Microorganisms in the Environment and Environmental Safety
After reading this chapter, the student will be able to:
• Discuss microbial ecology and describe the levels of microbial relationships within the environment
• Discuss the importance of biogeochemical cycles, including the carbon, nitrogen, sulfur, and phosphorus cycles
• Discuss soil microbiology, with emphasis on the abundance and type of microbes in the soil
• Discuss aquatic microbiology, both freshwater and marine environments, and include the zones observable in these bodies of water
• Describe the characteristics of natural disasters including floods, tsunamis, earthquakes, and hurricanes
• Illustrate the most common infections/diseases associated with natural disasters
• Explain the concepts of biological warfare and bioterrorism
• Describe the initiatives taken to advance national public health capabilities in response to bioterrorism
• Define and describe the three groups of agents that are of concern in bioterrorism
Normal Environmental Conditions
Microbial Ecology
The study of the interrelationships of microorganisms in their natural environments is referred to as microbial ecology. Terms used to describe the levels of microbial relationships in the environment are as follows (Figure 24.1):
• Populations are formed by individual organisms through growth and reproduction.
• Guilds are populations of microorganisms that perform linked metabolic activities.
• Communities are sets of guilds and are characteristically heterogeneous. On occasion, especially in extreme environments, a community can consist of a single population.
• Microhabitats are specific small niches in which populations and guilds within a community reside because of optimal conditions for survival.
• Habitats are physical locations where organisms are found and they consist of groups of microhabitats. Within habitats the microorganisms interact with larger organisms.
• Ecosystems consist of organisms, their abiotic environment, and the relationships between them.
• Biospheres are regions of the earth populated by living organisms.
Thriving ecosystems are able to support great biodiversity, but many microbes live in harsh ecosystems where nutrients are limited. Therefore, in order to survive, these microbes must have special adaptation to a given environment. Environments may cycle between excess nutrient availability and depletion. To survive, some microorganisms form biofilms (see Chapter 6, Bacteria and Archaea) on surfaces where nutrients accumulate. Biodiversity is controlled by competition between the microbes, but usually an ecosystem is heterogenic. Microbes often use the waste products of other organisms for their own metabolic activities; in other cases the waste products of one type of microbe may make the environment more favorable for another. The formation of biofilms serves as a good example of this phenomenon.
Biodiversity ensures a healthy interaction between life forms on earth. This complex web of life is essential for the maintenance of sufficient food and freshwater. The United States Environmental Protection Agency (EPA) and other national and international organizations recognize the importance of biodiversity for human health and well-being. The EPA in partnership with other organizations implemented the biodiversity-human health project (http://epa.gov/ncer/biodiversity/index.html). One focus of the project is to better understand the relationship of emerging infectious diseases (see Chapter 18, Emerging Infectious Diseases), changing environments, and factors that manipulate biodiversity.
Biogeochemical Cycles and Microbes
A biogeochemical cycle is a circuit or pathway through which organic compounds or a chemical element is changed from one chemical state to another by moving through both biotic and abiotic compartments of an ecosystem (Box 24.1). The study of these chemical transformations is called biogeochemistry.
The Carbon Cycle
Carbon is the fourth most abundant element in the universe, and essential to life on earth. Carbon is the element that provides the backbone of all organic molecules (see Chapter 2, Chemistry of Life). All living organisms from microorganisms, to plants and animals, contain a large number of organic compounds and therefore a large amount of carbon. Thus the carbon cycle is considered to be the primary biogeochemical cycle.
The next step in the carbon cycle involves the consumption of autotrophs by chemoheterotrophs such as animals and protozoans, which then catabolize the organic molecules for energy, resulting in the release of CO2. Some organic molecules produced by the autotrophs may be incorporated into the tissues of heterotrophs, where they remain until that organism dies. Dead organisms are decomposed with the help of microorganisms, metabolizing organic molecules and releasing CO2 in the process. The release of CO2 into the atmosphere starts the cycle over again (Figure 24.2).
The Nitrogen Cycle
Nitrogen gas is the most abundant gas in the earth’s atmosphere, representing about 79% of all the gases in air. Unfortunately, the nitrogen in the atmosphere is unusable by most organisms. Only about 0.03% of the earth’s nitrogen is fixed in the form of nitrates, nitrites, ammonium ion, and organic compounds. Nitrogen is necessary for all living organisms for the synthesis of proteins, nucleic acids, and other nitrogen-containing compounds. The nitrogen cycle consists of several steps as illustrated in Figure 24.3.
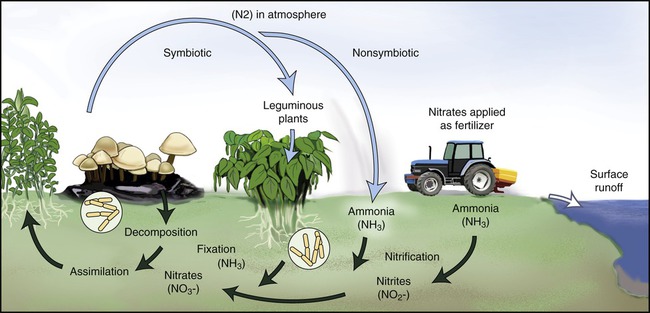
Nitrogen is also an essential element for living organisms. Nitrogen from the atmosphere goes through fixation, nitrification, and denitrification and absorption by living organisms. This is then followed by decomposition, ammonification, and then nitrification again.
Anammox is the acronym for anaerobic ammonium oxidation and is a recent addition to knowledge of the nitrogen cycle. In this process, performed by anammox bacteria (previously thought to be denitrifying bacteria), nitrite and ammonium are directly converted to dinitrogen gas. This process accounts for up to 50% of the dinitrogen gas produced in the oceans. Ammonia is the major source of fixed nitrogen in the oceans and because the anammox bacteria remove ammonia efficiently from those bodies of water, these organisms limit oceanic primary productivity. On the positive side, the ability of anammox bacteria to convert ammonium and nitrite directly to dinitrogen gas provides a promising alternative to current methods of removal of nitrogen from wastewater (see Life Application: Anammox and Wastewater Treatment).
The Sulfur Cycle
The sulfur cycle is similar to the nitrogen cycle, except that sulfur originates from natural sedimentary deposits. The sulfur cycle includes both atmospheric and terrestrial processes (Figure 24.4). The terrestrial process begins with the weathering of rocks, resulting in the release of stored sulfur. Subsequently, the sulfur comes in contact with air and is then converted into sulfate (SO4). Plants and microorganisms will take up the sulfate and convert it into organic forms. Animals will consume the organic forms via food they consume, thereby moving the sulfur through the food chain. As organisms die, bacteria decompose them, releasing sulfur-containing amino acids into the environment. The sulfur released from the amino acids is converted by microorganisms to its most reduced form, hydrogen sulfide (H2S). This process is called sulfur dissimulation. H2S is then oxidized to elemental sulfur and then to sulfate by various microorganisms under various conditions. These organisms include nonphotosynthetic autotrophs such as Thiobacillus and Beggiatoa and photoautotrophic green and purple sulfur bacteria. The cycle starts again with the uptake of sulfate by plants and algae that animals eat. Anaerobic respiration by the bacterium Desulfovibrio reduces sulfate back to H2S.
The Phosphorus Cycle
Phosphorus is an essential nutrient for plants, animals, and microorganisms. It is part of nucleic acids (DNA, RNA, ATP, and ADP) and of the plasma membranes (phospholipids). Phosphorus can be found in water, soil, and sediments. Unlike nitrogen and sulfur, phosphorus cannot be found in the atmosphere in the gaseous state. Phosphorus exists in the form of phosphate ion and undergoes little change in its oxidation state. The phosphorus cycle (Figure 24.5) involves changes of phosphorus from insoluble to soluble forms that are available for uptake by organisms, and from organic to inorganic forms by pH-dependent processes. Because no gaseous form of phosphorus exists it has the tendency to accumulate in the seas and other bodies of water.
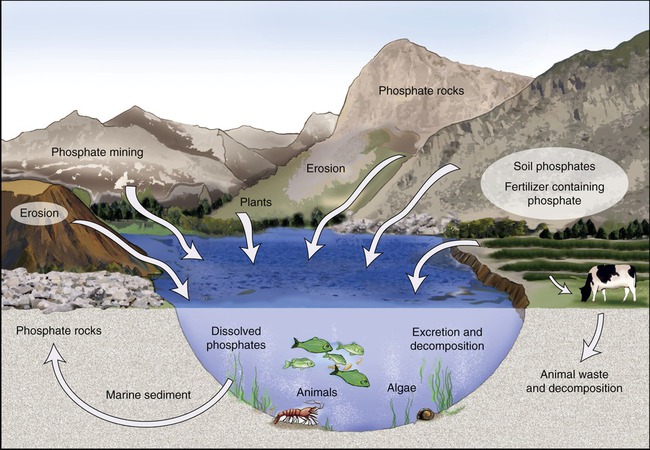
The phosphorus cycle involves changes of phosphorus from insoluble to soluble forms that are available for uptake by organisms, and from organic to inorganic forms by pH-dependent processes. Because no gaseous form of phosphorus exists it has the tendency to accumulate in the seas and other bodies of water.
Soil Microbiology
Soil composition such as organic matter, soil structure, nutrient content, nutrient cycling, nutrient availability, and water-holding capacity are influenced by, or dependent on, billions of organisms, microscopic ones as well as fairly large insects and earthworms. All these organisms together form a pulsating, living community in soil. Soil microorganisms can be classified into major groups such as microarthropods, nematodes, protozoa, fungi, algae, and bacteria. The most numerous organisms in the soil are bacteria—each gram of typical soil contains millions of bacteria. The population of bacteria is largest in the top few centimeters of soil and declines rapidly with depth. Organic matter is metabolized by microbes in the soil, and via the biogeochemical cycles, elements are oxidized and reduced (see Chapter 2, Chemistry of Life, for oxidation/reduction reactions) by microorganisms to meet their metabolic needs.
• Moisture is essential for the survival of microorganisms. In dry soil microbes will exhibit lower metabolic activity, they will be less diverse, and lower numbers will be present than in moist soil.
• Oxygen dissolves poorly in water; therefore, moist soils are lower in oxygen content than dry soils. Waterlogged soil will result in a decline in microbial diversity and anaerobic organisms will dominate. The presence or absence of rainwater determines moisture, and thus dissolved oxygen.
• The pH of soil influences the type of organisms that predominate. It will influence whether the soil is rich in bacteria or rich in fungi. In highly acidic or highly basic soils fungi will predominate, whereas bacteria prefer soil with a pH of about 7.
• The temperature of soil determines the types of microbe that can flourish. Most soil organisms are mesophiles and grow well in areas where winters and summers are not too extreme. Psychrophiles grow only in cold environments and cannot survive in areas that experience spring thawing. Thermophiles, on the other hand, grow only in warm environments where winters are not harsh.
• Nutrient availability also affects the microbial abundance and diversity of soil. The majority of soil microbes are heterotrophic and utilize the organic matter present in the soil. It is the amount of organic material rather than the kind of organic material that determines the abundance of microorganisms in a microbial community. A continuous influx of organic material (i.e., agricultural land) will support a wider assortment of microorganisms than soil with low amounts of organic material.
Microbial Populations in Soil
HEALTHCARE APPLICATION
Examples of Soil-borne Diseases
Microorganism | Disease |
Bacillus anthracis | Anthrax |
Clostridium tetani | Tetanus |
Hantavirus | Hantavirus pulmonary syndrome |
Histoplasma capsulatum | Histoplasmosis |
Blastomyces dermatitidis | Blastomycosis |
Sporothrix schenckii | Sporotrichosis |
Aquatic Microbiology
Freshwater Ecosystems
About 3% of the world’s water is freshwater, 99% of which is either frozen in glaciers and pack ice, or is buried in aquifers. The remainder of the freshwater is found in lakes, ponds, rivers, and streams. Microorganisms are dispersed vertically within lake and pond systems, according to the availability of oxygen, light intensity, and temperature. Surface waters are higher in oxygen and light intensity, and warmer than deeper waters. In contrast to stagnant waters where oxygen is readily depleted, in larger lakes the wave action continuously mixes nutrients, oxygen, and organisms, resulting in efficient use of its resources. In stagnant waters, because of the depletion of oxygen, anaerobic organism will dominate and a lesser water quality will be the result. Four distinct vertical zones can be observed in lakes and ponds (Figure 24.6): a littoral zone, a limnetic zone, a profundal zone, and a benthic zone:
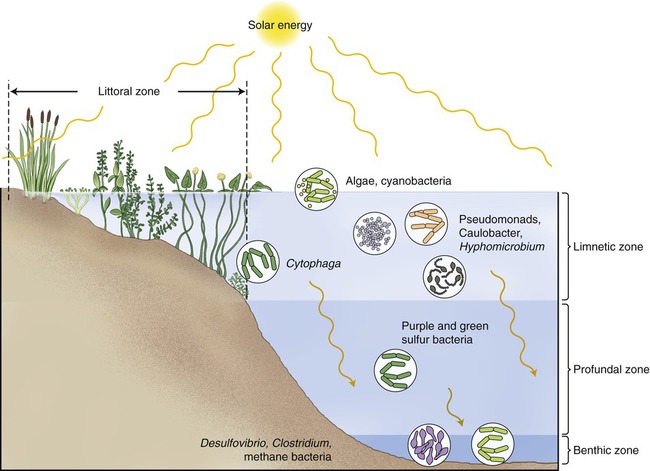
Four distinct vertical zones can be observed in lakes and ponds: a littoral zone, a limnetic zone, a profundal zone, and a benthic zone.
• The littoral zone (Figure 24.7) is the area along the shoreline with considerable rooted vegetation and good light source. This is the zone where nutrients enter the lake or pond, providing an abundance of food and promoting a high diversity of animals and bacteria in the biotope.
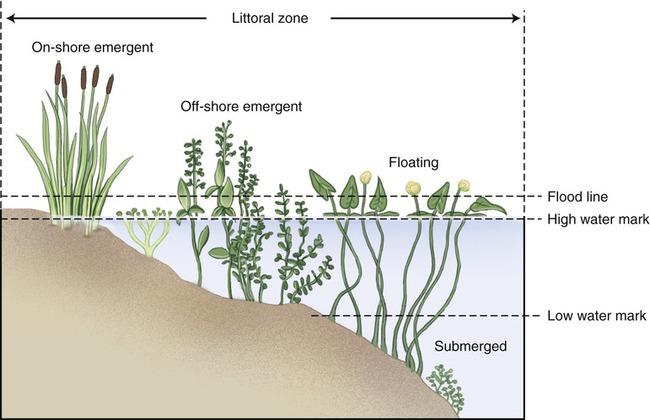
This area along the shoreline exhibits considerable rooted vegetation and good light source.
• The limnetic zone is the well-lit, upper layer of water away from the shore. This area is occupied by phytoplankton consisting of algae and cyanobacteria, and zooplankton, small crustaceans, and fish. Areas of the limnetic zone with sufficient oxygen supply also contain Pseudomonas spp., Cytophaga, Caulobacter, and Hyphomicrobium.
• The profundal zone is the deeper water located below the limnetic zone. It is characterized by limited light penetration (diffuse light), lower oxygen content, and lower temperature. The organisms of this zone depend on import of organic matter drifting down from the littoral and limnetic zones. This zone is inhabited mainly by primary consumers that are either attached to or crawl along the sediments at the bottom of the lake. These bottom-dwelling organisms are referred to as benthos. Purple and green sulfur bacteria can also be found in this zone.
• The benthic zone consists of the sediments at the bottom of a lake; it includes the sediment surface as well as some subsurface layers. This layer also contains benthos, which generally live in close relationship with the substrate bottom. These life forms can tolerate cool temperatures and low oxygen levels. Bacteria found in this zone include Desulfovibrio, methane-producing bacteria, and Clostridium species.
Marine Ecosystems
Marine (saltwater) ecosystems are part of the largest aquatic system on earth, covering more than 70% of the earth’s surface. Marine ecosystems host many different species ranging from planktonic organisms that comprise the base of the marine food web, to fish, and large marine mammals. These ecosystems have some unique qualities, specifically the presence of dissolved compounds, particularly salts. As in freshwater systems the oceans can also be divided into layers, but they do have a fifth zone, the abyssal zone, which encompasses the deep ocean trenches (Figure 24.8). The majority of marine microorganisms are present in the littoral zone, where light is readily available and nutrient levels are high. The upper 200 m of ocean contains an unseen population of microbes that supports oceanic life. These are photosynthetic organisms called the marine phytoplankton. The many kinds of different bacteria among the phytoplankton are a food source for protozoa, which in turn are prey for the multicellular organisms among the zooplankton. The zooplankton is a source of food for the fish. The carbon dioxide and mineral nutrients released as by-products of the metabolic activities of bacteria, protozoa, and zooplankton are recycled into the photosynthetic phytoplankton.
Natural Disasters
Floods
A flood is an overflowing of water onto land that is normally dry. When the volume of water within a body of water, such as a river or lake, surpasses the total capacity of the body, water will flow out of its perimeters, causing a flood. The flood of the Yellow River in 1931 is generally considered to be the deadliest natural disaster ever recorded, certainly in the twentieth century, discounting pandemic diseases such as the influenza pandemic of 1918. The estimated number of people killed in this flood is between 1 and 2 million. Flooding in more recent times is illustrated in Table 24.1.
TABLE 24.1
Lethal Flooding in the Years 2000 to 2008
Year | Location | Event | Estimated Death Toll |
2008 | United States | Midwest flooding | 17 |
2007 | China | Mountain torrents, mud-rock flows, landslide | 1029 |
2007 | Sudan, Nigeria, Burkina Faso, Ghana, Kenya, and other African countries | African Nations Flood | 353 |
2007 | Indonesia | Central and East Java torrential monsoon rain, flood | 119 |
2007 | United Kingdom | United Kingdom floods | 11 |
2006 | Ethiopia | Omo River delta, Dire Dawa, Tena, Gode, flash flood | 705 |
2006 | North Korea | North Korea flooding | 610 |
2005 | China | Fujian, Anhui, Zhejiang flood | 1624 |
2005 | India | Mumbai, Maharashtra, Kamataka, monsoon rain | 1503 |
2005 | United States | New Orleans dike failure | 70 |
2004 | India, Bangladesh | Eastern India, Bangladesh monsoon rain | 3076 |
2004 | Haiti, Dominican Republic | Spring flooding | 1605–3363 |
2003 | Indonesia | Sumatra flood, Jambi, Batanghari, Tondano, torrential rain, flash flood | 313 |
2002 | Nepal | Nepal flood, Makwanpur, torrential rain | 429 |
2001 | Algeria | Algiers, Bab El Oued | 827 |
2000 | Mozambique | Mozambique flood | 800 |
Waterborne Diseases
Waterborne diseases that can occur after floods include typhoid fever, cholera, leptospirosis, and hepatitis A (see Chapter 1 [Scope of Microbiology] and Chapter 12 [Infections of the Gastrointestinal System]). Although flooding is associated with an increased risk of infection, the risk is low unless there is a significant population displacement and/or major contamination of the water supply. Therefore a major risk factor for outbreaks associated with floods is the contamination of drinking water facilities. The risk can be minimized once the threat has been recognized and disaster response addresses the provision of safe drinking water as a priority.
Dead Humans and Animals
• Tuberculosis can be transmitted if the bacillus is in aerosol form due to residual air in the lungs exhaled, or from fluid from the lungs spurted up through nose and mouth during handling of the corpses.
• Blood-borne viruses can be acquired through direct contact with injured skin, blood, or body fluids, or from exposure to mucous membranes from splashing blood or body fluids.
• Gastrointestinal infections are more common because dead bodies generally leak fecal material. Transmission can occur via the fecal–oral route, by direct contact with the body, soiled clothing, or contaminated equipment. Gastrointestinal infections can also occur if the water supply has been contaminated due to large numbers of corpses.
Tsunamis
Most of the risks of infectious diseases are similar in river or lake floods and in tsunamis. Some of the more recent tsunamis are listed in Table 24.2.
TABLE 24.2
Tsunamis in the Twentieth and Twenty-first Centuries
Year | Location | Event (Specific Location) | Estimated Death Toll |
2007 | Solomon Islands | 2007 Solomon Islands earthquake | 52 |
2006 | Indonesia | Java earthquake | 540 |
2004 | Indian Ocean | Indian Ocean earthquake | >250,000 (sources vary) |
1998 | Papua New Guinea | 1998 Papua New Guinea earthquake (earthquake followed by undersea landslide) | 3,000 |
1993 | Japan | Okushiri | 202 |
1983 | Japan | Northern Honshu | 102 |
1979 | France | Nice | 23 |
1976 | The Philippines | Moro Gulf | 5,000 |
1964 | United States: Alaska and Hawaii | Good Friday earthquake | 131 |
1960 | Chile, United States (Hawaii), the Philippines, and Japan | Great Chilean earthquake | 2,000 |
1952 | Russia | Kuril Islands, Kamchatka Peninsula | 2,300 |
1946 | United States (Alaska and Hawaii) | Aleutian Island earthquake | 173 |
1944 | Japan | Tonankai | 1,223 |
1933 | Japan | Sanriku | 3,008 |
1929 | Canada (Newfoundland) | 1929 Grand Banks earthquake | 28 |
1908 | Italy | 1908 Messina earthquake | 70,000 |
Earthquakes
An earthquake, the result of a sudden release of the energy in the earth’s crust, produces seismic waves that can be measured with a seismometer (seismograph) indicating the strength of the quake. Strong earthquakes can have devastating results. Other than the material losses, the consequences may include a lack of basic necessities, loss of life, and disease. Infectious diseases that may occur after an earthquake include waterborne diseases due to an unsafe water supply, foodborne illnesses due to lack of refrigeration, vector-borne illnesses resulting from standing water, and any disease that might occur because of compromised sanitation and overcrowding of shelters. A listing of some recent earthquakes with lethality is provided in Table 24.3.
TABLE 24.3
Earthquakes in the Years 2001 to 2007
Year | Location | Event (Specific Location) | Estimated Death Toll |
2007 | Indonesia | March 6 earthquake (southern Sumatra) | 25 |
2007 | Peru | August 12 earthquake (near central coast) | 514 |
2007 | Solomon Islands | April 1 earthquake | 52 |
2007 | Indonesia | September 12 earthquake (southern Sumatra) | 67 |
2006 | Indonesia | May 2006 Java earthquake | 6,234 |
2005 | Pakistan and India | 2005 Kashmir earthquake | 87,350 |
2005 | Iran | Zarand | 564 |
2004 | Indian Ocean | Indian Ocean earthquake | >250,000 (sources vary) |
2004 | Morocco | Morocco earthquake | 571 |
2003 | Iran | Bam earthquake | 26,271 |
2003 | Algeria | 2003 Boumerdes earthquake | 2,266 |
2001 | India | Gujarat earthquake | 20,000 |
Bioterrorism
• Review potential general criteria for selecting the biological agents that pose the greatest threats to civilians
• Review lists of previously identified biological threat agents
• Identify those that should be further evaluated and prioritized for public health preparedness efforts
Category A Agents
• They are easily transmitted from person to person.
• They have a high mortality rate and the potential for a major public health impact.
• They have the potential to cause public panic and social disruption.
• They require special action for public health preparedness.
Bacterial Agents
Anthrax is caused by Bacillus anthracis, a gram-positive, spore-forming rod. It is a zoonotic disease that can cause three forms of anthrax: cutaneous, gastrointestinal, and respiratory (see section on inhalation anthrax in Chapter 11, Infections of the Respiratory System). Although all forms can lead to dangerous and potentially fatal infections, the forms that best lend themselves to use as bioweapons are the inhalation and cutaneous forms.
Inhalation anthrax is the most lethal form of anthrax. Beginning on September 18, 2001, letters containing anthrax spores were mailed to several news media offices and to two Democratic U.S. Senators (Figure 24.9). In this process, postal workers were also endangered. Among those who developed anthrax during this attack, all who presented the full symptoms of inhalation anthrax before antibiotic therapy was applied, died. The weaponized form of anthrax involved in both inhalation and cutaneous forms of the disease consists of spores that are typically maintained as a powder or are aerosolized. These spores are much more potent than those that occur naturally in the environment. The lethal dose for inhalation anthrax has now been reestimated as several hundred spores instead of earlier estimates of 2500 to 55,000.
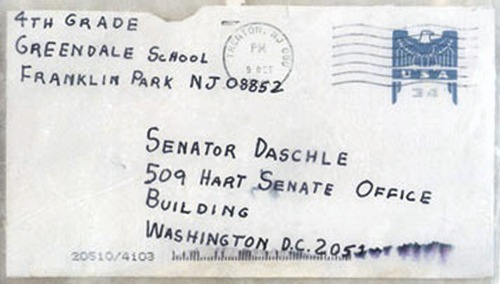
This letter contained anthrax spores capable of causing inhalation anthrax.
The infection process begins when the spores are inhaled into the lungs, where they reach the alveolar spaces. Most are destroyed by alveolar macrophages (Figure 24.10). Surviving spores can move into the lymphatic system and travel to the mediastinal lymph nodes to undergo germination into the vegetative bacillus form. Once germination begins toxins are released, causing both local and systemic symptoms. These symptoms include hemorrhagic damage to the lungs and a systemic inflammatory response. The severity of the infection is proportional to the level of toxins in the blood and not the number of bacteria in the blood culture. In other words, a culture negative for the bacterium does not necessarily indicate that the danger of anthrax does not exist. The symptoms of inhalation anthrax change with progression of the disease. Early symptoms and symptoms of late stages (2 to 3 d after the onset of the early stages) of the infection are illustrated in Box 24.2.
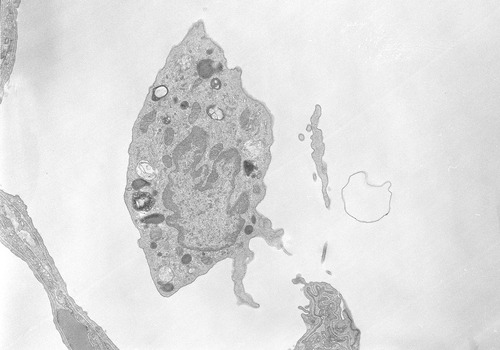
Cutaneous anthrax accounts for 95% of cases of the naturally occurring form of anthrax. Eleven of the 22 cases involved in the anthrax powder attacks after 9/11 were cutaneous forms of the disease. The infection results from spore contact with the skin, particularly in areas where there are abrasions or breaks in the skin. The mechanism of the infection is much the same as for the inhalation form, differing primarily on the tissue being affected. At the infection site, painless, pruritic, papular lesions will form within 1 week of exposure to the spores. Within 2 days fluid vesicles around the papules form, containing numerous bacilli and some white blood cells. These vesicles will enlarge and the site will become edematous (swollen with fluid). Eventually the lesions rupture and become a necrotic ulcer covered by a black eschar (Figure 24.11). Within 2 weeks the eschar will dry up and fall off. On occasion a patient may experience a secondary infection with Staphylococcus aureus if the organism is present at the lesion site. In rare cases a systemic infection may occur as a late manifestation of a cutaneous infection. This systemic infection can be manifested as bacteremia, possibly resulting in renal failure, anemia, bleeding, and ecchymoses.
Plague is caused by the bacterium Yersinia pestis, a gram-negative rod (see Chapter 14, Infections of the Circulatory System). The plague can take two forms, bubonic and pneumonic, with the latter having a higher mortality rate. One of the natural methods of transmission of the organism to humans is through the bite of infected fleas that have in turn become infected by biting infected rodents, the reservoirs of the pathogen. This infection route usually results in development of the bubonic form of plague. Another route of transmission is through the inhalation of aerosolized droplets containing the pathogen. In a bioterrorism attack using Y. pestis, the most likely means of transmission would be through aerosolization of the pathogen.
Deliberate infection of the natural animal reservoirs in densely populated areas is another possible but rather inefficient means of attempting to spread the plague. The incubation time for the Y. pestis organism is between 2 and 7 days. General symptoms for the pneumonic plague and advanced stage symptoms with sepsis are illustrated in Box 24.3.
The forms most likely to be encountered in a bioterrorism attack using aerosolized F. tularensis would be typhoidal, pneumonic, and oropharyngeal. Of these, the typhoidal form of tularemia would most likely be the dominant form of the disease encountered. Each of these forms has a specific set of clinical symptoms (Box 24.4). Typhoidal and pneumonic forms are the most dangerous, causing the highest mortality rates. These forms of tularemia are especially detrimental in persons with any impaired cellular immunity because this pathogen is an intracellular organism.
Diagnosis of tularemia includes microscopic examination of sputum or blood for the presence of this small coccobacillus. Standard biochemical tests are not always effective because the organism is rather fastidious and slow growing. No readily rapid test for detection is currently available. A more accurate diagnosis will require use of the polymerase chain reaction (PCR), fluorescent antibody testing, enzyme-linked immunosorbent assay (ELISA), and pulsed-field gel electrophoresis (see Chapter 25, Biotechnology) at BSL-2 or BSL-3 laboratories (see Chapter 5, Safety Issues).
Botulism is the result of a potent toxin produced by Clostridium botulinum, a gram-positive, spore-forming, anaerobic bacillus (Figure 24.12) (see Chapter 13, Infections of the Nervous System and the Senses). The botulinum toxin is the most potent natural neurotoxin in existence and if dispersed ideally a single gram of this toxin could kill over 1 million people! Botulism is distinct among the Category A agents in that it is the toxin and not the bacterium itself that causes the disease. The means of natural transmission of the organism include consuming contaminated food or to a lesser extent water, and wound-site contact with the organism. The bacterium cannot penetrate through intact skin but can be absorbed through the mucosal linings of the gastrointestinal or respiratory tracts.
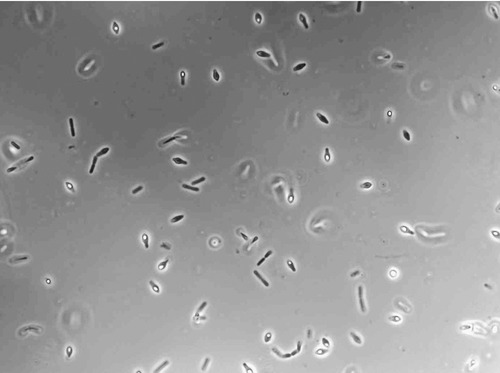
C. botulinum is a gram-positive, spore-forming, anaerobic bacillus, and the causative agent of botulism. (Courtesy K.M. Marshall, Eric Johnson Lab, University of Wisconsin, Madison.)
In a bioterrorism attack the toxin or organism would most likely be transmitted in an aerosol form, or through contamination of food and water. A number of factors about the toxin limit its effectiveness as a bioweapon. The toxin can be denatured when food is properly cooked. The chlorine levels used in most water treatment plants are also sufficient to denature the toxin, rendering it harmless if used in food or water supplies. Furthermore, the fact that the cells and any aerosolized toxin can be filtered from the air by means of a couple of layers of cloth, and that the toxin itself is denatured by sunlight within 1 to 3 hours of exposure, limits its use in aerosol form. These limitations can be somewhat mitigated by using enormous quantities of the toxin but especially in the case of water supply contamination, the botulinum toxin would be an impractical bioweapon. As previously discussed in Chapter 13 (Infections of the Nervous System and Senses), the botulinum toxin causes a number of symptoms usually associated with the nervous system, with the final stage involving suffocation due to paralysis of the respiratory muscles.
Viral Agents
Viruses are true parasites that cannot live on their own; they need a host cell to reproduce and then cause disease (see Chapter 7, Viruses). Although viruses cannot be grown on a plate or in a test tube, their genomes are generally smaller than bacterial genomes, making them easier to manipulate (see Chapter 25, Biotechnology). Because of the available technology, an increasing commercial exploitation of genetic engineering in both agriculture and medicine has occurred. This trend also may have the potential to create viruses and bacteria that are more virulent than nature’s worst case. The production of synthetic viruses with the ability to multiply by the millions represents a bioterrorism threat as great as that with natural occurring viruses, or maybe even more.
In 1980 the World Health Organization (WHO, Geneva, Switzerland) declared the smallpox virus to be eradicated as a result of a global vaccination program (see Chapter 7, Why You Need to Know and Life Application: Smallpox as Biological Weapon). The smallpox virus is usually inhaled, then taken up by macrophages and transported to lymph nodes where the virus multiplies. This viral increase activates T and B cells and produces a host antibody response (see Chapter 20, The Immune System). Within about 4 days viremia develops and the virus spreads to the spleen, bone marrow, and other lymph nodes. As the infection proceeds the virus infects the dermis and oropharynx, causing the classic skin lesions associated with smallpox. The lesions in the oropharyngeal region form quickly, resulting in the saliva of this region containing large amounts of the virus. Transmission readily occurs through contact with skin lesions before they scab over, or via aerosolized droplets from the oropharynx. Infection can also occur through contact with objects contaminated with the virus including clothes, bedding, and body fluids such as urine, sweat, or sputum.
Symptoms of a smallpox infection include the following:
• Vesicular or pustular poxlike rash: Rash begins at the mouth and face and moves outward to the arms and legs
Viral hemorrhagic fevers (VHFs) are caused by four families of RNA viruses:
1. Filoviridae: Ebola and Marburg viruses
2. Arenaviridae: Machupo and Lassa viruses
3. Bunyaviridae: Congo-Crimean hemorrhagic fever virus, Rift Valley virus
With the exception of the filoviruses, all these viruses are zoonotic. The pathogenic mechanism of VHFs is not fully understood; however, all the families display similar symptoms, suggesting similar mechanisms. Several mechanisms including platelet deficiency, direct endothelial and platelet injury, and cytokine dysregulation have been identified and vary somewhat depending on the family. These mechanisms account for the significant bleeding common to all four viral families. Because of the high mortality rate and dangers inherent in working with these viruses, testing for VHFs must be done at a BSL-4 facility (see Chapter 5, Safety Issues).
Diagnosis of a specific viral infection is difficult. Healthcare professionals must often rely on patient history and clinical symptoms to determine the possibility of VHF. Nasal, stool, serum, or almost any body fluid of an infected individual will contain the virus and should be sent to a designated BSL-4 facility for definitive diagnosis. These laboratories will then use ELISA, PCR, and viral isolation to confirm a preliminary diagnosis. Through electron microscopy the presence of specific viruses can also be observed. Ebola virus is easily identified by its unique “shepherd’s hook” shape (Figure 24.13).
HEALTHCARE APPLICATION
Category A Agents/Diseases
Disease | Organism | Transmission (as Bioweapon) | Treatment |
Bacterial | |||
Anthrax | Bacillus anthracis | Primarily as aerosol/powder | Ciprofloxacin, doxycycline |
Plague | Yersinia pestis | Aerosolized droplets | Streptomycin, gentamicin, doxycycline, ciprofloxacin |
Tularemia | Francisella tularensis | Aerosolized droplets | Streptomycin, gentamicin, doxycycline, ciprofloxacin |
Botulism | Clostridium botulinum | Toxin dispersed in aerosolized form, also as a food or water contaminant | Botulism antitoxin, supportive measures |
Viral | |||
Smallpox | Variola major virus | Initially dispersed as aerosol, followed by spread through contact | Prevention: Vaccine Treatment: Cidofovir (antiretroviral drug) is being studied |
Viral hemorrhagic fevers (VHFs) | Filoviridae: Ebola virus and Marburg virus | Aerosol and person-to-person contact | Supportive care |
Arenaviridae: Machupo virus and Lassa virus | Aerosol | Supportive care, ribavirin | |
Bunyaviridae: Rift Valley virus and Congo-Crimean fever virus | Aerosol | Supportive care, ribavirin | |
Flaviviridae: Dengue fever virus and yellow fever virus | Aerosol | Supportive care |
Category B Agents
• Brucellosis (Brucella species)
• Epsilon toxin from Clostridium perfringens
• Staphylococcus enterotoxin B
• Psittacosis (Chlamydia psittaci)
• Glanders (Burkholderia mallei)
• Melioidosis (Burkholderia pseudomallei)
• Ricin toxin from Ricinus communis (castor bean): Sometimes included as a Category A agent
• Typhus fever (Rickettsia prowazekii)
• Venezuelan, Eastern, and Western equine encephalitis (alphaviruses)
2. Pulmonary: Myalgias, headache, chest pain
3. Septic: Sepsis, possible CNS involvement, associated with a high mortality rate
HEALTHCARE APPLICATION
Category B Agents/Diseases
Disease | Organism | Transmission (as Bioweapon) | Treatment |
Bacterial | |||
Brucellosis | Brucella species | Aerosol | Doxycycline and rifampin |
Gastrointestinal, inhalation, systemic infection | Clostridium perfringens | Aerosol, food or water contamination | Supportive care |
Salmonellosis | Salmonella species | Aerosol, food contamination | Supportive care |
Food poisoning/sepsis | Escherichia coli O157:H7 | Food, water contamination | Supportive care |
Shigellosis | Shigella species | Food, water contamination | Supportive care |
Staphylococcal gastroenteritis | Staphylococcus aureus | Aerosol, food contamination | Supportive care |
Cholera | Vibrio cholerae | Food, water contamination | Doxycycline, trimethoprim-sulfamethoxazole, furazolidone (for pregnant women) |
Psittacosis | Chlamydia psittaci | Aerosol | Tetracycline, doxycycline |
Glanders | Burkholderia mallei | Aerosol | Amoxicillin, tetracycline |
Melioidosis | Burkholderia pseudomallei | Aerosol | Amoxicillin, tetracycline |
Q fever | Coxiella burnetii | Aerosol | Doxycycline |
Plant toxin | |||
Ricin toxin | Derived from castor bean plant (Ricinus communis) | Aerosol, food or water contamination, injection | Supportive care |
Viral | |||
Typhus fever | Rickettsia prowazekii | Aerosol | Tetracycline, doxycycline |
Alphavirus encephalitides | Alphaviruses | Aerosol | Supportive care |
Cryptosporidiosis | Cryptosporidium parvum | Food, water contamination | Supportive care |
Category C Agents
There are a number of agents that could fall into the category C grouping, including newly emerging infectious diseases (see Chapter 18, Emerging Infectious Diseases). Hantavirus and Nipah virus are representatives of the types of agents that may be considered category C agents. Hantavirus is discussed in Chapter 9 (Infection and Disease) and Chapter 11 (Infections of the Respiratory System) and Nipah virus is briefly covered in Chapter 7 (Viruses). It is anticipated that as bioweapons both of these agents would be transmitted in an aerosolized form. Typically, symptoms for infection by these agents would include the following:
HEALTHCARE APPLICATION
Category C Agents/Diseases
Disease | Organism | Transmission | Treatment |
Hantavirus pulmonary syndrome | Hantavirus (Family Bunyaviridae) | Aerosol | Supportive care |
Nipah virus encephalitis | Nipah virus (Family Paramyxoviridae) | Aerosol | Supportive care |
Role of First Responders in Bioterrorism
• Procedures for handling mail and parcels
• Communications protocols within the institution, and with other local/state emergency response agencies
• Communications with and reporting to local/state departments of health and clinical laboratory facilities
• Communications with and reporting to the Federal Bureau of Investigation (FBI), the Department of Homeland Security, and the CDC
First responders will also need to be knowledgeable about the requirements for and the proper use and disposal of personal protective equipment that may be needed to respond to a bioweapons attack (see Chapter 5, Safety Issues). Special attention should be given to respiratory and dermal protection as these will be the primary transmission routes for many bioterrorism agents.
• Hazardous Waste Operations and Emergency Response (OSHA 1910.120)
• Hazardous Communication Standard (OSHA 1910.1200)
• Respiratory Protection Standard (OSHA 1910.134)
• Personal Protective Equipment (OSHA 1910.132)
• Maintenance, Safeguards and Operational Features for Exit Routes (OSHA 1910.37)
In addition to healthcare facilities that will be treating the victims of a bioterrorism, there is also a critical need to quickly identify and evaluate the biological agents used in an attack. Many facilities will not have the equipment, facilities, or expertise to conduct advanced diagnostic testing. In 1999 the federal government created the Laboratory Response Network (LRN) to integrate the efforts of numerous designated clinical laboratories to facilitate the surveillance, detection, identification, and advanced testing of suspected bioterrorism agents. This network is made up of more than 140 government-supported laboratories located in all 50 states as well as in other nations such as Britain, Canada, and Australia. Samples from hospitals and local facilities will be sent to a laboratory in the LRN or evaluated at the facility according to protocols for handling bioterrorism agents as established by the CDC, based on the biosafety level (see Chapter 5, Safety Issues) required to handle the suspected agent.
Summary
• The study of the relationships of microorganisms in their natural environments is referred to as microbial ecology. The levels of these relationships are as follows: populations, guilds, communities, microhabitats, habitats, ecosystems, and finally biospheres.
• Biogeochemical cycles are circuits or pathways through which organic compounds or elements are changed from one chemical state to another, by moving through biotic and abiotic compartments of the ecosystem.
• The biogeochemical cycles include the carbon cycle, the nitrogen cycle, the sulfur cycle, and the phosphorus cycle.
• Soil microbiology deals with the microbial abundance and the various microbial populations in the soil. It is dependent on moisture content, oxygen concentration, pH, and temperature of the soil.
• Water occupies nearly three fourths of the earth’s surface and aquatic microbiology deals with the study of microorganisms and their activities in freshwater and marine environments.
• Natural disasters such as floods, tsunamis, earthquakes, hurricanes, and others pose a potential threat of infectious diseases due to contaminated water and food. Vector-borne diseases due to natural disasters pose another problem in affected areas.
• The use of biological agents to incapacitate or kill civilians and military personnel is categorized as biological warfare and/or bioterrorism.
• On the basis of potency and various chemical and biological criteria, agents that can be used in biological warfare and/bioterrorism are placed in Category A, B, or C.
• Healthcare facilities are critical in the initial identification and isolation of suspected biological agents.
• The actions of first responders in natural disasters and bioterrorism are regulated and guided by federal, state, and local agencies.
Review Questions
1. A set of guilds is referred to as:
2. All of the following are free-living, nitrogen-fixing bacteria except:
3. Which of the following is not found in the atmosphere?
4. Which of the following zones is present only in oceans?
5. Most marine microorganisms are present in the:
6. Which of the following is a category A agent?
8. Which of the following is the fourth most abundant element in the universe?
9. The process by which nitrate is reduced to nitrogen is called
10. Eutrophication is a term used in the:
11. A region or regions of the earth populated by living organisms is referred to as __________.
12. The physical location where organisms are found is called a(n) __________.
13. The conversion of CO2 to organic molecules is called __________.
14. Cholera is considered a Category __________ disease.
15. Rhizobium is involved in the __________ cycle.
16. Describe the sulfur cycle.
17. Name the U.S. agency designated as lead agency in overall public health planning in response to bioterrorism and list the activities for which it is responsible.
18. Name and describe the four vertical layers that can be found in lakes.
19. Name and briefly describe the public health concerns regarding disease transmission after a flood.
20. Name and explain the three different categories of agents that could be involved in bioterrorism.