Chapter 10 Management of Recurrent Gliomas
Confirmation of Recurrence
Differential Diagnosis
• A distinctly new tumor may arise at the site of an eradicated tumor. This is more likely to occur if there is a genetic predisposition to tumor development shared by cells in the area; for example, multiple gliomas may occur in a patient with tuberous sclerosis.
• A tumor of related histology may supplant the original tumor; for example, the astrocytic component may replace the oligodendrocytic component as the predominant subtype of a mixed glioma, or a gliosarcoma may arise from a previously treated glioblastoma.
• The initial therapy may induce a secondary tumor of a different type; for example, a glioblastoma in the radiation field of a low-grade glioma.
• Non-neoplastic lesions may mimic tumor growth; for example, an abscess or granuloma at the site of resection of a tumor induced by treatment of the original tumor, or radiation necrosis following focal high-dose irradiation.1,2
Malignant Progression
The first scenario is the renewed growth of a low grade tumor. When low grade gliomas regrow after therapy, approximately half remain nonanaplastic, but the other 50% have progressed to a more malignant form.3 Molecular analyses have delineated genetic correlates of this progression.4 Enlarging low grade tumors will usually resemble the original tumor on imaging studies. When progression in grade has occurred, the new tumor may also resemble the old one, especially if the original tumor enhanced with contrast. Enhancement is highly predictive of likelihood of recurrence; low grade enhancing tumors are 6–8 times more likely to recur than nonenhancing ones.3 Most commonly, new malignant growth in a previously nonenhancing glioma enhances and thus is readily identified. In one study, only 30% (16/42) of low-grade tumors enhanced initially, but 92% (22/24) enhanced at recurrence.3 Occasionally, an enlarging malignant focus may not enhance. It might, however, be apparent as a region of hypermetabolism on a 2-deoxyglucose or 11-C methionine PET study, or have an increased rate of enhancement on a dynamic MRI scan, increased activity on a dual-isotope, single-photon emission computerized tomogram (SPECT), or increased choline signal on magnetic resonance spectroscopy (MRS).5–7 The differential specificity of each of these new modalities is approximately 80% to 90%. Usually, however, histologic analysis after biopsy or resection is warranted to verify malignant transformation.8
Radiation Effects
The second scenario that causes diagnostic difficulty is renewed enlargement of a tumor mass following radiation. Often, CT and MR imaging inadequately distinguish recurrent tumor from radiation-induced enlargement. Usually only large, very malignant tumors grow sufficiently fast to show significant enlargement during, or within 3 months of completing, a course of radiation. When this does occur, the prognosis is particularly poor.9
Radiation can cause tumor enlargement in three ways:1 through an early reaction, occurring during or shortly after irradiation, which is likely to be edema;2 through an early delayed reaction arising a few weeks to a few months after radiation which involves edema and demyelination; and3 through a late delayed reaction that occurs 6 to 24 months after radiation and reflects radiation-induced necrosis.10 Regional teletherapy to a dose of 60 Gy is the current standard radiation treatment for most gliomas.11 Although these doses have a low risk of inducing radiation necrosis, regional early and early delayed effects are relatively common. In most cases, tissue swelling represents edema and is transient. Acute symptoms from early and early delayed effects of radiation usually respond quickly to a short course of corticosteroids. The low density, T1 hypointense, T2 hyperintense regions of edema correspond to the area irradiated. Chronically, these volumes of brain will demonstrate parenchymal atrophy, enlargement of subarachnoid spaces, and ex vacuo ventricular dilatation. Dementia with apathy, inanition, and memory loss and decline in fine motor control are the clinical correlates. In the absence of new tumor growth, enhancement on CT and MRI beyond the initial resection margin is infrequent; when it does occur, it is patchy, irregularly marginated, and it can be distinguished from the more focal appearance of recurrent tumor.
In contrast, the late delayed effect of radiation-induced necrosis appears at about the time malignant tumors might be expected to recur.12 It is thus more likely to be mistaken for recurrent tumor growth. The risk of radiation necrosis increases with the volume of tissue treated, the dose delivered, and the fraction size.13 Radiation necrosis following fractionated treatment to doses less than 70 Gy is rare, but it is much more common following brachytherapy or radiosurgery, which deliver high doses of radiation to relatively small volumes over a short time period.12,14,15 A common protocol for brachytherapy is a 50- to 60-Gy boost (to 60 Gy of regional external beam radiotherapy) to a 0- to 5-cm tumor delivered over approximately 1 week. The radiosurgery equivalent is a 10- to 20-Gy boost to a 0- to 3-cm diameter tumor delivered in less than 1 hour.16 Necrosis is radiographically and pathologically evident in almost all cases and symptomatic in about half.
Whether it arises from higher doses of fractionated radiotherapy, brachytherapy, or radiosurgery, radiation necrosis is often difficult to distinguish from recurrent tumor. It forms a ring contrast-enhancing mass that resembles a malignant tumor. It has a CT hypodense, T1 hypointense, T2 hyperintense center; an enhancing annular region; and a hypodense, T1 hypointense, T2 hyperintense surround. The surround corresponds to edema that strikingly radiates along white matter tracts. The similarity of this appearance to that of recurrent tumors and the time course of its occurrence frequently necessitates additional measures to differentiate radiation-induced necrosis from recurrent tumor. A variety of functional neurodiagnostic imaging techniques attempt to distinguish between these two possibilities. These include PET scans, SPECT studies, cerebral blood volume mapping, and MRS. Regions of high activity are thought to distinguish recurrent tumor from relatively metabolically inactive and hypovascular radiation necrosis.6 Although specificity in differentiating tumor from radiation necrosis of up to 100% has been claimed, in many cases these studies are inconclusive and the diagnosis is revealed either by the clinical course or by analysis of a pathology specimen.
When an enlarging mass, which is either recurrent tumor, radiation necrosis, or both, becomes symptomatic, corticosteroid therapy is required.17 Up to half the patients receiving brachytherapy and radiosurgery for a malignant glioma develop symptoms that either prove refractory to corticosteroids or require debilitating long-term steroid use.12,18,19 Surgery for resection of an enlarging, symptomatic mass is needed in 20% to 40% of cases following brachytherapy or radiosurgery of a malignant glioma. At reoperation for presumed radiation necrosis following focal radiation treatment of a malignant glioma, necrosis without tumor was found in 5% of cases, tumor alone in 29%, and a mixture of radiation necrosis and tumor in 66%.12 In almost all cases, the tumor that is seen is of reduced viability.20,21
Causes of Recurrence
Renewed growth of a brain tumor following surgery and possibly radiation and chemotherapy indicates failure of these therapies to reduce the tumor mass to a size and cell number that would permit its eradication by the patient’s immune system (Fig. 10-1).22 Failure arises from a number of factors that limit the efficacy of each modality.
Recurrence after Surgery
Surgery may fail because of anatomic considerations, pathologic features, or errors in judgment or technique. The involvement of critical structures may limit the initial resection: involvement of the optic pathways, diencephalon, internal capsule, brain stem, or eloquent cortex by a glioma often precludes complete removal. Tumor recurrence, despite removal of all macroscopically evident tumor, can occur if there is microscopic infiltration of adjacent structures. Even low grade cerebral gliomas are usually infiltrative. Anaplastic astrocytomas characteristically are widely invasive. Finally, errors in judgment, such as preoperatively underestimating the amount of tumor that can be safely removed or intraoperatively failing to remove tumor that was targeted, result in potentially resectable tumor being left as a nidus of regrowth.
Recurrence after Radiation
Radiation therapy may fail because of inadequate targeting, underutilization of tolerable dose, or radiation resistance of the tumor cells. Proximity to critical anatomical structures can also limit maximal allowable radiation dosage. Furthermore, the correlations between imaging abnormality and tumor extent are incomplete. Pathologic studies have shown that individual tumor cells can be found throughout and even beyond CT hypodense and MRI T2 hyperintense areas of malignant glioma.23,24 For each individual case, the extent of brain invasion from the contrast-enhancing margin of a malignant glioma is incompletely known. The choice of field size for irradiation of such lesions is difficult and relies as much on the trade-off between target volume and tolerable dose as on the accurate delineation of tumor boundaries. Failure to include an adequate annulus of tissue about the tumor to accommodate imaging uncertainty and technical error may leave tumor cells incompletely irradiated.
Even if the maximal dose tolerated by infiltrative surrounding brain is delivered, tumor cells may remain viable. Hypoxic, nonproliferating cells and tumor stem cells are particularly radioresistant; with time or change in the physiologic conditions following therapy, re-entry of cells into the cell cycle permits the proliferation that results in clinically apparent tumor recurrence.25,26 Analysis of patterns of failure demonstrates that, even with maximal tolerable doses of photon radiation of 70 to 80 Gy, almost all malignant astrocytomas fail centrally.27 A study of high dose fractionated proton irradiation following radical resection of glioblastomas showed the following:1 a dose between 80 and 90 Gy is sufficient to prevent tumor regrowth;2 outside of this high dose volume, tumor regrows, usually in areas receiving between 60 and 70 Gy;3 enlargement of the high dose volume to include more peripheral areas is likely to induce unacceptably high levels of symptomatic radiation-related necrosis.28
Recurrence after Chemotherapy
Chemotherapy fails as a result of inadequate drug delivery, toxicity, or cell resistance. The blood–brain barrier is deficient in the contrast-enhancing region of the tumor, but surrounding brain usually has an intact blood–brain barrier; lipid-insoluble drugs thus have limited access to tumor cells infiltrating peripheral regions. The margin between drug efficacy and neurotoxicity, bone marrow suppression, pulmonary injury, and gastrointestinal side-effects is often narrow. Non-cycling cells and tumor stem cells are resistant to cell-cycle specific drugs, and potentially vulnerable cells often rapidly develop biochemical means of resistance to chemotherapeutic agents.25,26,29
Even if these therapies significantly reduce the tumor burden, the patient’s immune response may be rendered ineffective by chemotherapy and by the tumor’s secretion of factors antagonistic to immune function, such as IL-10, prostaglandin E2, and transforming growth factor (TGF)-beta 2, and its expression of apoptosis-inducing molecules, such as Fas ligand (FasL) and galectin-1.30 Each of these limitations of each component of multimodality therapy may contributes to failure to prevent tumor regrowth. At the time of tumor recurrence, consideration of these reasons for failure is essential to assessment of prognosis and to the choice of subsequent therapy.
Prognostic Implications of Residual and Recurrent Tumor
Residual Tumor
Cytoreductive surgery is a fundamental part of the treatment of most systemic malignancies.31 In most cases, there is a strong relationship between the extent of resection and outcome. For gliomas, correlation between extent of resection or, more significantly, size of residual tumor, and outcome measures, such as interval to tumor progression and survival, has been strongly suggested by retrospective and prospective series, but not by randomized clinical trials.32 More recently, one study, reported a significant survival advantage when 98% or greater resection is achieved.33
Correlation of survival with extent of resection for low grade gliomas has been suggested by retrospective uncontrolled reviews and comparisons with historical controls.3,34–36 One study of 461 adult patients with low grade cerebral gliomas found that gross total surgical removal correlated with length of survival.37 Another reported median survival duration of 7.4 years following maximal surgical resection. The median survival of a subgroup patients with hemispheric tumors compared favorably (10 years vs. 8 years) with that of a comparable series treated with biopsy and radiation alone.3,35 Additional studies have demonstrated that extensive resection of low grade gliomas delays tumor recurrence.36,38
For high grade gliomas, the correlations between the extent of resection at the initial operation and1 the time to tumor recurrence and2 the duration of patient survival have been disputed.39 Historical reports and reviews of large series have noted the association of survival and extent of resection for both astrocytomas and oligodendrogliomas.11,40–43 Extensive reviews of the literature, however, have failed to locate randomized, controlled clinical trials comparing survival after biopsy with that after radical resection of malignant gliomas.44,45 Nevertheless, the benefit of surgical cytoreduction has been strongly suggested by the following findings:
1. Reviews of multicentered trials have shown that the more complete the resection, the longer the patient lived.46–48
2. In a study of 243 patients, multivariate regression analysis identified extent of resection as an important prognostic factor (p < 0.0001) for survival.49
3. In a retrospective review of 1215 patients with WHO grade III or IV, increasing extent of surgical resection was associated with improved survival independent of age, degree of disability WHO grade, or subsequent treatment modalities used.50
4. Single center studies have confirmed this relationship: in one study containing 21 glioblastomas and 10 anaplastic astrocytomas, median duration of survival after gross total resection was 90 weeks versus only 43 weeks following subtotal resection, and the 2-year survival rates were 19% and 0%, respectively, even though the two groups were well matched for other prognostically significant variables;34,51 in another study, patients with gross total resection of malignant glioma lived longer (76 vs. 19 weeks) than those who underwent only a biopsy, even after correction for tumor accessibility and all other prognostically significant variables52 and one large recent series showed that GTR (>98%) significantly increases the duration of survival.33
5. In two larger series, patients with resected cortical and subcortical grade IV gliomas lived longer (50.6 vs. 33.0 weeks53 and 39.5 vs. 32.0 weeks54 after surgery and radiation than those who underwent biopsy and radiation.
6. Small postoperative tumor volume has been shown to correlate with longer time to tumor progression after surgery55 and longer patient survival.56,57
Recurrent Tumor
Regrowth of tumors after an initial response (diminution or stability) to surgery and radiation therapy is ominous. This is particularly true if the growth is more rapid or more infiltrative than that of the original tumor. Such growth often exhibits changes in the basic biology of the tumor that make it less responsive to subsequent therapy. A short interval between initial treatment and recurrence of symptoms often indicates rapid regrowth and a poor prognosis. Factors to be considered in estimating prognosis include the biology of the tumor (its pathology, growth rate, and invasiveness), its resectability, its prior response to radiation and chemotherapy, and the age and performance status of the patient.58 Estimates of the recurrent tumor’s size, growth rate, invasiveness, and location must be made in assessing its potential for causing both neurologic deficit and death. Reappearance of a slowly growing, well-demarcated frontal convexity oligodendroglioma with deletions of chromosomes 1p and 19q in a middle-aged patient of good neurologic condition after a 10-year interval of postsurgical quiescence clearly carries a prognosis very different from that of diffuse diencephalic spread of a glioblastoma multiforme in an elderly patient with a poor performance status 3 months after treatment with surgery, radiation, and chemotherapy.59
Therapy of Recurrent Glial Tumors
Therapy of Recurrent Gliomas
The choice of therapy of a recurrent glioma is based on a comparison of the natural history of the regrowing tumor with the risk–benefit ratio of potential therapies. Recurrent gliomas warrant aggressive multimodality therapy if the patient is in good neurologic and general medical condition and therapeutic options offer a realistic chance for significant improvement in neurologic status or extension of survival.60
Patterns of Recurrence of Gliomas
When gliomas recur, most do so locally. Historically, more than 80% of recurrent glioblastoma multiforme arose within 2 cm of the original margin of contrast-enhancing tumor.61,62 In one series, over 90% of glioma cases showed recurrence at the original tumor location, while 5% developed multiple lesions after treatment.63 In another study of 36 patients with malignant gliomas receiving 70 to 80 Gy of fractionated radiation, 32 (89%) had central (at least 95% of the recurrent tumor within the volume receiving at least 95% of the maximum dose) or in-field (at least 80% of tumor within this highest dose volume) recurrence, and 3 (8%) had marginal recurrence. Only one (3%) fell predominantly outside the high dose range. Seven patients had multiple sites of recurrence, but only one had a large recurrence outside the high dose volume.27 This tendency to recur locally is a function of tumor cell distribution. There is a gradient of tumor cell density in which tumor cell number decreases rapidly at increasing distances from the contrast-enhancing rim of solid tumor. Thus, although individual tumor cells spread through the brain at great distances from the primary site, there are so many more cells locally that the odds favor local reaccumulation of tumor mass.23,24
Factors contributing to the likelihood of local recurrence include the following:
1. Relative predominance of tumor cell mass in the region
2. Statistical likelihood that a local cell will be the cell that first develops a competitive proliferative advantage
3. Possibility that the physiologic milieu (hypervascularity and increased permeability, disrupted tissue architecture, and paracrine growth factor stimuli) at the site is particularly conducive to regrowth.
As tumor cell proliferation resumes at the initial tumor site, cells again spread rapidly and diffusely. Tumor cell proliferation resumes at distant sites as a result of the influx of these new, mitotically active cells or the renewed growth of cells that spread before the initial treatment.63 Biologic therapeutic agents may also affect the pattern of recurrence; recent experience with bevacizumab (Avastin) suggests that tumors treated with this inhibitor of VEGF are more likely to recur as diffusely infiltrative lesions distant from the original site of tumor.64 Consequently, treatments targeting local recurrence alone will, at best, be briefly palliative. Treatment of tumor recurrence thus usually involves a combination of modalities aimed at both local and distant disease.
Epidemiology of Recurrent Glioblastoma
The heterogeneity in defining recurrence and the variability of treatment algorithms employed at different institutions result in a vague profile of recurrent glioblastoma multiforme.65 In a multi-center trial of reoperation for resection and placement of cavitary biodegradable BCNU-wafer in 222 patients with recurrent glioblastoma and a Karnofsky Performance Score of at least 60, the median interval from initial diagnosis to tumor recurrence was 12 months.66 Among a cohort of 301 patients with GBM, 223 patients had tumor recurrence at a median interval from initial diagnosis of 4.9 months;67 64% of these had a Karnovsky Performance Score greater than 70 at the time of recurrence.
Glioblastoma recurrence is demonstrated on imaging obtained in routine surveillance or in response to new or recurring symptoms. In a questionnaire-based study of patients with recurrent glioblastoma or anaplastic astrocytoma and a KPS >70, self-reported symptoms included fatigue, uncertainty about the future, motor difficulties, drowsiness, communication difficulties, and headache.68 While most symptoms likely reflected tumor recurrence, confounding factors such as radiation necrosis and steroid treatment may have contributed to generalized fatigue, and pain and uncertainty about the future may have resulted from the diagnosis alone, independent of current tumor status. Incoordination, weakness, visual loss, and pain were reported more frequently by patients with recurrent glioblastoma than by those with anaplastic astrocytoma, providing evidence that more aggressive disease will cause greater neurological deficit.
Therapy of Recurrent Malignant Glioma
Choice of therapy for a recurrent glioma must consider the tumor’s current and previous histology, previous treatment, and location and the patient’s age, medical and neurologic conditions, and preferences. An enlarging lesion that was originally a low grade glioma should undergo biopsy (stereotactically or, if resection is anatomically feasible, by open craniotomy) to confirm histology (Fig. 10-2). If the tumor remains low grade and a large part of the lesion can be resected without inflicting significant neurologic deficit, it should be removed; if previously irradiated to significantly less than maximal tolerable dose, the tumor bed and surrounding area should receive fractionated radiotherapy. The longer the interval since the initial radiotherapy, the higher the dose that can be given safely at recurrence. If the tumor is inaccessible to surgery, radiation alone should be prescribed. If a low grade tumor previously irradiated to a maximal tolerable dose recurs as a low grade glioma, it should be resected, if possible. If it is inaccessible, stereotactically delivered focal radiation is an attractive option.69,70
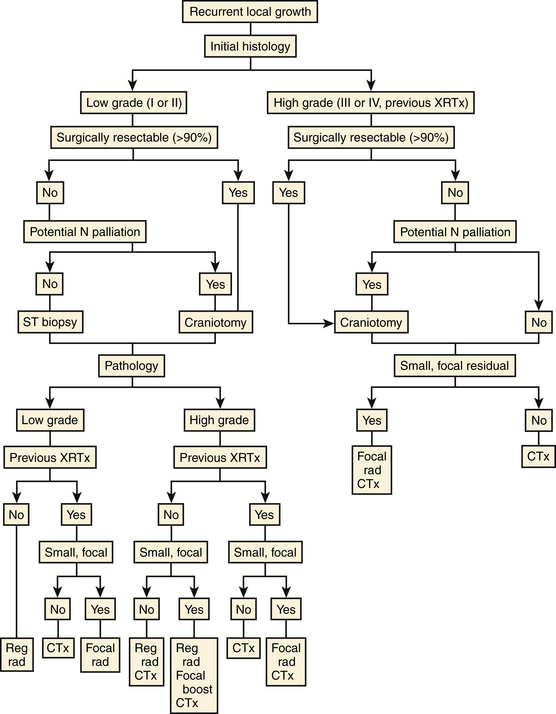
FIGURE 10-2 Recurrent glioma. Decisions in the management of a recurrent tumor should consider grade, resectability, and prior therapy. N, neurologic; reg rad, regional fractionated radiation therapy; focal rad/boost, stereotactic radiosurgery, brachytherapy, or radiotherapy; small, focal, less than 10 cm3, radiographically demarcated; CTx, chemotherapy; ST, stereostatic; XRTx, radiotherapy.
Surgery
If the low grade tumor recurs as a high grade tumor or if a high grade tumor recurs, reoperation should be attempted if the patient has a Karnofsky score of at least 70 and removal of all or almost all of the contrast-enhancing tumor is potentially attainable, or if the tumor mass is causing neurologic symptoms that might be palliated by its reduction. Removal of tumor may improve the patient’s quality of life by alleviating neurologic deficit or permitting reduction of steroid dose. It may also prolong survival by reducing tumor burden and improving response to radiation, chemotherapy, immunotherapy, or biologic therapy.71,72
Radiation Therapy
If the tumor was not irradiated previously, the tumor bed and its annular margin should receive regional radiotherapy. Even when radiotherapy has been used initially, it is an option at recurrence, but doses permitted under standard guidelines for conventional fractionation and volumes are unlikely to be high enough to be effective. Other possibilities include highly conformal conventionally fractionated radiotherapy (e.g., IMRT, intensity modulated radiation therapy), hypofractionated stereotactic radiotherapy, interstitial brachytherapy, and stereotactic radiosurgery.72–76 In one study, the use of highly conformal teletherapy for re-irradiation of recurrent gliomas (mean re-irradiation dose of 38 Gy, range 30.6–59.4 Gy) at a median time of 38 months (range 9–234 months) produced radiographic stability or regression and neurologic improvement in two thirds of the patients.75 In another study, 10 patients with recurrent malignant gliomas treated with intensity-modulated radiation therapy (daily fractions of 5 Gy to a total median dose of 30 Gy) demonstrated median overall survival duration of 10.1 months from the time of treatment, with 1- and 2-year survival rates of 50% and 33%, respectively.76
Hypofractionated stereotactic radiotherapy (SRT) combines high dose per fraction with stereotactic targeting. Hypofractionated SRT (e.g., 20–30 Gy in 2–5-Gy fractions) of recurrent malignant gliomas resulted in a median duration of subsequent survival of 9.3 months (15.4 months for grade III tumors and 7.9 months for grade IV tumors) in one study.77 Another protocol that delivered 20 to 50 Gy in 5-Gy fractions to 29 patients with recurrent high grade astrocytomas resulted in a median duration of survival after retreatment of 11 months.78 Steroid dependent toxicity occurred in 36% of patients, reoperation was required in 6%, and a total dose in excess of 40 Gy predicted radiation damage (p < 0.005). Another study used 24 Gy in 3 Gy fractions, 30 Gy in 3 Gy fractions, and 35 Gy in 3.5 Gy fractions to boost previously irradiated residual or recurrent malignant gliomas at a mean of 3.1 months (range 1–46 months) after standard treatment to 60 Gy. Sixty percent of patients required less steroid and 45% improved neurologically. Eighty percent of those receiving 30 or 35 Gy responded. The median duration of survival was 10.5 months. No grade 3 toxicity occurred. Reoperation was not performed.79 A fourth protocol combined fractionated stereotactic radiosurgery and taxol as a radiosensitizer for recurrent malignant gliomas. It resulted in median survival duration of 14.2 months in 14 selected patients.80 These four studies suggest that hypofractionated SRT has moderate efficacy and acceptable safety in selected patients.
Although several studies suggested promise for brachytherapy in treating glioblastomas, both initially and at the time of recurrence, tumors in these studies were highly selected for small size and focality, two features that would make them appropriate for stereotactic radiosurgery or radiotherapy, less invasive techniques with equivalent results.12,81,82 In a retrospective comparison of interstitial brachytherapy and radiosurgery for recurrent glioblastomas, the median durations of survival in the two groups were similar (11.5 months and 10.2 months, respectively). Patterns of failure were similar. The actuarial risks of reoperation for necrosis at 12 and 24 months were 33% and 48% respectively, after radiosurgery, and 54% and 65%, respectively, after brachytherapy, with the caveat that the brachytherapy group had larger tumors and longer follow-up.83,84
Other forms of focal radiation therapy of recurrent gliomas such as photodynamic therapy (PDT), boron neutron capture therapy (BNCT), intraoperative radiation (IORT), and radiolabeled monoclonal antibodies to tumor cells’ surface receptors have been studied.85–90 A dozen clinical trials have measured the safety and efficacy of irradiation of a tumor’s resection cavity by 131I-labeled mAb 81C6 (anti-tenascin C). Promising results for this technique during initial resection suggest its potential utility during reoperation as well.90
Chemotherapy
Chemotherapy of infiltrative gliomas at diagnosis and at recurrence is often valuable. Temozolamide (Temodar, TMZ), an oral DNA-methylating agent with a benefit-toxicity profile superior to that of antecedent alternative intravenous alkylating agents such as BCNU and CCNU, has become the drug of choice. TMZ is appropriate for those low grade tumors not treated by chemotherapy at the time of initial presentation; one study reported a response rate of 47%.91 If the tumor recurs despite TMZ, a protracted schedule of TMZ administration or strategies using CCNU alone, PCV, bevacizumib (Avastin) alone or bevacizumib combined with other drugs (e.g., Irinotecan) can be given.64,92–95
For Grade III tumors, TMZ is also recommended.96 Perhaps reflective of the favorable biology of the tumor, TMZ of anaplastic oligodendrogliomas with deletions of chromosomes 1p and 19q is particularly effective.59,97 In the initial treatment of patients with GBM, the addition of TMZ to radiotherapy increases the percentage of patients surviving at 2 years to 26.5% from 10.4% for radiotherapy alone and the median duration of survival to 14.6 months from 12.1 months.98 At the time of renewed growth of a high grade tumor, if the tumor has not previously been exposed to TMZ, TMZ should be given. If TMZ had been used but it was discontinued prior to either tumor progression or treatment-limiting toxicity occurring, rechallenge with TMZ or a single nitrosurea agent would be appropriate.99 Patients treated for recurrent or progressive GBM with TMZ showed an overall response rate of 19% and mean time to progression of 11.7 weeks.99 Similarly, treatment of recurrent GBM with a standard TMZ regimen (150 to 200 mg/m2 × 5 days in 28-day cycles) produced a progression-free survival rate of 6 months (PFS-6) of 21% vs. 8% following procarbazine.100 A more intensive regimen (150 mg/m2 daily on a week on/week off cycle) yielded a PFS-6 of 48% with an overall PFS-12 of 81%101 and combinations of TMZ with the matrix metalloproteinase inhibitor, marimastat, or 13-cis-retinoic acid, produced rates of PFS-6 of 39% and 32%, respectively.102 If TMZ was used to treat the primary tumor and toxicity occurred, then an agent with a different toxicity risk profile should be used.95