Microorganisms in the Environment and Environmental Safety
After reading this chapter, the student will be able to:
• Discuss microbial ecology and describe the levels of microbial relationships within the environment
• Discuss the importance of biogeochemical cycles, including the carbon, nitrogen, sulfur, and phosphorus cycles
• Discuss soil microbiology, with emphasis on the abundance and type of microbes in the soil
• Discuss aquatic microbiology, both freshwater and marine environments, and include the zones observable in these bodies of water
• Describe the characteristics of natural disasters including floods, tsunamis, earthquakes, and hurricanes
• Illustrate the most common infections/diseases associated with natural disasters
• Explain the concepts of biological warfare and bioterrorism
• Describe the initiatives taken to advance national public health capabilities in response to bioterrorism
• Define and describe the three groups of agents that are of concern in bioterrorism
Normal Environmental Conditions
Microbial Ecology
The study of the interrelationships of microorganisms in their natural environments is referred to as microbial ecology. Terms used to describe the levels of microbial relationships in the environment are as follows (Figure 24.1):
• Populations are formed by individual organisms through growth and reproduction.
• Guilds are populations of microorganisms that perform linked metabolic activities.
• Communities are sets of guilds and are characteristically heterogeneous. On occasion, especially in extreme environments, a community can consist of a single population.
• Microhabitats are specific small niches in which populations and guilds within a community reside because of optimal conditions for survival.
• Habitats are physical locations where organisms are found and they consist of groups of microhabitats. Within habitats the microorganisms interact with larger organisms.
• Ecosystems consist of organisms, their abiotic environment, and the relationships between them.
• Biospheres are regions of the earth populated by living organisms.
Thriving ecosystems are able to support great biodiversity, but many microbes live in harsh ecosystems where nutrients are limited. Therefore, in order to survive, these microbes must have special adaptation to a given environment. Environments may cycle between excess nutrient availability and depletion. To survive, some microorganisms form biofilms (see Chapter 6, Bacteria and Archaea) on surfaces where nutrients accumulate. Biodiversity is controlled by competition between the microbes, but usually an ecosystem is heterogenic. Microbes often use the waste products of other organisms for their own metabolic activities; in other cases the waste products of one type of microbe may make the environment more favorable for another. The formation of biofilms serves as a good example of this phenomenon.
Biodiversity ensures a healthy interaction between life forms on earth. This complex web of life is essential for the maintenance of sufficient food and freshwater. The United States Environmental Protection Agency (EPA) and other national and international organizations recognize the importance of biodiversity for human health and well-being. The EPA in partnership with other organizations implemented the biodiversity-human health project (http://epa.gov/ncer/biodiversity/index.html). One focus of the project is to better understand the relationship of emerging infectious diseases (see Chapter 18, Emerging Infectious Diseases), changing environments, and factors that manipulate biodiversity.
Biogeochemical Cycles and Microbes
A biogeochemical cycle is a circuit or pathway through which organic compounds or a chemical element is changed from one chemical state to another by moving through both biotic and abiotic compartments of an ecosystem (Box 24.1). The study of these chemical transformations is called biogeochemistry.
The Carbon Cycle
Carbon is the fourth most abundant element in the universe, and essential to life on earth. Carbon is the element that provides the backbone of all organic molecules (see Chapter 2, Chemistry of Life). All living organisms from microorganisms, to plants and animals, contain a large number of organic compounds and therefore a large amount of carbon. Thus the carbon cycle is considered to be the primary biogeochemical cycle.
The next step in the carbon cycle involves the consumption of autotrophs by chemoheterotrophs such as animals and protozoans, which then catabolize the organic molecules for energy, resulting in the release of CO2. Some organic molecules produced by the autotrophs may be incorporated into the tissues of heterotrophs, where they remain until that organism dies. Dead organisms are decomposed with the help of microorganisms, metabolizing organic molecules and releasing CO2 in the process. The release of CO2 into the atmosphere starts the cycle over again (Figure 24.2).
The Nitrogen Cycle
Nitrogen gas is the most abundant gas in the earth’s atmosphere, representing about 79% of all the gases in air. Unfortunately, the nitrogen in the atmosphere is unusable by most organisms. Only about 0.03% of the earth’s nitrogen is fixed in the form of nitrates, nitrites, ammonium ion, and organic compounds. Nitrogen is necessary for all living organisms for the synthesis of proteins, nucleic acids, and other nitrogen-containing compounds. The nitrogen cycle consists of several steps as illustrated in Figure 24.3.
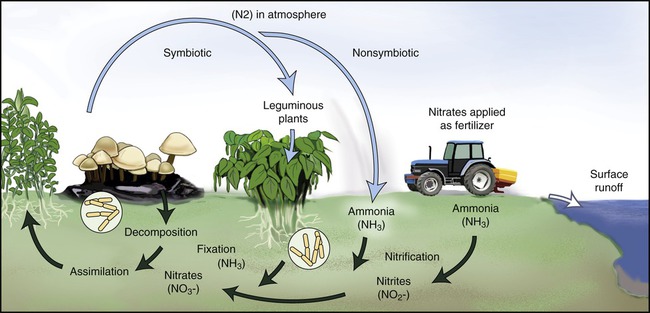
Nitrogen is also an essential element for living organisms. Nitrogen from the atmosphere goes through fixation, nitrification, and denitrification and absorption by living organisms. This is then followed by decomposition, ammonification, and then nitrification again.
Anammox is the acronym for anaerobic ammonium oxidation and is a recent addition to knowledge of the nitrogen cycle. In this process, performed by anammox bacteria (previously thought to be denitrifying bacteria), nitrite and ammonium are directly converted to dinitrogen gas. This process accounts for up to 50% of the dinitrogen gas produced in the oceans. Ammonia is the major source of fixed nitrogen in the oceans and because the anammox bacteria remove ammonia efficiently from those bodies of water, these organisms limit oceanic primary productivity. On the positive side, the ability of anammox bacteria to convert ammonium and nitrite directly to dinitrogen gas provides a promising alternative to current methods of removal of nitrogen from wastewater (see Life Application: Anammox and Wastewater Treatment).
The Sulfur Cycle
The sulfur cycle is similar to the nitrogen cycle, except that sulfur originates from natural sedimentary deposits. The sulfur cycle includes both atmospheric and terrestrial processes (Figure 24.4). The terrestrial process begins with the weathering of rocks, resulting in the release of stored sulfur. Subsequently, the sulfur comes in contact with air and is then converted into sulfate (SO4). Plants and microorganisms will take up the sulfate and convert it into organic forms. Animals will consume the organic forms via food they consume, thereby moving the sulfur through the food chain. As organisms die, bacteria decompose them, releasing sulfur-containing amino acids into the environment. The sulfur released from the amino acids is converted by microorganisms to its most reduced form, hydrogen sulfide (H2S). This process is called sulfur dissimulation. H2S is then oxidized to elemental sulfur and then to sulfate by various microorganisms under various conditions. These organisms include nonphotosynthetic autotrophs such as Thiobacillus and Beggiatoa and photoautotrophic green and purple sulfur bacteria. The cycle starts again with the uptake of sulfate by plants and algae that animals eat. Anaerobic respiration by the bacterium Desulfovibrio reduces sulfate back to H2S.
The Phosphorus Cycle
Phosphorus is an essential nutrient for plants, animals, and microorganisms. It is part of nucleic acids (DNA, RNA, ATP, and ADP) and of the plasma membranes (phospholipids). Phosphorus can be found in water, soil, and sediments. Unlike nitrogen and sulfur, phosphorus cannot be found in the atmosphere in the gaseous state. Phosphorus exists in the form of phosphate ion and undergoes little change in its oxidation state. The phosphorus cycle (Figure 24.5) involves changes of phosphorus from insoluble to soluble forms that are available for uptake by organisms, and from organic to inorganic forms by pH-dependent processes. Because no gaseous form of phosphorus exists it has the tendency to accumulate in the seas and other bodies of water.
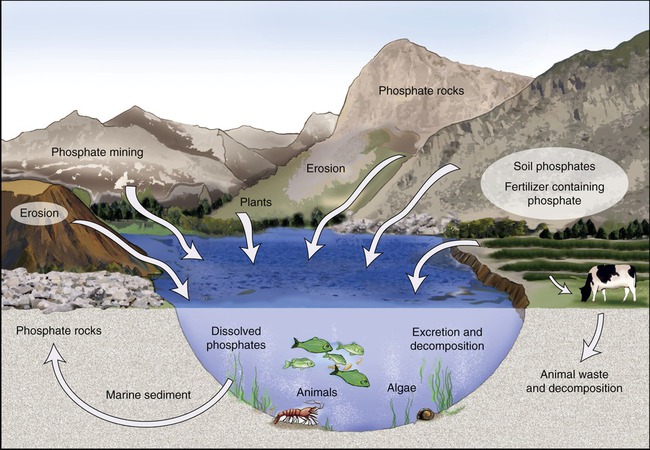
The phosphorus cycle involves changes of phosphorus from insoluble to soluble forms that are available for uptake by organisms, and from organic to inorganic forms by pH-dependent processes. Because no gaseous form of phosphorus exists it has the tendency to accumulate in the seas and other bodies of water.
Soil Microbiology
Soil composition such as organic matter, soil structure, nutrient content, nutrient cycling, nutrient availability, and water-holding capacity are influenced by, or dependent on, billions of organisms, microscopic ones as well as fairly large insects and earthworms. All these organisms together form a pulsating, living community in soil. Soil microorganisms can be classified into major groups such as microarthropods, nematodes, protozoa, fungi, algae, and bacteria. The most numerous organisms in the soil are bacteria—each gram of typical soil contains millions of bacteria. The population of bacteria is largest in the top few centimeters of soil and declines rapidly with depth. Organic matter is metabolized by microbes in the soil, and via the biogeochemical cycles, elements are oxidized and reduced (see Chapter 2, Chemistry of Life, for oxidation/reduction reactions) by microorganisms to meet their metabolic needs.
• Moisture is essential for the survival of microorganisms. In dry soil microbes will exhibit lower metabolic activity, they will be less diverse, and lower numbers will be present than in moist soil.
• Oxygen dissolves poorly in water; therefore, moist soils are lower in oxygen content than dry soils. Waterlogged soil will result in a decline in microbial diversity and anaerobic organisms will dominate. The presence or absence of rainwater determines moisture, and thus dissolved oxygen.
• The pH of soil influences the type of organisms that predominate. It will influence whether the soil is rich in bacteria or rich in fungi. In highly acidic or highly basic soils fungi will predominate, whereas bacteria prefer soil with a pH of about 7.
• The temperature of soil determines the types of microbe that can flourish. Most soil organisms are mesophiles and grow well in areas where winters and summers are not too extreme. Psychrophiles grow only in cold environments and cannot survive in areas that experience spring thawing. Thermophiles, on the other hand, grow only in warm environments where winters are not harsh.
• Nutrient availability also affects the microbial abundance and diversity of soil. The majority of soil microbes are heterotrophic and utilize the organic matter present in the soil. It is the amount of organic material rather than the kind of organic material that determines the abundance of microorganisms in a microbial community. A continuous influx of organic material (i.e., agricultural land) will support a wider assortment of microorganisms than soil with low amounts of organic material.
Microbial Populations in Soil
HEALTHCARE APPLICATION
Examples of Soil-borne Diseases
Microorganism | Disease |
Bacillus anthracis | Anthrax |
Clostridium tetani | Tetanus |
Hantavirus | Hantavirus pulmonary syndrome |
Histoplasma capsulatum | Histoplasmosis |
Blastomyces dermatitidis | Blastomycosis |
Sporothrix schenckii | Sporotrichosis |
Aquatic Microbiology
Freshwater Ecosystems
About 3% of the world’s water is freshwater, 99% of which is either frozen in glaciers and pack ice, or is buried in aquifers. The remainder of the freshwater is found in lakes, ponds, rivers, and streams. Microorganisms are dispersed vertically within lake and pond systems, according to the availability of oxygen, light intensity, and temperature. Surface waters are higher in oxygen and light intensity, and warmer than deeper waters. In contrast to stagnant waters where oxygen is readily depleted, in larger lakes the wave action continuously mixes nutrients, oxygen, and organisms, resulting in efficient use of its resources. In stagnant waters, because of the depletion of oxygen, anaerobic organism will dominate and a lesser water quality will be the result. Four distinct vertical zones can be observed in lakes and ponds (Figure 24.6): a littoral zone, a limnetic zone, a profundal zone, and a benthic zone:
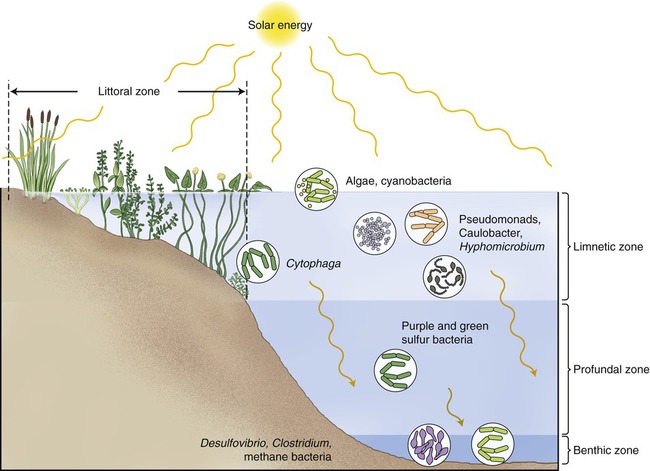
Four distinct vertical zones can be observed in lakes and ponds: a littoral zone, a limnetic zone, a profundal zone, and a benthic zone.
• The littoral zone (Figure 24.7) is the area along the shoreline with considerable rooted vegetation and good light source. This is the zone where nutrients enter the lake or pond, providing an abundance of food and promoting a high diversity of animals and bacteria in the biotope.
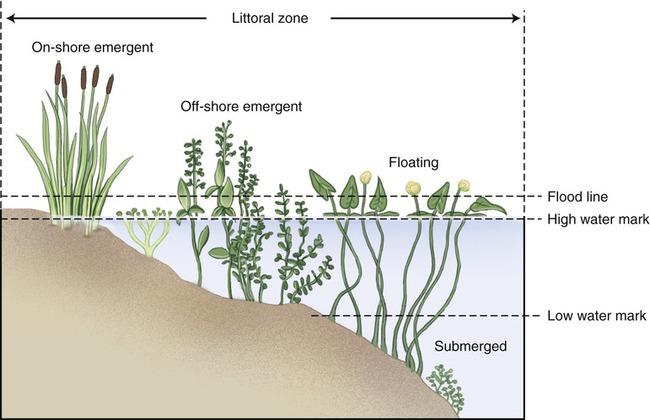
This area along the shoreline exhibits considerable rooted vegetation and good light source.
• The limnetic zone is the well-lit, upper layer of water away from the shore. This area is occupied by phytoplankton consisting of algae and cyanobacteria, and zooplankton, small crustaceans, and fish. Areas of the limnetic zone with sufficient oxygen supply also contain Pseudomonas spp., Cytophaga, Caulobacter, and Hyphomicrobium.
• The profundal zone is the deeper water located below the limnetic zone. It is characterized by limited light penetration (diffuse light), lower oxygen content, and lower temperature. The organisms of this zone depend on import of organic matter drifting down from the littoral and limnetic zones. This zone is inhabited mainly by primary consumers that are either attached to or crawl along the sediments at the bottom of the lake. These bottom-dwelling organisms are referred to as benthos. Purple and green sulfur bacteria can also be found in this zone.
• The benthic zone consists of the sediments at the bottom of a lake; it includes the sediment surface as well as some subsurface layers. This layer also contains benthos, which generally live in close relationship with the substrate bottom. These life forms can tolerate cool temperatures and low oxygen levels. Bacteria found in this zone include Desulfovibrio, methane-producing bacteria, and Clostridium species.
Marine Ecosystems
Marine (saltwater) ecosystems are part of the largest aquatic system on earth, covering more than 70% of the earth’s surface. Marine ecosystems host many different species ranging from planktonic organisms that comprise the base of the marine food web, to fish, and large marine mammals. These ecosystems have some unique qualities, specifically the presence of dissolved compounds, particularly salts. As in freshwater systems the oceans can also be divided into layers, but they do have a fifth zone, the abyssal zone, which encompasses the deep ocean trenches (Figure 24.8). The majority of marine microorganisms are present in the littoral zone, where light is readily available and nutrient levels are high. The upper 200 m of ocean contains an unseen population of microbes that supports oceanic life. These are photosynthetic organisms called the marine phytoplankton. The many kinds of different bacteria among the phytoplankton are a food source for protozoa, which in turn are prey for the multicellular organisms among the zooplankton. The zooplankton is a source of food for the fish. The carbon dioxide and mineral nutrients released as by-products of the metabolic activities of bacteria, protozoa, and zooplankton are recycled into the photosynthetic phytoplankton.
Natural Disasters
Floods
A flood is an overflowing of water onto land that is normally dry. When the volume of water within a body of water, such as a river or lake, surpasses the total capacity of the body, water will flow out of its perimeters, causing a flood. The flood of the Yellow River in 1931 is generally considered to be the deadliest natural disaster ever recorded, certainly in the twentieth century, discounting pandemic diseases such as the influenza pandemic of 1918. The estimated number of people killed in this flood is between 1 and 2 million. Flooding in more recent times is illustrated in Table 24.1.
TABLE 24.1
Lethal Flooding in the Years 2000 to 2008
Year | Location | Event | Estimated Death Toll |
2008 | United States | Midwest flooding | 17 |
2007 | China | Mountain torrents, mud-rock flows, landslide | 1029 |
2007 | Sudan, Nigeria, Burkina Faso, Ghana, Kenya, and other African countries | African Nations Flood | 353 |
2007 | Indonesia |