarization and increased developmental delay.78 Although steroids are still given in clinical practice, they should be used cautiously and only after the risks have been thoroughly explained to the parents.
Control of Breathing
Apnea of Prematurity
Background
Apnea of prematurity is a common, controllable disorder among premature infants. It usually resolves over time.79–84 Premature infants frequently have periodic respiration, which comprises sequential short apneic episodes of 5 to 10 seconds followed by 10 to 15 seconds of rapid respiration. Apneic spells are abnormal if (1) they last longer than 15 seconds; or (2) they are associated with cyanosis, pallor, hypotonia, or bradycardia.
If no effort to breathe occurs during a spell, the apnea is called central apnea. If breathing efforts occur, but obstruction prevents airflow, the apnea is termed obstructive. Mixed apnea is a combination of the central and obstructive types that starts as obstructive apnea and then develops into central apnea.79–83,85
Etiology
Premature infants have immature control of respiratory drive in response to oxygen and carbon dioxide. In mature animals, an increase in alveolar PaCO2 elicits an increase in VT and respiratory rate. A decrease in FiO2 below room air also triggers an increase in VT. Conversely, in premature animals, an increase in PaCO2 temporarily increases VT but does not increase respiratory rate. A decrease in FiO2 below room air decreases VT and respiratory rate. This effect can lead to apnea in a premature infant. Several factors in addition to prematurity can cause apnea in infants. Table 31-2 summarizes the potential causes, associated signs, and diagnostic indicators.86
TABLE 31-2
Evaluation of an Infant With Apnea
Possible Cause | Associated Signs | Investigation |
Infection | Lethargy, respiratory distress, temperature instability | Complete blood count, sepsis evaluation |
Metabolic disorder | Poor feeding, lethargy, jitteriness | Glucose, calcium, electrolyte levels |
Impaired oxygenation | Respiratory distress, tachypnea, cyanosis | O2 monitoring, arterial blood gases, chest radiograph |
Maternal drugs | Maternal history, hypotonia, central nervous system depression | Magnesium level, urine drug screen |
Intracranial lesion | Abnormal neurologic findings, seizures | Cranial ultrasonography |
Environmental | Lethargy | Monitor temperature (infant and environment) |
Gastroesophageal reflux | Feeding difficulty | Specific observation, radiographic barium swallow examination |
From Stark AR: Disorders of respiratory control in infants. Respir Care 36:673, 1991.
Treatment
Infants with apnea need continuous monitoring of heart and respiratory rates. Continuous noninvasive monitoring of oxygenation by transcutaneous electrode or pulse oximetry is recommended. Most apneic incidents can be quickly terminated with gentle mechanical stimulation, such as picking the infant up, flicking the sole of the foot, or rubbing the skin.79,81,82,85,87 If the cause of apnea is not prematurity, treatment must be directed at resolving the underlying condition. Table 31-3 outlines current treatment strategies for infants with apnea.86 Apnea secondary to prematurity responds well to methylxanthines, especially theophylline and caffeine.82,85,87 These agents stimulate the central nervous system and increase the infant’s responsiveness to CO2. For infants with apnea that is refractory to treatment with theophylline, doxapram can be used.88–90 However, doxapram is delivered by continuous infusion and has multiple toxicities.
TABLE 31-3
Treatment Strategies for Infants With Apnea
Treatment | Rationale |
Manage underlying cause if identified | Removes precipitating factor |
Tactile stimulation | Increases respiratory drive by sensory stimulation |
CPAP | Reduces mixed and obstructive apnea by splinting the upper airway |
Theophylline or caffeine | Increases respiratory center output and CO2 response, enhances diaphragm strength, adenosine antagonist |
Doxapram | Stimulates respiratory center and peripheral chemoreceptors |
Transfusion | Decreases hypoxic depression by increasing O2-carrying capacity |
Mechanical ventilation | Provides support when respiratory effort is inadequate |
From Stark AR: Disorders of respiratory control in infants. Respir Care 36:673, 1991.
CPAP can also be used to manage infant apnea.91 Although the mechanism of action is not established, CPAP probably increases FRC and improves arterial partial pressure of oxygen (PaO2) and PaCO2. CPAP may also stimulate vagal receptors in the lung, increasing the output of the brainstem respiratory centers. Severe or recurrent apnea that is unresponsive to these interventions may necessitate mechanical ventilatory support.
Apnea monitoring can allow infants who are otherwise ready for discharge but still having occasional episodes of apnea to go home.79,81,85,92–95 However, the presence of a home apnea monitor is a significant inconvenience to the family. Home monitors lack the sophisticated filtering systems of hospital monitors, and they have very frequent false alarms.
Pulmonary Vascular Disease
Persistent Pulmonary Hypertension of the Newborn
Background
Persistent pulmonary hypertension of the newborn (PPHN) is a complex syndrome with many causes.96 The common denominator in PPHN is a return to fetal circulatory pathways, usually because of elevated PVR. This condition results in further right-to-left shunting, severe hypoxemia, and metabolic and respiratory acidosis.
Pathophysiology
There are three fundamental types of PPHN: vascular spasm, increased muscle wall thickness, and decreased cross-sectional area of pulmonary vessels.97 Vascular spasm is an acute event that can be triggered by many different conditions, including hypoxemia, hypoglycemia, hypotension, and pain. Increased muscle wall thickness is a chronic condition that develops in utero in response to several different etiologic factors, including chronic fetal hypoxia, increased pulmonary blood flow (e.g., intrauterine closure of the ductus arteriosus), and pulmonary venous obstruction (e.g., total anomalous pulmonary venous return with obstructed below-diaphragm return). Decreased cross-sectional area is related to hypoplasia of the lungs and occurs with congenital diaphragmatic hernia, Potter sequence (absent kidneys), and oligohydramnios syndromes (decreased amniotic fluid).