Chapter 22 Mechanical Cardiac Support
INTRAAORTIC BALLOON COUNTERPULSATION
Intraaortic balloon counterpulsation (IABC) is indicated for the short-term support of patients with severe myocardial ischemia or left ventricular systolic failure. Occasionally, the device may be used for longer than a week, but the incidence of complications increases when use extends beyond 48 hours.1 The insertion and removal of and the complications involved with intraaortic balloon pumps (catheters; IABPs) are described in Chapter 40.
Equipment and Physiology
The IABP has two lumens: one for the inflation and deflation of the balloon with helium and one for the measurement of aortic pressure. The catheter is connected to an external console that has a pneumatic pump, a microprocessor for timing inflation and deflation, and a screen that continuously displays the electrocardiogram (ECG), arterial blood pressure, and balloon pressure. The ECG is obtained from electrodes attached to the patient or by “slaving” the ECG from the patient monitor. The balloon is inflated during diastole and deflated just prior to ventricular ejection (Fig. 22-1).
The effects of IABC are twofold. First, because the majority of coronary blood flow to the left ventricle occurs in diastole, balloon inflation at this time increases diastolic aortic pressure and augments coronary perfusion, increasing myocardial oxygen delivery. Second, by deflating just prior to ventricular ejection, aortic end-diastolic pressure is decreased, which leads to a reduction in left ventricular systolic wall tension (and therefore afterload). This augments cardiac output and reduces myocardial oxygen consumption. The diastolic pressure-time index (the area under the diastolic aortic pressure-time curve), which reflects oxygen supply, increases and the systolic time-tension index (the area under the systolic aortic pressure-time curve), which reflects oxygen consumption, is reduced. Thus, IABC improves the balance between myocardial oxygen demand and supply, improves cardiac performance, and reduces left atrial pressure (Table 22-1).
Table 22-1 Physiologic Effects of Intraaortic Balloon Counterpulsation
Coronary blood flow | ↑ |
Pulmonary capillary wedge pressure | ↓ |
Mean diastolic pressure | ↑ |
Mean systolic pressure | ↓ |
Mean arterial pressure | ↓ |
Stroke volume and cardiac output | ↓ |
Left ventricular afterload | ↓ |
Heart rate | ↓ |
Indications
The main indications for IABC are listed in Table 22-2.2
Table 22-2 Principal Indications for Intraaortic Balloon Counterpulsation
Cardiogenic shock | 27.3% |
Support for high-risk cardiac catheterization | 27.2% |
Mechanical complications of myocardial infarction | 11.7% |
Preoperative support for high-risk cardiac surgery | 11.2% |
Postmyocardial infarction unstable angina | 10.0% |
Weaning from cardiopulmonary bypass | 4.8% |
Refractory left ventricular failure | 4.5% |
Refractory ventricular arrhythmias | 1.3% |
Cardiac support during noncardiac surgery | 0.5% |
Other indication or not recorded | 1.5% |
From Stone GW, Ohman EM, Miller MF, et al: Contemporary utilization and outcomes of intra-aortic balloon counterpulsation in acute myocar dial infarction: the benchmark registry. J Am Coll Cardiol 41:1940-1945, 2003.
Cardiac Surgery
IABC is widely used to assist weaning from cardiopulmonary bypass (CPB) and to treat hemodynamic instability after surgery (see Chapter 20). IABC may be used after all types of cardiac surgery, but the greatest benefit appears to be in patients undergoing isolated coronary artery bypass graft (CABG) surgery or cardiac transplantation.3 For high-risk patients undergoing CABG surgery, placement of an IABP 1 to 2 hours prior to surgery is associated with reduced mortality rates and shorter stays in the intensive care unit (ICU) and in the hospital compared with patients with undergoing postoperative insertion of an IABP.4–7 Preoperative insertion of an IABP is recommended in patients undergoing CABG surgery who have acute ischemia or an ejection fraction less than 25%.8 Preoperative insertion should also be considered for patients with hemodynamic instability or pulmonary edema secondary to acute mitral valve dysfunction and ventricular septal rupture.
Cardiogenic Shock Secondary to Myocardial Infarction
Cardiogenic shock due to predominant left ventricular failure complicating myocardial infarction is a widely accepted indication for IABC, but there are few data supporting this strategy. Data from the SHOCK trial registry (see Chapter 19) suggest that the use of IABC in combination with revascularization is associated with improved outcome compared to standard medical therapy.9
Myocardial Infarction and Ischemia
IABC is indicated for patients with unstable angina despite maximal medical therapy and for refractory angina following myocardial infarction, even in the absence of hemodynamic instability. Some data show that the routine application of IABC for 24 to 48 hours following successful percutaneous coronary intervention for acute myocardial infarction reduces the incidence of reocclusion of the infarct-related artery and decreases adverse events.10,11 However, this has not been a consistent finding12 and, in the absence of ongoing myocardial ischemia or cardiogenic shock, routine use of IABC after percutaneous coronary intervention is not indicated.
Right Ventricular Dysfunction
IABC is indicated primarily for left ventricular systolic dysfunction, but in patients with right ventricular dysfunction following heart transplantation it produces a substantial improvement in cardiac output and mean arterial pressure and a reduction in central venous pressure (CVP) and pulmonary artery wedge pressure (PAWP).13
Contraindications
Absolute contraindications to IABPs are severe aortic regurgitation, aortic dissection, severe (mobile) atheroma in the descending thoracic aorta, and occlusive vascular disease involving the distal aorta or the femoral or iliac arteries. Minimal diastolic augmentation is obtained in patients with low systemic vascular resistance or excessive diastolic aortic runoff due to systemic-to-pulmonary (e.g., patent ductus arteriosus) or arteriovenous shunts; however, these states are not contraindications. The severity of any dynamic left ventricular outflow tract obstruction will be made worse by IABC because of reduced left ventricular afterload.
IABP Controls
Triggering and Timing
The most useful trigger for balloon deflation is the R wave of the ECG because this signal is usually reliably identified by the device and is coincident with the onset of ventricular systole (see Fig. 1-3). If ECG triggering is unreliable due to variable R wave amplitude or artifact, pressure triggering can be used. Both balloon inflation and deflation are set relative to the upstroke of the arterial pressure waveform. Every minute pumping is suspended for one beat to permit the IABP to confirm appropriate inflation and deflation timing. Optimal timing of deflation results in a 10 to 15 mmHg reduction in aortic end-diastolic pressure (see Fig. 22-1), and manual adjustment of the deflation control may be required to achieve this.
Unlike deflation, in earlier model IABPs, balloon inflation usually has to be set manually. The inflation control is adjusted so that there is a sharp V at the dicrotic notch of the arterial pressure waveform (see Fig. 22-1). Current generation IABPs have an automatic mode in which the machine automatically sets the timing of inflation and deflation. Early IABPs were unable to augment effectively at high heart rates because of limitations on gas shuttling speed, but this is not an issue in current models.
Patient Management During IABC
IABC can result in thrombocytopenia,14 and the platelet count should be monitored daily. Arterial thrombosis can occur but this risk is minimized by ensuring that the balloon is kept cycling at all times.15 Some authors16,17 recommend anticoagulation with unfractionated heparin for patients receiving IABC; however, in a recent randomized trial, this strategy was not shown to result in reduced limb ischemia.18
Timing Errors
Early and Late Inflation
With early inflation (Fig. 22-2), the balloon inflates during late systole, before the aortic valve has closed. The arterial waveform shows augmentation occurring prior to the dicrotic notch. Early inflation results in increased left ventricular wall stress and myocardial oxygen consumption and may result in increased left ventricular end-diastolic volume and pressure. Aortic regurgitation is made worse by early inflation.
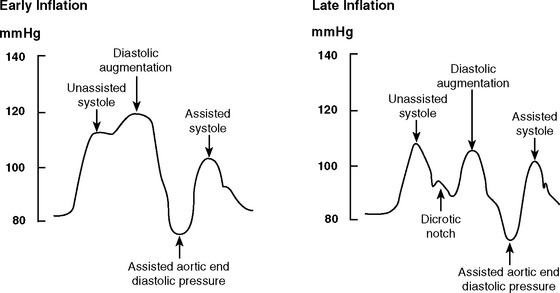
Figure 22.2 Intraaortic balloon counterpulsation showing early and late balloon inflation.
(Image courtesy of Datascope, Fairfield, NJ.)
With late inflation (see Fig. 22-2), the balloon inflates after closure of the aortic valve. The arterial waveform shows diastolic augmentation commencing after the dicrotic notch, absence of a sharp V at the dicrotic notch, and reduced diastolic augmentation. Augmentation of coronary perfusion is suboptimal.
Early and Late Deflation
With early deflation (Fig. 22-3), the arterial waveform shows a pronounced drop in pressure before late diastole. The assisted aortic end-diastolic pressure may be equal to or less than the unassisted aortic enddiastolic pressure, and the assisted systolic pressure may increase. Augmentation of coronary perfusion and afterload reduction are suboptimal. Also, there is the potential for retrograde coronary (and carotid) blood flow, which may exacerbate angina. Myocardial oxygen demand may increase.
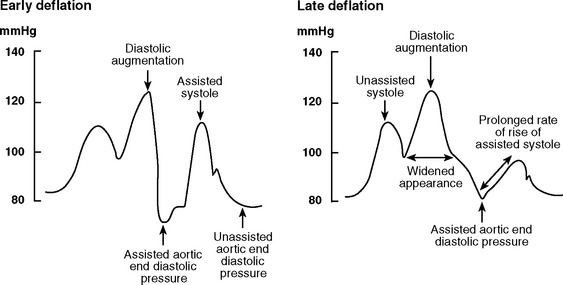
Figure 22.3 Intraaortic balloon counterpulsation showing early and late balloon deflation.
(Image courtesy of Datascope, Fairfield, NJ.)
With late deflation (see Fig. 22-3), the arterial waveform shows an assisted aortic end-diastolic pressure that is equal to or greater than the unassisted aortic end-diastolic pressure. The rate of rise of assisted systole is prolonged and diastolic augmentation may appear widened. Afterload is increased and myocardial oxygen consumption is increased.
Late deflation and early inflation are more deleterious than early deflation and late inflation. Accurate timing, and therefore effective augmentation, are difficult to achieve with tachyarrhythmias or when the dicrotic notch is poorly defined. When the dicrotic notch is difficult to discern, inflation should err on the side of lateness to minimize the risk for increasing afterload and myocardial oxygen consumption. Atrial fibrillation, particularly when rapid, can create problems with triggering and timing and can result in suboptimal augmentation.
Troubleshooting
Poor Augmentation
Failure to achieve optimal diastolic augmentation may be caused by a timing error (see previous material), by incorrect positioning of the IABP catheter, or by incomplete unfurling of the balloon. If technical problems have been excluded, the most likely reason for poor diastolic augmentation is low systemic vascular resistance. High compliance of the arterial tree—which is common in young patients—also reduces the effectiveness of IABC.19,20
Loss of Triggering
Loss of the trigger occurs when the device cannot identify the R wave on the ECG. Options include:
Balloon Leak
Balloon leak occurs in about 1% of patients.21 IABPs incorporate rapid and slow gas-leak alarms that automatically suspend cycling when a leak is detected. The presence of blood in the IABP gas line also implies balloon rupture. A complete rupture results in a gas embolism of one balloon volume (typically about 40 ml) before the device automatically ceases cycling. This generally does not cause major systemic effects and, fortunately, is extremely rare. If balloon rupture is suspected, the gas line should be clamped to prevent blood from entering the console, and the catheter should be removed immediately. The patient should be given 100% oxygen and placed in a head-down position.
Weaning
Unless complications intercede, a patient can be weaned from an IABC once inotropic drug therapy has been reduced to a low level (see Chapter 20). Ideally, the patient should have a mean arterial pressure of at least 70 mmHg, a cardiac output of at least 2.2 l/min/m2, a PAWP less than 18 mmHg, and minimal arrhythmias.15,22 Weaning from the IABP can occur before or after discontinuation of mechanical ventilation. The main advantage of weaning from the IABP first is that the patient can be placed in a sitting position prior to extubation. Augmentation frequency, rather than augmentation volume, should be weaned to ensure continued motion of the balloon and thereby minimize the risk of thrombus formation. In most circumstances, if the patient is stable after 2 to 4 hours of 1:2 augmentation, the IABP can be safely removed. However, if the hemodynamic state is marginal with 1:2 augmentation or the patient is recovering from severe ventricular dysfunction, a trial of 1:3 augmentation over several hours is appropriate before deciding whether to proceed with removal.
Outcome
IABC is employed in high-risk patient populations. Overall mortality rates in large series are about 20%,2,21 with lower mortality rates occurring in cardiac surgery patients and higher rates in patients with cardiogenic shock. Mortality rates directly related to the device itself are very low (0.05% to 0.4%).17,21,23
VENTRICULAR ASSIST DEVICES
The most appropriate candidates for insertion of mechanical ventricular assist devices (VADs) are patients facing imminent death due to acute or chronic heart failure who have preserved function of other organs.24 These patients’ illnesses fall into one of four categories: (1) cardiogenic shock following cardiac surgery; (2) cardiogenic shock following acute myocardial infarction; (3) acute heart failure, usually resulting from myocarditis but also resulting from other causes, such as cardiomyopathy and drug overdose; (4) decompensated chronic heart failure. In each of these categories a VAD may be used as a bridge to recovery, as a bridge to heart transplantation, or as permanent support (destination therapy). In the first three categories, the ideal goal is bridging to recovery. Should recovery not occur, the goal becomes bridging to transplantation. The last group of patients, those with chronic heart failure, usually can be bridged to transplantation, or they may receive a VAD as destination therapy.
The main use of VADs is as a bridge to recovery or transplantation. The use of VADs for destination therapy is, as yet, relatively uncommon. The availability of destination therapy also depends on whether a local program with funding exists. In the United States there are about 5 million people with heart failure, of whom approximately 400,000 have New York Heart Association class IV symptoms, and about 100,000 are younger than 65 years of age. There are approximately 2500 donors per year, making heart transplantation an epidemiologically trivial therapy for heart failure. As a result, there is considerable interest in VADs as destination therapy.25
Indications
Cardiogenic Shock Following Cardiac Surgery
Cardiac failure requiring VAD support occurs in less than 1% of all cardiac operations, as a result of ventricular dysfunction or intractable arrhythmias. Myocardial stunning is a major contributor to postcardiac surgery cardiogenic shock. Recovery from stunning usually occurs within 48 to 72 hours and is unlikely after 5 to 7 days.26 The likelihood of recovery is less if there was poor ventricular function before surgery, if there has been a large perioperative myocardial infarction, or if there are residual lesions (e.g., valve dysfunction, myocardial ischemia). Between 20% and 40% of patients who require a VAD following cardiac surgery survive to hospital discharge. Survival rates are much lower in patients over 65 years (17%) than in patients under 65 years (36%).27
Cardiogenic Shock Following Myocardial Infarction
Even optimally managed with IABC and revascularization, cardiogenic shock has a mortality rate of at least 40%.28 Between 50% and 60% of patients who receive a VAD in this circumstance are able to be weaned from the device, and 38% are discharged from the hospital.27 Recovery occurs within 5 to 7 days, so a short-term device is suitable for nontransplant candidates and a short- or intermediate-term device for transplant candidates.
Myocarditis and Cardiomyopathy
Fulminant, as opposed to acute, myocarditis is characterized by rapid onset and progression, fever, and severe hemodynamic compromise. If supported, patients tend to recover completely. With acute myocarditis, recovery is less likely and VAD support is more likely to be directed toward transplantation. If explantation is not possible after 3 months of VAD support, recovery is extremely unlikely, and the patient should undergo transplantation.29 When a VAD is required, the survival rate in cases of myocarditis is as high as 70%, with approximately half of survivors requiring transplantation and half recovering without transplantation.27,29,30 A short- or intermediate-term device is appropriate, with conversion to a long-term device if required. Some patients with acute cardiomyopathy, notably women with peripartum cardiomyopathy, also have a high rate of recovery with VAD support.
Decompensated Chronic Heart Failure
Decompensated chronic heart failure is the most common indication for long-term VADs. Most patients are on the heart transplant waiting list, and the aim is to bridge them to transplantation. Bridging to heart transplantation is highly effective; it carries a 60% to 90% 1-year survival rate.31 A VAD does not adversely affect survival after transplantation.32 Survival to transplantation and after transplantation may be better with a VAD than with intravenous inotropes.33 Among patients who decompensate acutely and require VAD support, there is a better survival rate if transplantation is delayed by 2 to 4 weeks to allow for end-organ recovery.34
Only one randomized trial of VAD support for nontransplant patients has been published. In the Randomized Evaluation of Mechanical Assistance for the Treatment of Congestive Heart Failure (REMATCH) trial, patients were randomized to a HeartMate (Thoratec, Pleasanton, CA) or to maximal medical therapy. The 1- and 2-year survival rates were 52% and 23%, respectively, for the VAD group and 25% and 8% for the medical therapy group.35 However, the VAD patients had 2.5 times more adverse events, the predominant ones being infection, bleeding, and device malfunction. Infection accounted for 41% of the deaths of the patients who had VADs. At 2 years, the probability of device malfunction was 35%. The incidence of bleeding complications and stroke were 42% and 16%, respectively. Thus, although the treatment effect was three times better than that of medical therapy, there were considerable quality-of-life limitations. Currently, the RELIANT trial is recruiting patients to compare the improved Novacor (Worldheart, Oakland, CA) and the HeartMate XVE (Thoratec, Pleasanton, CA) devices in nontransplant patients.
Between 5% and 15% of patients bridged to heart transplantation experience sustained improvement in native heart function such that the VAD can be explanted. Most of these patients have acute heart failure, but recovery is described in chronic heart failure too. Recovery is more likely when the cause is nonischemic and when there is minimal myocardial fibrosis.36,37 Recovery after more than 2 to 3 months of VAD support is exceedingly unlikely.29,37
Patient Evaluation
General Hemodynamic State
If a patient meets the criteria for a VAD, there are two critical questions to be asked: (1) Is there a reasonable chance of recovery? and (2) Is the patient a candidate for heart transplantation? If the answer to both of these questions is no, in the absence of a destination VAD program, mechanical support should not be offered. However, if the patient is a candidate for transplantation, a short-term device might be used initially if there is a good chance for recovery. Otherwise, a medium- or long-term device should be chosen. When there is clinical uncertainty about the potential for recovery or transplant status, a short-term device to bridge to a long-term device (a “bridge-to-bridge” device) may be used; it does not reduce the chances for survival.39 An example would be where there is uncertainty about whether brain damage has occurred following a cardiac arrest and time is required for neurologic assessment. If a destination VAD program is available, this is an option for non-transplant candidates. Assessment also involves review of other cardiac and noncardiac considerations.
Right Ventricular Function
Between 20% and 30% of patients with severe left ventricular failure also have significant right heart dysfunction, but less than 10% of patients receiving an LVAD require an RVAD. Predictors of the need for an RVAD include a CVP more than 16 to 20 mmHg, female gender, nonischemic cause, and preoperative circulatory support.40 High right ventricular volumes and poor contractility (suggested by low pulmonary artery pressures) are also important predictors.40,41 Pulmonary hypertension has been shown by some, but not others, to be a risk factor for requirement of an RVAD.40,41 Most commonly, the need for an RVAD is established during implantation of the LVAD because of low pump flows despite optimal inotropic support. Survival rates are lower when an RVAD is deployed later, so it should be used early if it is indicated.42 The need for an RVAD increases the incidence of bleeding and is associated with a lower survival rate than the use of an LVAD alone.40,43
Intracardiac Shunts
If a patient has a patent foramen ovale or an atrial septal defect, it should be closed during the implant procedure because it may cause significant right-to-left shunting and hypoxemia. This may not be apparent until after the LVAD has commenced and left atrial pressure drops.44 Transesophageal echocardiographic (TEE) assessment specifically looking for atrial shunting should be routinely performed before and after LVAD implantation.
Noncardiac Considerations
In most programs, age limits are the same as those used for heart transplantation (60 to 65 years).25 Age above 65 years is an independent predictor of poor outcome.45 Neurologic function should be fully assessed, especially if there has been a preoperative cardiac arrest or prolonged hypotension. Significant liver dysfunction is a very poor prognostic sign and may contraindicate a VAD. A prothrombin ratio above 1.5 or transaminases or bilirubin more than five times normal were exclusion criteria in the REMATCH trial.35 Severe renal dysfunction is not a contraindication to VAD implantation.46 In the absence of multiple organ dysfunction syndrome (see Chapters 2 and Chapter 20), renal function usually recovers following VAD implantation. Temporary renal replacement therapy is commonly required prior to and following VAD implantation.
Device Selection
Pulsatile Versus Nonpulsatile Pumps
Pulsatile devices (Figs. 22-4 and 22-5) are volumedisplacement pumps. They have a pumping chamber in which blood collects and is then intermittently rapidly compressed, either pneumatically or by an electric motor. Unidirectional blood flow is achieved with valves. They eject a “stroke volume” that is determined by the chamber volume and the outflow resistance. Nonpulsatile (continuous-flow) devices generate flow using either an axial or a centrifugal flow pump (Figs. 22-6 and 22-7). Axial flow pumps are smaller than centrifugal pumps and operate at higher rotational speeds to achieve the same flow and pressure.47
Pulsatile pumps have the longest track record for intermediate- and long-term support. All of the VADs currently approved by the U.S. Federal Drug Administration (FDA) are pulsatile devices. The advantages of pulsatile perfusion are debated. Nonpulsatile flow has been described as causing widespread microcirculatory and functional changes, including changes in the heart, brain, lungs, kidneys, and vascular system. Many of the studies showing these changes are based on short-term studies and are not applicable to long-term support. Animal studies demonstrate that is it possible to provide long-term nonpulsatile flow with no detectable change in end-organ function.48,49
Extracorporeal Pulsatile Devices
Abiomed BVS 5000.
The Abiomed BVS 5000 (Abiomed, Danvers, MA) is a two-chamber, pneumatically driven device. It is the most widely used device for short-term support following cardiac surgery and is licensed by the FDA for this purpose. Its advantages are that it can be used for either univentricular or biventricular support, it is easy to implant and maintain, and it is widely available.50 Its disadvantages are that thromboembolic, bleeding, and infectious complications (often leading to multiple organ dysfunction syndrome) occur with prolonged use. Systemic anticoagulation is required, and support is limited to about 2 weeks.
Thoratec.
Thoratec (Thoratec, Pleasanton, CA) is a pneumatically powered system that has a seamless polyurethane blood sac inside a rigid polycarbonate housing (see Fig 22-4). It has mechanical valves, is capable of flows of 1.3 to 7.2 l per minute, and has been implanted in patients who weigh between 17 and 144 kg. It can provide left, right, or biventricular support and is used for short- to medium-term support.51 Its external position makes it less suitable for long-term support but it allows easier pump exchange in the event of malfunction, thrombus, or infection. Systemic anticoagulation is required. It is FDA-approved for postcardiotomy support and as a bridge to transplantation.
Intracorporeal Pulsatile Devices
HeartMate.
There are two types of HeartMate (Thoratec, Pleasanton, CA) devices. The HeartMate IP (Implantable Pneumatic) is powered by an external pneumatic unit; the HeartMate XVE (Vented Electric; see Fig. 22-5) contains an electric motor within the blood pump’s housing. The external unit is therefore smaller so that the XVE allows for greater patient mobility. The XVE replaced the earlier VE (used in the REMATCH study discussed earlier) with modifications including alterations to the power lead and to the inflow valve conduit. They are both FDA-licensed for bridge to transplantation, and the XVE is the only device licensed for destination therapy. A unique feature of both devices is that the blood-contacting surfaces are textured and become covered by a “pseudoneointimal” layer. As a result, patients do not necessarily require anticoagulation other than with aspirin.
Novacor.
The Novacor (Worldheart, Oakland, CA) uses electrically driven dual pusher plates that compress a seamless polyurethane blood sac. It requires systemic anticoagulation. This device initially had a high risk of thromboembolic complications,52 but these have been reduced by modifications of the cannula design.53
Nonpulsatile Axial Pumps
Axial flow pumps commonly operate at around 8000 to 12,000 rpm and have flow rates of 5 to 7 l/min. Some are capable of flow rates above 10 l/min. They are typically the size of a D-cell battery, and all are intracorporeal. One concern with axial pumps is that because they do not have valves, if they fail, blood can pass from the aorta back through the device and into the left heart. However, experience with the Jarvik 2000 (Jarvik Heart, New York, NY) axial device has shown that when the pump is deliberately stopped, the regurgitant flow is on average 0.35 l/min/m2 and that this is well tolerated by the majority of patients.54
Jarvik 2000.
The Jarvik 200055,56 (Jarvik Heart, New York, NY; see Fig. 22-6) pump is placed in the left ventricle and can deliver flows of 2 to 7 l/min. Clinical experience suggests that the device remains well aligned during changes in left ventricular shape during unloading, thus removing problems of inflow cannula kinking and distortion. The outflow conduit is anastomosed to the descending aorta. The power cable can be bought out through the abdominal wall or, for long-term support, can be tunneled up to exit behind the patient’s ear, where power is delivered through a rigidly fixed percutaneous titanium pedestal.
MicroMed DeBakey.
The MicroMed DeBakey57 (MicroMed Cardiovascular, Houston, TX) is a titanium pump with inflow and outflow cannulas. It is implanted below the diaphragm under the left costal margin. It is connected to the left ventricular apex and the ascending aorta.
Berlin Heart Incor.
The Berlin Heart Incor58 (Berlin Heart, Berlin, Germany) is unique among axial flow pumps in having a magnetically suspended impeller. There is therefore no mechanical friction or heat generation and less risk of thrombus formation at a bearing site. At 12 cm, it is longer than other axial pumps.
Centrifugal Pumps
Conventional centrifugal pumps, such as the Biomedicus (Medtronic, Eden Prairie, ME), the Jostra Rotaflow (Maquet Cardiopulmonary, Rastatt, Germany), and the Levitronix (Levitronix, Waltham, MA), provide excellent short-term support via a CPB circuit extracorporeal membrane oxygenation (ECMO), or as a short-term VAD. They require direct cardiac cannulation and are therefore particularly with an extracorporeal membrane oxygenation circuit suited to support after cardiac surgery. In addition, a percutaneous centrifugal pump (Tandem Heart Percutaneous Transseptal Ventricular Assist (PTVA) System [Cardiac Assist, Pittsburgh, PA]) has been utilized. This uses groin cannulation: left atrial cannulation is made via the inferior vena cava and across the interatrial septum, and return is made via the femoral artery.59 These centrifugal pumps are prone to causing thrombus formation and have limited durability and significant heat generation by their bearings. This limits their use to around 10 to 14 days, and pump head changes may be required during this time.
A new generation of bearingless centrifugal pumps has been developed and is undergoing phase-two clinical trials. They all have magnetically or hydrodynamically suspended rotors or both. Like the Berlin Heart Incor, they have only one moving part, are frictionless (so parts do not wear out), and cause less heat generation and potentially less thrombus formation. They include the HeartMate III (Thoratec, Pleasanton, CA); the VentrAssist (Ventracor, Queensland, Australia [see Fig. 22-7]); the CorAide (Arrow International, Tucson, AZ); the HeartQuest (World Heart, Oakland, CA); and the Terumo (Terumo, Tokyo, Japan).
Total Artificial Hearts
Two total artificial hearts, the CardioWest (Syncardia Systems, Tucson, AZ, Figure. 22-8) and the AbioCor (Abiomed, Danvers, MA), are undergoing clinical trials. These are pulsatile devices with four valves that replace the native heart. The CardioWest is designed as a temporary device, and it has been used in a study of patients with biventricular failure as a bridge to transplantation. Survival to transplantation occurred in 79% of those bridged with the device compared with a historical control rate of 46% in patients who had the same entry criteria.60 The AbioCor is undergoing clinical trials for destination therapy.
ICU Management
Preoperative management of cardiogenic shock and end-stage heart failure is discussed in Chapters 19 and Chapter 20. An echocardiogram should be performed preoperatively to confirm the presence of severely impaired ventricular function and also to examine for aortic incompetence, mitral valve disease, intraventricular thrombus, and either a patent foramen ovale or an atrial septal defect (Table 22-3). Postoperative management is determined by the patient’s preoperative state and the intraoperative course. When VAD insertion has been performed as an elective procedure and the intraoperative course has been uncomplicated, the patient may be awakened rapidly and extubated within a few hours of surgery. However, a patient who is acutely ill prior to surgery or who has had a long CPB time may be very unstable in the early postoperative period and may require prolonged ICU care. In a patient with marginal right ventricular function and in one who has developed a marked systemic inflammatory response to CPB, LVAD support may be inadequate to maintain an adequate hemodynamic state. High doses of inotropic and vasopressor drugs, with or without an RVAD, may be required.
Table 22-3 Conditions That Should Be Assessed by Transesophageal Echocardiography in Patients With Ventricular Assist Devices
Before Implantation | During Implantation | After Implantation |
---|---|---|
Patent foramen ovale and atrial septal defect | Deairing of heart | Hypovolemia |
Aortic regurgitation | Right ventricular function | Right ventricular function |
Mitral stenosis | Inflow cannula position | Inflow cannula obstruction |
Right ventricular function | Patent foramen ovale and atrial septal defect | Cardiac tamponade |
Intracavity thrombosis | Outflow cannula obstruction | |
Aortic atheroma | Intracavity or conduit thrombus | |
Inflow valve regurgitation (late) |
Adapted from Scalia GM, McCarthy PM, Savage RM, et al: Clinical utility of echocardiography in the management of implantable ventricular assist devices. J Am Soc Echocardiog 13:754-763, 2000.
Complications
Bleeding
Problematic bleeding is common following VAD implantation.61 Treatment involves aggressive use of blood, blood component therapy, antifibrinoltyics (see Chapter 30), and early reoperation.
Low Pump Flows
Right ventricular dysfunction is the most common cause of low LVAD pump flows. Treatment is twofold. First, right ventricular function should be supported with inotropes, vasopressors, and judicious fluid loading (see Chapter 20). Second, pulmonary vascular resistance should be kept as low as possible by avoiding hypoxemia and acidemia, avoiding high airway pressures and positive end-expiratory pressure (PEEP), and using pulmonary vasodilators, such as inhaled nitric oxide or aerosolized prostacyclin (see Chapter 24). In some patients it is possible to achieve adequate LVAD flows even with absent right ventricular function (e.g., with intractable ventricular fibrillation), although RVAD support is usually required. The choice of RVAD depends on the type of LVAD used because many devices can be used in both positions.
Infection
In the REMATCH trial, device-related infection was 28% by 3 months, and sepsis was the most common cause of death. The extensive foreign material and relative immunosuppression that occurs with chronic illness make successful treatment difficult if not impossible, if the device is involved with infection. Prophylactic antibiotics—including antifungal agents—should be given preoperatively and continued for 48 hours after surgery. Routine culturing of blood, urine, tracheal secretions, and wound sites is indicated, and antimicrobial treatment should be started at a threshold lower than that provided for other patients in the ICU. Invasive catheters and cannulas should be inspected regularly, replaced at the first sign of infection, and removed at the earliest opportunity. Preoperative risk factors for infection include preexisting infection, malnutrition, immunosuppressive drug therapy, mechanical ventilation, and intravascular catheters. Staphylococcus species, Pseudomonas aeruginosa, Enterococcus species, and Candida species are the usual causative agents.62,63
Device-related infections are of three types: (1) drive-line infections, (2) pump-pocket infections, and (3) infections on blood-contacting surfaces. Drive-line infections are suggested by poor healing and inflammation of the skin sites. They should be managed by careful cleaning of the exit site and by topical and systemic antimicrobials. If the infections are refractory to these treatments, aggressive surgical debridement is indicated.64 Pump-pocket infections may be indolent and show few symptoms or may cause systemic sepsis. They may also cause purulent discharge from the drive line. If there is persistent bacteremia or signs of persistent local infection despite systemic antibiotics, surgical exploration may be required. Some authors advocate leaving antibiotic-impregnated beads in the pocket at the time of drainage.63 Infective endocarditis stemming from the pump can be difficult to distinguish from blood stream infections derived from other sites. The diagnosis is suggested by: (1) septic emboli in the absence of vegetations on native valves; (2) new incompetence of the device inflow or outflow valves; (3) persistently positive blood cultures despite appropriate antimicrobials; (4) no other source of infection.63 Infection can be suppressed by systemic antimicrobials or treated by transplantation. If urgent transplantation is not possible, replacement of the device may be required.
Neurologic Injury
Neurologic problems arising within the first few days following surgery are relatively uncommon and are usually caused by perioperative hypotension. Late (over weeks to months) neurologic problems are usually due to thromboembolism caused by the VAD and are very common. In the REMATCH trial, 44% of patients experienced at least one neurologic event and 16% experienced a stroke.65 VAD thromboembolic disease is commonly associated with infection in the device and is very difficult to treat. The best option is to remove the VAD and have the patient undergo urgent heart transplantation.
Device Malfunction
In the REMATCH trial, the incidence of major device malfunction was 35% by 2 years, and this was the second most common cause of death. In a more recent series, device malfunction occurred in 12.8% of patients.31
With pulsatile devices, the inflow valve is under high mechanical stress because it opposes high pressures from a noncompliant pump. Inflow valve regurgitation is suggested by rapid VAD cycling when the device is in automatic mode. This occurs due to rapid filling of the VAD by the distended left ventricle. The diagnosis is usually confirmed by demonstrating regurgitant flow through the inflow cannula by Doppler flow imaging using TEE. Doppler flow imaging through the outflow cannulas shows reduced blood velocity. In addition, the left ventricle appears dilatated and the aortic valve, which normally opens infrequently, may open more frequently.66 The average time to onset of inflow valve regurgitation is 6 months (range 2 to 15 months).67 Outflow valve regurgitation is rare.
Obstruction to either conduit can also occur. The diagnosis is suggested by velocities within these conduits greater than 2.5 m/sec as indicated by continuous-wave Doppler.68 The position of the inflow cannula should be assessed at the time of VAD implantation and subsequently in the ICU so as to ensure that it is central in the apical ventricular cavity. In practice, it is often angulated anteroseptally and, although this does not usually cause problems, it can become obstructed by the ventricular wall. This may be suggested by high velocity (aliased) flow at the cannula orifice as shown by pulsed-wave or color-flow Doppler.69 Thrombus within the cavities of the heart or within the conduits may also be assessed by TEE.
EXTRACORPOREAL MEMBRANE OXYGENATION
For more detailed information on all aspects of ECMO, the reader is referred to ECMO: Extracorporeal Cardiopulmonary Support in Critical Care, produced by the Extracorporeal Life Support Organization (ELSO).70
Background
The first successful use of ECMO was reported in 1972. Since then there have been a number of randomized and nonrandomized trials comparing ECMO with standard medical therapy. Most of these trials have been in neonatal respiratory failure, and all of them have shown a survival benefit. There have been two randomized trials in adults with ARDS and neither has shown a survival benefit.71,72 Both used ECMO techniques that are now outdated and had complication rates considerably higher than those reported recently by high-volume ECMO centers. Currently, a well-designed randomized trial of ECMO in adults with acute respiratory failure (the CESAR trial: www.cesar-trial.org) is being conducted in the United Kingdom.
Despite the lack of supporting trial data, a number of experienced ECMO centers have reported survival rates of 55% to 76% in adults with severe ARDS and predicted mortality rates of more than 70% to 80%.73–76 ECMO can fully support and save the lives of some patients with severe respiratory or cardiac failure, but the best results are obtained in centers with established programs and well-trained and experienced staff who use standardized protocols and equipment.
Indications and Contraindications
Cardiac Failure
The indication for ECMO for cardiac support is acute heart failure causing cardiogenic shock (cardiac output <2 l/min/m2, systolic blood pressure <90 mmHg, lactic acidosis) despite maximal medical treatment, invasive ventilation, and IABC. In this setting, ECMO may be used as a bridge to recovery, to a VAD (“bridge-to-bridge”), or to heart transplantation. For left ventricular failure, an LVAD (or BiVAD) is preferable to ECMO because an oxygenator adds considerable inflammatory activation and complexity to the circuit. However, virtually all VADs (apart from the Tandem Heart PTVA system) require sternotomy and usually CPB for insertion, whereas ECMO may be commenced with percutaneous cannulation. ECMO may therefore be preferable to a VAD in certain situations, including (1) when there is also significant acute lung injury or right ventricular failure; (2) when the patient has not had a recent sternotomy and urgent or short-term cannulation only is required; (3) when there is significantly more institutional experience with ECMO than with VADs. Similar results have been achieved with ECMO and with VADs in this situation.77,78
Contraindications
Absolute contraindications include: (1) contraindications to systemic anticoagulation; (2) underlying moderate to severe chronic lung disease; (3) significant neurologic injury; (4) severe immunosuppression; (5) advanced multiple organ failure; (6) terminal underlying disease. Relative contraindications are being older than 60 to 70 years of age and having been mechanically ventilated for longer than 10 days.79,80 Death rate on ECMO is directly related to the duration of mechanical ventilation prior to its institution and rises significantly when that time exceeds 5 days.79
ECMO Circuits
The basic components of an ECMO circuit are: (1) drainage (venous) and return (arterial) cannulas; (2) a blood pump; (3) an oxygenator; (4) a heat exchanger; (5) connecting tubing (Fig. 22-9). Additional components may also be included (see Circuit Components in the subsequent material). There are also devices to maintain the safety of and measure the functioning of the circuit. Circuit components should be chosen to enable a blood flow of at least 50 to 60 ml/kg/min. As much as 70 to 100 ml/kg/min may sometimes be required in septic patients. The Paco2 is determined by the oxygenator gas flow (or sweep speed). In practice, CO2 is efficiently cleared by ECMO and usually Paco2 is easily controlled. The determinants of Pao2 are more complex, but the most important determinant is the circuit blood flow.
Modes of Support: Venoarterial and Venovenous ECMO
Venoarterial.
Despite the advantage of providing complete cardiorespiratory support, there are a number of disadvantages of VA ECMO. First, a large artery must be cannulated. Arterial cannulation usually requires a surgical cutdown, which may cause persistent bleeding at the insertion site. It also poses a small risk of arterial gas embolism, and it may compromise distal limb perfusion. Second, cardiac function may be compromised due to the high afterload imposed by the retrograde flow from the cannula toward the heart. Third, pulmonary perfusion is greatly reduced, which may adversely affect recovery from acute lung injury. Fourth, if the left ventricle is completely nonfunctioning, it may become grossly distended, resulting in cardiac damage and pulmonary edema. Fifth, if VA ECMO is utilized (inappropriately) for respiratory failure in a patient with good cardiac function, the coronary arteries and cerebral vessels may be perfused by deoxygenated blood. These last two problems are discussed in Complications subsequently. Because of these difficulties, VA ECMO should be reserved for patients with profound cardiac failure.
Circuit Components
Cannulas.
The size of the venous cannula determines blood flow. The largest possible venous cannula should therefore be placed. A 23F cannula is adequate for most patients (23F through 29F have been used). For the return cannula, a 19F through a 21F is used for arterial cannulation and a 21F through a 23F for venous cannulation. With VV ECMO, placement of the drainage cannula in the femoral vein and the return cannula in the jugular vein provide higher flow rates and less recirculation than the reverse situation.81 With this arrangement, the tip of the drainage cannula should sit below the level of the diaphragm and the tip of the return cannula in the proximal superior vena cava above the level of the right atrium. The position of both cannulas can usually be confirmed by TEE and a chest radiograph. TEE will also show the pattern of flow out of the cannula, which may be helpful if recirculation is suspected. For VA ECMO, the venous cannula is best placed in the internal jugular vein (with the tip positioned in the proximal superior vena cava). Venous cannulas can be placed percutaneously in the majority of cases.82 Arterial cannulas for VA ECMO may be placed in the femoral, axillary, or carotid arteries. Although femoral arterial cannulas can be placed percutaneously, a distal leg perfusion cannula (10F to 12F) is commonly required, and that requires surgical placement.83 This distal cannula is placed routinely in our practice.
Blood Pump.
The blood pump may be either a roller pump or a centrifugal pump. There is debate as to the relative merits of the two, but in practice both are satisfactory and the choice will be determined by institutional experience. A roller pump requires a bladder or reservoir between the venous cannula and the pump and utilizes gravity for drainage into the reservoir. Centrifugal pumps do not require gravity, so they can be placed at any height in relation to the patient. Centrifugal pumps can generate very high negative pressures, which may cause hemolysis. However, if the pressure is measured continuously at the circuit end of the venous cannula, excessively high negative pressures can be avoided. Older style centrifugal pumps are also prone to thrombus formation in the pump head. This problem is reduced with newer generation pumps (e.g., Jostra RotaFlow, Maquet Cardiopulmonary, Rastatt, Germany; Fig. 22-10).
Oxygenators.
Oxygenators provide gas exchange and may be composed of silicone membrane or hollow fiber. Silicone membrane oxygenators (Fig. 22-11) have been the standard for many years. They have excellent biocompatibility and good gas-exchange characteristics, and they are relatively nonthrombogenic. However, they have a high resistance to blood flow, are difficult and time-consuming to prime, and cannot be coated with heparin. They are less efficient than hollow-fiber devices, and sometimes two oxygenators, placed in parallel, are required. In comparison, hollow-fiber oxygenators have more efficient gas exchange, are quick and easy to prime, and can be coated with heparin. The original polypropylene hollow-fiber oxygenators (e.g., Maxima [Medtronic Bio Medicus, Eden Prairie, ME]) develop a plasma leak through the membrane, usually after 1 to 5 days, which requires replacement of the oxygenator and makes them unsuitable for anything other than emergency or short-term ECMO. There is now a new generation of polymethylpentene hollow-fiber oxygenators, such as Jostra Quadrox (Maquet Cardiopulmonary, Rastatt, Germany [Fig. 22-12]) and Medos Hilite 7000LT (Medizintechnik, Stolberg, Germany), which do not develop a plasma leak, have lower platelet consumption, and require less blood product support.84 Currently, these polymethylpentene hollow-fiber oxygenators are not licensed by the FDA.
Bridge.
The location of the bridge within the circuit is shown in Figure 22-9A. It is normally clamped but can be opened in an emergency, for instance, when there is air within the circuit (see Air in the Circuit in subsequent material). With the bridge open, blood can continue to circulate within the circuit (and therefore de-airing can potentially be achieved), while the drainage and return cannulas are clamped. However, many centers do not use bridges. An alternative is a “stopcock” bridge using stopcocks instead of Y connectors. The bridge is flushed with heparinized clear fluid and remains closed. This removes the risks associated with intermittent flushing of the bridge by circuit blood (i.e., clot embolization, sudden changes in blood pressure and cerebral blood flow) but provides the safety benefits.
Management of ECMO
Commencing ECMO
Following cannulation, ECMO is commenced by gradually increasing blood flow until venous return is limited by venous drainage. For most adults, a flow of 5 l/min should be possible. If the circuit blood flow that can be achieved is significantly less than this and the patient’s blood volume is adequate, there is likely to be a problem with the position of the drainage cannula. Once adequate flow has been achieved, mechanical ventilation should then be lowered to “rest” settings (typically FIo2 0.3 to 0.5, peak inspiratory pressure 20 to 25 cm H2O, PEEP 10 to 15 cm H2O, breath rate 5/min). Circuit blood flow commonly improves once mean airway pressure is reduced. It is important to stress that the goal of VV ECMO is not to achieve normal oxygenation, but to achieve the same levels as would be found in a mechanically ventilated patient with severe ARDS (i.e., Sao2 85% to 92%) and to rest the lungs. Once rest ventilator settings have been achieved, circuit blood flow should be adjusted to achieve an Sao2 of 85% to 92%. The circuit venous saturation will usually be approximately 80% to 85%. The oxygenator fresh gas flow should be altered to achieve a Paco2 of 40 to 50 mmHg (5.3 to 6.7 kPa). Paco2 is almost always easily maintained at the desired level. Early in the course of the ECMO run, flow should be increased to establish the flow limitation of venous drainage. With a roller pump, this is the flow at which the bladder collapses; for a centrifugal pump, it is the flow at which inlet pressure rapidly becomes more negative and flow starts to fall. Usually this flow rate is significantly greater than that required to support the patient. However, if despite good positioning of cannulas and intravenous volume loading, maximal flow is inadequate or if mechanical ventilation cannot be reduced to rest settings, a second drainage cannula is required. With VA ECMO, blood flow is adjusted according to blood pressure and signs of adequate systemic blood flow. This includes a falling lactate level and a circuit venous saturation of approximately 75%, which will usually produce an Sao2 greater than 90%.
Laboratory Testing and Anticoagulation
Blood gases should be measured every 4 to 8 hours, and anticoagulation should be monitored hourly by examining activated clotting times (ACTs). This should be supplemented with standard coagulation tests and a complete blood count 2 to 4 times daily. Some centers also measure antithrombin III levels daily and perform regular thromboelastography. Anticoagulation is achieved by means of a heparin infusion adjusted to maintain an ACT of 160 to 180 seconds (Hemotec; the goal ACT will be different when other methods of measuring ACT are used). Platelets should be transfused to maintain the count above 100,000/mm3. Hematocrit should be maintained above a minimum of 30%, and some centers maintain it above 40% to 45%.73 Plasma-free hemoglobin should be measured twice daily to monitor for hemolysis related to either circuit thrombus or excessive negative pressure. Daily circuit blood cultures should be obtained.
Troubleshooting and Complications
A range of complications and problems can occur during ECMO. Some of them are common to all forms of ECMO and some are specific to VV or VA ECMO or to certain types of circuit components (e.g., centrifugal pumps). The symptoms that may occur (e.g., low venous saturation, increasingly negative inlet pressure) and their potential causes are listed in Table 22-4. Specific problems relating to the circuit and the patient are described next.
Table 22-4 Troubleshooting ECMO: Common Symptoms and Their Potential Causes and Solutions
Symptom | Possible Causes | Interventions |
---|---|---|
Low arterial oxygen saturation | Oxygenator failure, disconnected gas supply, ↑ patient VO2 (e.g., sepsis), ↓patient lung function (e.g., nosocomial pneumonia) | Check gas connections, pre- and postoxygenator pressures, and blood gases; sedate and paralyze; perform CXR, bronchoscopy, and BAL. |
Low circuit venous saturation | Inadequate circuit flow | Increase circuit flow. |
High (negative) inlet pressure | Hypovolemia (e.g., inadequate fluids, bleeding), displaced cannula, tension pneumothorax or hemothorax, cardiac tamponade | Check volume load, perform CXR, chest/abdomen ultrasound, echocardiogram. |
Hypotension | Hypovolemia (e.g., inadequate fluids, bleeding), displaced cannula, tension pneumothorax or hemothorax, cardiac tamponade, myocardial dysfunction (e.g., ischemia, arrhythmias), sepsis | Check volume load, perform CXR, echocardiogram, chest/abdomen ultrasound, hemoglobin, electrolytes, blood cultures, bronchoscopy, and BAL. |
Bleeding | Surgical site bleeding, coagulopathy | Perform surgical exploration; diathermy all bleeding sites; keep platelets >150,000/mm3, fibrinogen >200 mg/dl, PR <1.5; check aprotinin; lower ACT target. |
Air in circuit | Circuit opening before pump (e.g., side hole of venous cannula out of vessel, connection/three-way tap open), highly negative prepump pressure | Large air volume: clamp arterial and venous cannulas, open bridge (if present), stabilize patient, remove air or replace circuit. |
Small air volume: shift air to reservoir, oxygenator, or heat exchanger, and aspirate. | ||
High plasma hemoglobin | Clots in circuit, high inlet pressures (centrifugal pump) | Check circuit, change component or whole circuit. |
Low ACT despite “adequate heparinization” | Low antithrombin III | Administer recombinant antithrombin III, plasma. |
ACT, activated clotting time; BAL, bronchoalveolar lavage; CXR, chest radiograph; ECMO, extracorporeal membrane oxygenation; PR, prothrombin ratio; VO2, oxygen consumption.
Circuit Problems
Air Embolism.
If a small volume of air enters the circuit it may be able to be moved to the venous reservoir, the oxygenator, or the heat exchanger and then aspirated. If a large volume of air enters the circuit, an air embolism or venous airlock develops. In this circumstance, the drainage and return cannulas should be clamped immediately and primary attention directed to maintaining the patient’s ventilation and circulation. If a bridge is present, it should be opened and the circuit kept running while attempts are made to remove the air.
Loss of Circuit Flow.
Loss of circuit flow is usually caused by loss of venous return as a result of cannula malposition or hypovolemia. With centrifugal pumps, an increasingly negative inlet pressure usually indicates hypovolemia. With a roller pump, hypovolemia results in the slowing or stopping of the pump due to collapse of the bladder. Initially, loss of circuit flow should be managed by giving intravenous fluid. Circuit flow may have to be transiently decreased to prevent or release suction by the vessel on the cannula. The circuit should also be checked for any obstruction to venous flow, such as a kinked cannula or a misplaced clamp. Less common, patient-related causes of loss of flow include pericardial fluid, pneumothorax, and hemothorax. If flow is not easily restored by intravenous volume expansion, a chest radiograph and an echocardiogram should be performed.
LV Distension.
LV distension can occur in a patient on VA EMCO who has a completely nonfunctioning left ventricle. Any blood that passes through the lungs and returns to the left heart is not ejected into the aorta and can cause severe left ventricular distension and florid pulmonary edema. Permanent ventricular damage can occur. Increasing the pump flows to reduce blood flow through the lungs may ameliorate the situation. Failing this, urgent venting of the left heart, either by direct cannulation of the left atrium (via a thoracotomy) or by percutaneous atrial septostomy (in the catheterization laboratory), must be performed.85
Patient Problems
Bleeding.
Minor bleeding should be managed by ensuring that the platelet count is over 100,000/mm3 and that coagulation is normal apart from the effects of heparin. Bleeding at percutaneous cannulation sites is uncommon, requiring intervention in only 6% of cases.82 Usually hemostasis can be achieved by a purse-string suture around the site. Surgical-site, tracheotomy, and gastrointestinal bleeding also occur. When major bleeding occurs, coagulation should be managed by increasing the platelet count to more than 150,000/mm3 and ensuring that the fibrinogen concentration is more than 200 mg/l and that the prothrombin ratio is less than 1.5. If fibrinolysis is identified by TEG, an antifibrinolytic should be given (see Chapter 30). If bleeding continues, the target ACT range should be lowered (e.g., to 140 to 160 seconds).
Minor procedures such as tube thoracostomy can cause significant bleeding, and the need for all such procedures should be carefully reviewed. For example, a nontension pneumothorax may not need to be drained. If a chest drain is required, small drains placed by means of a Seldinger technique should be used for draining air and serous fluid (see Chapter 40). Larger chest drains, necessary to drain blood or purulent fluid, should be placed surgically using diathermy.
The most serious bleeding complication is intracranial hemorrhage. Risk factors for this include a platelet count less than 50,000/mm3, female gender, and renal failure. It is usually fatal.86
Cardiorespiratory Deterioration.
Acute hypoxemia in a previously stable patient should be assessed first by reviewing the ECMO circuit flow. If it has fallen, management is the same as for loss of pump flow. If it is unchanged, the oxygenator may be failing or the oxygenator fresh gas flow may have become disconnected. After checking gas connections and the oxygenator for leaks before and after the oxygenator, blood gases should be checked. Slower deterioration in oxygenation occurs when the patient’s oxygen consumption is increasing (e.g., sepsis, increased patient activity) or when the native lung function is getting worse. There should be a low threshold for performing a bronchoscopy and bronchoalveolar lavage to assist in the diagnosis of this problem (see Chapter 35).
Sepsis and Infection.
Bloodstream and urinary tract infections are more common in patients undergoing ECMO than in patients in the ICU in general, and the rate increases with the duration of ECMO.87 Lower respiratory tract infections occur with a frequency similar to that in other mechanically ventilated patients. The organisms cultured are the same as those found in other critically ill patients. Circuit blood cultures should be performed daily in all patients. If sepsis is suspected, empiric antimicrobial therapy should be administered (see Chapter 35).
Weaning From ECMO
Signs of Reversibility
If recovery is going to occur, cardiac function recovers faster than lung function. If cardiac function has not recovered by 5 to 7 days, recovery is unlikely. Respiratory function usually recovers by 1 to 3 weeks but occasionally takes longer. A patient with respiratory failure who is not improving should receive aggressive diuresis. Progressive pulmonary arterial hypertension may indicate irreversible lung disease.79 Right ventricular failure and a mean pulmonary artery pressure more than two thirds that of the mean arterial pressure after days or weeks of VV ECMO support is almost always a sign of irreversibility.88 Equally, if the mean pulmonary artery pressure is less than half that of the systemic arterial pressure with no signs of lung improvement, recovery is still common.88
1 Scholz KH, Ragab S, von zur Muhlen F, et al. Complications of intra-aortic balloon counterpulsation. The role of catheter size and duration of support in a multivariate analysis of risk. Eur Heart J. 1998;19:458-465.
2 Stone GW, Ohman EM, Miller MF, et al. Contemporary utilization and outcomes of intra-aortic balloon counterpulsation in acute myocardial infarction: the benchmark registry. J Am Coll Cardiol. 2003;41:1940-1945.
3 Creswell LL, Rosenbloom M, Cox JL, et al. Intraaortic balloon counterpulsation: patterns of usage and outcome in cardiac surgery patients. Ann Thorac Surg. 1992;54:11-20.
4 Christenson JT, Simonet F, Badel P, et al. Evaluation of preoperative intra-aortic balloon pump support in high risk coronary patients. Eur J Cardiothorac Surg. 1997;11:1097-1103.
5 Christenson JT, Simonet F, Badel P, et al. The effect of preoperative intra-aortic balloon pump support in patients with coronary artery disease, poor left-ventricular function (LVEF 40%), and hypertensive LV hypertrophy. Thorac Cardiovasc Surg. 1997;45:60-64.
6 Christenson JT, Simonet F, Badel P, et al. Optimal timing of preoperative intraaortic balloon pump support in high-risk coronary patients. Ann Thorac Surg. 1999;68:934-939.
7 Holman WL, Li Q, Kiefe CI, et al. Prophylactic value of preincision intra-aortic balloon pump: analysis of a statewide experience. J Thorac Cardiovasc Surg. 2000;120:1112-1119.
8 Baskett RJ, Ghali WA, Maitland A, et al. The intraaortic balloon pump in cardiac surgery. Ann Thorac Surg. 2002;74:1276-1287.
9 Sanborn TA, Sleeper LA, Bates ER, et al. Impact of thrombolysis, intra-aortic balloon pump counterpulsation, and their combination in cardiogenic shock complicating acute myocardial infarction: a report from the SHOCK Trial Registry. Should we emergently revascularize Occluded Coronaries for cardiogenic shocK ? J Am Coll Cardiol. 2000;36:1123-1129.
10 Ohman EM, George BS, White CJ, et al. Use of aortic counterpulsation to improve sustained coronary artery patency during acute myocardial infarction: results of a randomized trial: the Randomized IABP Study Group. Circulation. 1994;90:792-799.
11 Talley JD, Ohman EM, Mark DB, et al. Economic implications of the prophylactic use of intraaortic balloon counterpulsation in the setting of acute myocardial infarction; the Randomized IABP Study Group, Intraaortic Balloon Pump. Am J Cardiol. 1997;79:590-594.
12 Stone GW, Marsalese D, Brodie BR, et al. A prospective, randomized evaluation of prophylactic intraaortic balloon counterpulsation in high-risk patients with acute myocardial infarction treated with primary angioplasty. Second Primary Angioplasty in Myocardial Infarction (PAMI-II) Trial Investigators. J Am Coll Cardiol. 1997;29:1459-1467.
13 Arafa OE, Geiran OR, Andersen K, et al. Intraaortic balloon pumping for predominantly right ventricular failure after heart transplantation. Ann Thorac Surg. 2000;70:1587-1593.
14 Vonderheide RH, Thadhani R, Kuter DJ. Association of thrombocytopenia with the use of intra-aortic balloon pumps. Am J Med. 1998;105:27-32.
15 Gott J. Intraaortic balloon pump, cardiopulmonary bypass. In: Mora C, editor. Principles and techniques of extracorporeal circulation. New York: Springer; 1995:416.
16 Meharwal ZS, Trehan N. Vascular complications of intra-aortic balloon insertion in patients undergoing coronary revascularization: analysis of 911 cases. Eur J Cardiothorac Surg. 2002;21:741-747.
17 Cohen M, Dawson MS, Kopistansky C, et al. Sex and other predictors of intra-aortic balloon counterpulsation-related complications: prospective study of 1119 consecutive patients. Am Heart J. 2000;139:282-287.
18 Jiang CY, Zhao LL, Wang JA, et al. Anticoagulation therapy in intra-aortic balloon counterpulsation: does IABP really need anti-coagulation ? J Zhejiang Univ Sci. 2003;4:607-611.
19 Papaioannou TG, Lekakis JP, Dagre AG, et al. Arterial compliance is an independent factor predicting acute hemodynamic performance of intra-aortic balloon counterpulsation. Int J Artif Organs. 2001;24:478-483.
20 Papaioannou TG, Mathioulakis DS, Nanas JN, et al. Arterial compliance is a main variable determining the effectiveness of intra-aortic balloon counterpulsation: quantitative data from an in vitro study. Med Eng Phys. 2002;24:279-284.
21 Ferguson JJ3rd, Cohen M, Freedman RJJr, et al. The current practice of intra-aortic balloon counterpulsation: results from the Benchmark Registry. J Am Coll Cardiol. 2001;38:1456-1462.
22 Krau SD. Successfully weaning the intra-aortic balloon pump patient: an algorithm. Dimen Crit Care Nurs. 1999;18:2-11.
23 Cohen M, Urban P, Christenson JT, et al. Intra-aortic balloon counterpulsation in US and non-US centres: results of the Benchmark Registry. Eur Heart J. 2003;24:1763-1770.
24 Frazier OH, Delgado RM. Mechanical circulatory support for advanced heart failure: where does it stand in 2003? Circulation. 2003;108:3064-3068.
25 Miller LW. Patient selection for the use of ventricular assist devices as a bridge to transplantation. Ann Thorac Surg. 2003;75:S66-S71.
26 Delgado DH, Rao V, Ross HJ, et al. Mechanical circulatory assistance: state of art. Circulation. 2002;106:2046-2050.
27 Entwistle JW3rd. Short- and long-term mechanical ventricular assistance towards myocardial recovery. Surg Clin North Am. 2004;84:201-221.
28 Hochman JS, Sleeper LA, Webb JG, et al. Early revascularization in acute myocardial infarction complicated by cardiogenic shock. SHOCK Investigators. Should We Emergently Revascularize Occluded Coronaries for Cardiogenic Shock. N Engl J Med. 1999;341:625-634.
29 Farrar DJ, Holman WR, McBride LR, et al. Long-term follow-up of Thoratec ventricular assist device bridge-to-recovery patients successfully removed from support after recovery of ventricular function. J Heart Lung Transplant. 2002;21:516-521.
30 Acker MA. Mechanical circulatory support for patients with acute-fulminant myocarditis. Ann Thorac Surg. 2001;71:S73-S76. discussion S82-S85
31 Morgan JA, John R, Rao V, et al. Bridging to transplant with the HeartMate left ventricular assist device: The Columbia Presbyterian 12-year experience. J Thorac Cardiovasc Surg. 2004;127:1309-1316.
32 Morgan JA, Park Y, Kherani AR, et al. Does bridging to transplantation with a left ventricular assist device adversely affect posttransplantation survival? A comparative analysis of mechanical versus inotropic support. J Thorac Cardiovasc Surg. 2003;126:1188-1190.
33 Aaronson KD, Eppinger MJ, Dyke DB, et al. Left ventricular assist device therapy improves utilization of donor hearts. J Am Coll Cardiol. 2002;39:1247-1254.
34 Gammie JS, Edwards LB, Griffith BP, et al. Optimal timing of cardiac transplantation after ventricular assist device implantation. J Thorac Cardiovasc Surg. 2004;127:1789-1799.
35 Rose EA, Gelijns AC, Moskowitz AJ, et al. Long-term mechanical left ventricular assistance for end-stage heart failure. N Engl J Med. 2001;345:1435-1443.
36 Bruckner BA, Razeghi P, Stetson S, et al. Degree of cardiac fibrosis and hypertrophy at time of implantation predicts myocardial improvement during left ventricular assist device support. J Heart Lung Transplant. 2004;23:36-42.
37 Kherani AR, Maybaum S, Oz MC. Ventricular assist devices as a bridge to transplant or recovery. Cardiology. 2004;101:93-103.
38 Aaronson KD, Patel H, Pagani FD. Patient selection for left ventricular assist device therapy. Ann Thorac Surg. 2003;75:S29-S35.
39 Pagani FD, Lynch W, Swaniker F, et al. Extracorporeal life support to left ventricular assist device bridge to heart transplant: a strategy to optimize survival and resource utilization. Circulation. 1999;100:II206-II210.
40 Ochiai Y, McCarthy PM, Smedira NG, et al. Predictors of severe right ventricular failure after implantable left ventricular assist device insertion: analysis of 245 patients. Circulation. 2002;106:I198-I202.
41 Nakatani S, Thomas JD, Savage RM, et al. Prediction of right ventricular dysfunction after left ventricular assist device implantation. Circulation. 1996;94:II216-II221.
42 Morgan JA, John R, Lee BJ, et al. Is severe right ventricular failure in left ventricular assist device recipients a risk factor for unsuccessful bridging to transplant and post-transplant mortality. Ann Thorac Surg. 2004;77:859-863.
43 Kavarana MN, Pessin-Minsley MS, Urtecho J, et al. Right ventricular dysfunction and organ failure in left ventricular assist device recipients: a continuing problem. Ann Thorac Surg. 2002;73:745-750.
44 Liao KK, Miller L, Toher C, et al. Timing of transesophageal echocardiography in diagnosing patent foramen ovale in patients supported with left ventricular assist device. Ann Thorac Surg. 2003;75:1624-1626.
45 Deng MC, Loebe M, El-Banayosy A, et al. Mechanical circulatory support for advanced heart failure: effect of patient selection on outcome. Circulation. 2001;103:231-237.
46 Khot UN, Mishra M, Yamani MH, et al. Severe renal dysfunction complicating cardiogenic shock is not a contraindication to mechanical support as a bridge to cardiac transplantation. J Am Coll Cardiol. 2003;41:381-385.
47 Song X, Throckmorton AL, Untaroiu A, et al. Axial flow blood pumps. Asaio J. 2003;49:355-364.
48 Saito S, Nishinaka T, Westaby S. Hemodynamics of chronic nonpulsatile flow: implications for LVAD development. Surg Clin North Am. 2004;84:61-74.
49 Letsou GV, Myers TJ, Gregoric ID, et al. Continuous axial-flow left ventricular assist device (Jarvik 2000) maintains kidney and liver perfusion for up to 6 months. Ann Thorac Surg. 2003;76:1167-1170.
50 Morgan JA, Stewart AS, Lee BJ, et al. Role of the Abiomed BVS 5000 device for short-term support and bridge to transplantation. Asaio J. 2004;50:360-363.
51 Magliato KE, Kleisli T, Soukiasian HJ, et al. Biventricular support in patients with profound cardiogenic shock: a single center experience. Asaio J. 2003;49:475-479.
52 Thomas CE, Jichici D, Petrucci R, et al. Neurologic complications of the Novacor left ventricular assist device. Ann Thorac Surg. 2001;72:1311-1315.
53 Strauch JT, Spielvogel D, Haldenwang PL, et al. Recent improvements in outcome with the Novacor left ventricular assist device. J Heart Lung Transplant. 2003;22:674-680.
54 Westaby S. Ventricular assist devices as destination therapy. Surg Clin North Am. 2004;84:91-123.
55 Westaby S, Banning AP, Jarvik R, et al. First permanent implant of the Jarvik 2000 Heart. Lancet. 2000;356:900-903.
56 Westaby S, Frazier OH, Beyersdorf F, et al. The Jarvik 2000 Heart: clinical validation of the intraventricular position. Eur J Cardiothorac Surg. 2002;22:228-232.
57 Goldstein DJ. Worldwide experience with the MicroMed DeBakey Ventricular Assist Device as a bridge to transplantation. Circulation. 2003;108(suppl 1):II272-II277.
58 Hetzer R, Weng Y, Potapov EV, et al. First experiences with a novel magnetically suspended axial flow left ventricular assist device. Eur J Cardiothorac Surg. 2004;25:964-970.
59 Thiele H, Lauer B, Hambrecht R, et al. Reversal of cardiogenic shock by percutaneous left atrial-to-femoral arterial bypass assistance. Circulation. 2001;104:2917-2922.
60 Copeland JG, Smith RG, Arabia FA, et al. Cardiac replacement with a total artificial heart as a bridge to transplantation. N Engl J Med. 2004;351:859-867.
61 Goldstein DJ, Beauford RB. Left ventricular assist devices and bleeding: adding insult to injury. Ann Thorac Surg. 2003;75:S42-S47.
62 Gordon SM, Schmitt SK, Jacobs M, et al. Nosocomial bloodstream infections in patients with implantable left ventricular assist devices. Ann Thorac Surg. 2001;72:725-730.
63 Holman WL, Rayburn BK, McGiffin DC, et al. Infection in ventricular assist devices: prevention and treatment. Ann Thorac Surg. 2003;75:S48-S57.
64 Pasque MK, Hanselman T, Shelton K, et al. Surgical management of Novacor drive-line exit site infections. Ann Thorac Surg. 2002;74:1267-1268.
65 Lazar RM, Shapiro PA, Jaski BE, et al. Neurological events during long-term mechanical circulatory support for heart failure: the Randomized Evaluation of Mechanical Assistance for the Treatment of Congestive Heart Failure (REMATCH) experience. Circulation. 2004;109:2423-2427.
66 Horton SC, Khodaverdian R, Chatelain P, et al. Left ventricular assist device malfunction: an approach to diagnosis by echocardiography. J Am Coll Cardiol. 2005;45:1435-1440.
67 Horton SC, Khodaverdian R, Powers A, et al. Left ventricular assist device malfunction: a systematic approach to diagnosis. J Am Coll Cardiol. 2004;43:1574-1583.
68 Ferns J, Dowling R, Bhat G. Evaluation of a patient with left ventricular assist device dysfunction. Asaio J. 2001;47:696-698.
69 Scalia GM, McCarthy PM, Savage RM, et al. Clinical utility of echocardiography in the management of implantable ventricular assist devices. J Am Soc Echocardiog. 2000;13:754-763.
70 Zwischenberger JB, Bartlett RH. Extracorporeal Cardiopulmonary Support in Critical Care, ed 3, Ann Arbor, MI: Extracorporeal Life Support Organization, 2005.
71 Morris AH, Wallace CJ, Menlove RL, et al. Randomized clinical trial of pressure-controlled inverse ratio ventilation and extracorporeal CO 2 removal for adult respiratory distress syndrome. Am J Respir Crit Care Med. 1994;149:295-305.
72 Zapol WM, Snider MT, Hill JD, et al. Extracorporeal membrane oxygenation in severe acute respiratory failure: a randomized prospective study. JAMA. 1979;242:2193-2196.
73 Lewandowski K, Rossaint R, Pappert D, et al. High survival rate in 122 ARDS patients managed according to a clinical algorithm including extracorporeal membrane oxygenation. Intens Care Med. 1997;23:819-835.
74 Linden V, Palmer K, Reinhard J, et al. High survival in adult patients with acute respiratory distress syndrome treated by extracorporeal membrane oxygenation, minimal sedation, and pressure supported ventilation. Intens Care Med. 2000;26:1630-1637.
75 Mols G, Loop T, Geiger K, et al. Extracorporeal membrane oxygenation: a ten-year experience. Am J Surg. 2000;180:144-154.
76 Ullrich R, Lorber C, Roder G, et al. Controlled airway pressure therapy, nitric oxide inhalation, prone position, and extracorporeal membrane oxygenation (ECMO) as components of an integrated approach to ARDS. Anesthesiology. 1999;91:1577-1586.
77 Doll N, Kiaii B, Borger M, et al. Five-year results of 219 consecutive patients treated with extracorporeal membrane oxygenation for refractory postoperative cardiogenic shock. Ann Thorac Surg. 2004;77:151-157.
78 Smedira NG, Moazami N, Golding CM, et al. Clinical experience with 202 adults receiving extracorporeal membrane oxygenation for cardiac failure: survival at five years. J Thorac Cardiovasc Surg. 2001;122:92-102.
79 Hemmila MR, Rowe SA, Boules TN, et al. Extracorporeal life support for severe acute respiratory distress syndrome in adults. Ann Surgery. 2004;240:595-605. discussion 605-607
80 Kolla S, Awad SS, Rich PB, et al. Extracorporeal life support for 100 adult patients with severe respiratory failure. Ann Surg. 1997;226:544-566.
81 Rich PB, Awad SS, Crotti S, et al. A prospective comparison of atrio-femoral and femoro-atrial flow in adult venovenous extracorporeal life support. J Thorac Cardiovasc Surg. 1998;116:628-632.
82 Pranikoff T, Hirschl RB, Remenapp R, et al. Venovenous extracorporeal life support via percutaneous cannulation in 94 patients. Chest. 1999;115:818-822.
83 Ceviz M, Ozyazicioglu A, Kocak H. The prevention of leg ischemia in femoral cannulation. Ann Thorac Surg. 1999;67:1217.
84 Peek GJ, Killer HM, Reeves R, et al. Early experience with a polymethyl pentene oxygenator for adult extracorporeal life support. Asaio J. 2002;48:480-482.
85 Seib PM, Faulkner SC, Erickson CC, et al. Blade and balloon atrial septostomy for left heart decompression in patients with severe ventricular dysfunction on extracorporeal membrane oxygenation. Cathet Cardiovasc Interven. 1999;46:179-186.
86 Kasirajan V, Smedira NG, McCarthy JF, et al. Risk factors for intracranial hemorrhage in adults on extracorporeal membrane oxygenation. Eur J Cardiothorac Surg. 1999;15:508-514.
87 Burket JS, Bartlett RH, Vander Hyde K, et al. Nosocomial infections in adult patients undergoing extracorporeal membrane oxygenation. Clin Infect Dis. 1999;28:828-833.
88 Bartlett RH. Management of ECLS in adult respiratory failure. In: Meurs KV, Lally KP, Peek G, Zwischenberer JB, editors. Extracorporeal Cardiopulmonary Support in Critical Care. ed 3. Ann Arbor, MI: Extracorporeal Life Support Organization; 2005:403-416.
89 Frazier OH, Myers TJ, Gregoric ID, et al. Initial clinical experience with the Jarvik 2000 implantable axial-flow left ventricular assist system. Circulation. 2002;105:2855-2860.