Chapter 134 Management of Penetrating and Blast Injuries of the Nervous System
Historical Aspects
The basic principles used in the management of penetrating brain injuries (PBIs) were developed while treating casualties during the wars of the past century. Modern antiseptic techniques were first applied to head injured patients during the Anglo-Boer war.1 During World War I, Harvey Cushing implemented en-block bone resection, suction debridement, magnet removal of metallic fragments, and two-layered closure of penetrating head injuries to effect a decrease in mortality from 54% to 28%.2,3 During World War II, widespread use of early antibiotics dramatically reduced rates of infection.4–7 Korean War experiences emphasized the role of early evacuation of patients with head injuries.8
In the Vietnam conflict, a wealth of experience and data were obtained, often in a prospective fashion.9 Routine use of skull x-rays and angiography allowed better visualization of penetrating bone and metallic fragments, which were thought to be associated with increased rates of infection and seizures. Military medical doctrine advocated the pursuit of all retained bone fragments even when this entailed multiple operations.9 This dogma was later questioned when follow-up studies of Vietnam veterans with retained fragments failed to find increased rates of either infectious complications10 or epilepsy.11
The routine international use of diagnostic computed tomography (CT) scanning coincided with the Arab-Israeli conflict in Lebanon. Its use in medical decision making prompted a less aggressive initial surgical approach in the absence of traumatic mass effect.12 Long-term analysis of Israeli survivors found 48% had retrained in-driven intracranial bone and further questioned the need to aggressively remove all penetrating fragments.13 The Iran-Iraq war also led to a wealth of published data, including reports on the causes of infections and the vascular complications of penetrating head injuries.14–16 Closed-head-injury data from bomb blasts in the Lebanon conflict17 and more recent reports from the Iraq Conflict18 emphasize the complexity of blast injuries, which combine the elements of both penetrating and blast effects.
Ballistics
The kinetic energy (KE) released by a missile fragment is related to its mass (M) and velocity (V) and may be estimated by the formula19:
This equation highlights the importance of missile velocity relative to mass. Most modern high-velocity rifles surpass 2500 ft/sec (762 m/sec), whereas most handguns fire 800 to 1400 ft/sec (244 to 427 m/sec). Shrapnel typically has a velocity of 600 feet/second (183 m/sec).20 A missile’s energy is greatest at the moment it is launched, and decays with time and distance. Therefore, it is the impact velocity of the projectile that more accurately reflects the true wounding potential of the missile Fig. 134-1. The actual energy delivered to tissue is determined not just by the kinetic energy available to the projectile (as expressed by the above equation) but also by the deformation and fragmentation of the missile itself.21 Weapons and ammunition are designed to create friction-free flight in air but to enhance resistance to passage in tissue. Expansion, tumbling, yaw, and fragmentation all enhance energy delivery to the tissue.20 Bone fragments driven into neural elements may act as secondary missiles. Thus even a tangential missile strike can achieve a release of energy leading to significant injury to the central nervous system (CNS).
Pathophysiology
The biomechanical effects of a missile strike on tissue were originally described in 1945 by E. Newton Harvey22 and more recently simulated in cold gel blocks by Martin Fackler and colleagues.23 Carey utilized a cat model to study the wounding effects of a projectile through an intact skull.24 First, a direct crush injury results in a permanent cylindrical cavity. Pressure waves resulting from kinetic energy transfer, termed “ordinary pressure waves,” are propelled radially from the missile path. These create a temporary cavity lasting approximately 20 milliseconds as the walls of the permanent cavity first expand, then contract. The ordinary pressure waves sometimes result in stretch injury to adjacent tissues even at distances far removed from the projectile tract. Collapse of the temporary cavity is followed by extravasation of blood from around the missile track, which may expand to occupy a much larger space than the initial cavity Figs. 134-2 and 134-3. In perforating injuries, the exit wound is inevitably larger than the entrance wound.
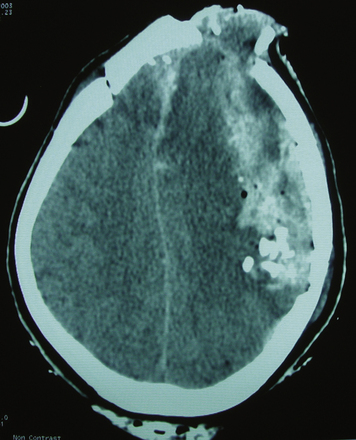
FIGURE 134-2 Axial computed tomography from patient in Fig. 134-1. Note hemorrhage into the temporary projectile cavity with surrounding contusions from stretch injury.
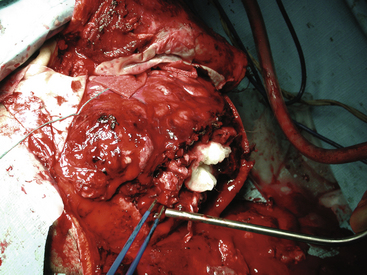
FIGURE 134-3 Intraoperative photo from patient in Fig. 134-1. Massive tissue injury and swelling are seen after high-velocity gunshot wound through the left hemisphere of the brain.
Animal models have been utilized to study the effects of a missile strike to the brain on the physiologic parameters of intracranial pressure (ICP), mean arterial blood pressure (MABP), and cerebral perfusion pressure (CPP). In a monkey model, Crockard and colleagues25 showed that a rapid rise in ICP immediately follows penetrating injury, peaking 2 to 5 minutes after injury and declining gradually thereafter. Carey and colleagues,26 utilizing his feline model, demonstrated that a marked rise in MABP in the first minute after injury actually results in a short-lived rise in CPP; however, the rise in ICP is more sustained over time and larger in magnitude relative to baseline values than the rise in MABP, and it leads to a reduction in CPP. Levett and colleagues, using Crockard’s model, showed that CPP values fell within 1 minute of injury to 41 mm Hg from a baseline of 90 mm Hg.27 In this model, cerebral blood flow was also reduced to a level more than 50% below control values following penetrating injury. These pathophysiologic changes following PBI likely contribute to further neuronal damage and lend the theoretical justification for monitoring of ICP and CPP after PBI.
Carey and colleagues noted that severe respiratory changes occur after projectile injury, even when the missile is of low energy and the tract is distant from the brain stem.26 Carey hypothesized that the location of the medullary respiratory center (directly below the fluid-filled fourth ventricle) may make those neurons more susceptible to the ordinary pressure waves generated by a missile. Above a certain level of energy, a missile often produces an apnea which is fatal unless the animal is supported by mechanical ventilation. The probable correlate in human injuries may be a significant factor responsible for mortality in PBI. Carey’s experiments suggest that only a narrow window exists between the level of energy required for a missile to penetrate the skull and the level that causes fatal apnea.
Initial Assessment and Resuscitation
Advanced Trauma Life Support (ATLS) principles guide initial assessment and resuscitation of the patient with penetrating neurologic injuries.28 Steps include the following:
1. Acute control of including airway, breathing, and circulation
4. A brief initial neurologic evaluation, including the Glasgow Coma Scale (GCS),29 assessment of the pupils, and an evaluation for any focal deficit
5. Assessment of the cranium and face for external injuries
6. Evaluation of the spine for deformities and/or open abnormalities
7. Concomitant head-to-toe evaluation for other life- or limb-threatening injuries
After primary survey for life-threatening injuries, vital signs are monitored frequently and isotonic intravenous fluids are administered as needed. Blood products should be immediately available to replace lost volume. Hypotension without tachycardia warrants consideration of spinal shock. Oxygenation should be monitored with continuous pulse oximetry. Blood is drawn for laboratory tests including arterial blood gas, blood count, electrolytes, coagulation profile, and blood type analysis. Chest x-ray should follow intubation of the trachea. Decompression of the stomach and bladder also allow monitoring of outputs. Most modern trauma centers have replaced routine cervical and pelvis x-rays with whole-body CT scans. Cervical and thoracolumbar spine precautions are maintained in multitrauma patients and those with unclear histories. Radiologic clearance of the cervical spine may not be necessary in patients with isolated penetrating brain injuries.30
Initial Medical Management
Anticonvulsants
Active seizures may lead to acute deterioration, and should be treated aggressively with intravenous diazepam or lorazepam. It is appropriate to administer a loading dose of anticonvulsant medication intravenously to all patients with PBI. These patients suffer higher rates of seizures than those with nonpenetrating traumatic brain injury (TBI).11,31–34 The rate of seizures after PBI has been reported as high as 30% to 50%, with 4% to 10% of seizures occurring within the first week after injury.11,31 Temkin and colleagues have demonstrated in a randomized trial the efficacy of phenytoin in reducing the incidence of seizures in the first week after head injury.34 Their study included patients with penetrating head injury. The use of anticonvulsant prophylaxis beyond 1 week is controversial. Salazar and associates reported a seizure rate of 53% in their study of Vietnam veterans followed over a 15-year period, with 18% of patients having their first seizure more than 5 years after injury.11 Although no studies have demonstrated the efficacy of prophylactic anticonvulsants in preventing late seizures, the high rate of late epilepsy in this population leads many authors to recommend continuing prophylaxis for 1 year or more after injury.35–38 Of interest, Temkin’s most recent meta-analysis of seizure prophylaxis in all traumatic brain injuries suggests that although Carbamazepine or Phenytoin suppress early seizures by as much as 25%, no tested medications have a positive effect on late seizures, even during treatment.39 The most recent opinion about long-term management of these seizures is to manage them the same way one would treat non-traumatic seizures of the same type.40
Antibiotics
Initiation of prophylactic broad-spectrum antibiotic therapy is recommended in penetrating neurologic injuries. The rate of infection is high because of contamination from foreign objects, skin, hair, clothing, and bone fragments, which all may lodge in the wound tract. In the preantibiotic era, rates of infection were nearly 60%, as reported by Whitaker during World War I.41 When penicillin was introduced in a successive fashion to the various theaters of war during World War II, reported rates of infection dropped to 6% to 13%.4 More recent reports from the military and civilian literature, based on routine use of modern broad-spectrum antibiotics, document rates of infection between 1% and 11%, with a trend toward higher rates in the military setting.10,14,42–51 Reports on causative organisms identify Staphylococcus species, Streptococcus, Acinetobacter, Escherichia coli, Klebsiella, and Enterobacter as the common organisms identified in cultures.5,42,44,52 Important risk factors for the development of infection include CSF leak,42,53 air sinus wounds,54 and wound dehiscence.10,44
The issue of which antibiotic regimen is best suited for prophylaxis is not settled. In a survey of American neurosurgeons, Kaufman and colleagues reported the use of a cephalosporin by 87% of respondents, chloramphenicol by 24%, penicillin by 16%, an aminoglycoside by 12%, and vancomycin by 6%.55 The issue of optimal duration of prophylaxis is also unsettled, with very little evidence in the literature to support a recommendation.56 Both the issues of choice of antibiotic and duration of treatment continue to merit a prospective, randomized controlled study.
Initial Imaging
Once the patient is stabilized and the initial neurologic evaluation completed, the patient is taken to the CT suite. Computed tomography is the examination of choice because the extent of brain injury (including intracranial hematomas and air), the extent of bone injury (including in-driven bone chips), and the presence of foreign bodies are all easily identified. The wound tract is readily visualized, and the presence of cerebral edema is noted. The size of the ventricles and cisterns is also noted. In spine injuries, bony detail and injury to surrounding structures is seen optimally. The utility of CT in both the military and civilian settings has been well-documented.12,13,57–59 Head CT findings associated with poor prognosis include bihemispheric injury,50,58,60–64 transventricular path of the projectile,12 intraventricular hemorrhage,61,64 diffuse cerebral edema, and effacement of cisterns Fig. 134-4.65
In rare cases, the patient is hemodynamically unstable because of abdominal or thoracic injuries, and must be taken urgently to the operating theater by the trauma team without CT scan. X-rays of the skull may help to define the projectile tract, the extent of bony injury, and the presence of intracranial air. In such circumstances the neurosurgeon’s clinical judgment and experience are important and dictate further therapy. In some cases a conservative approach is warranted, which may include placement of an ICP monitor until the patient is hemodynamically stable enough to proceed to CT. In the rare case when anisocoria is present, burr holes or an exploratory craniotomy may be undertaken with the aim of evacuating a life-threatening hematoma. Intraoperative ultrasound is useful to localize an intracerebral clot in such cases. In cranial stab wounds, angiography should be considered immediately to look for commonly seen vascular injuries.66 In other types of penetrating trauma, angiography can usually be postponed until after the patient has been stabilized and initial neurosurgical intervention is completed.
Decision Making in the Patient with Poor Prognosis
After stabilization and imaging, a decision concerning further therapy will need to be made. The most difficult clinical scenario exists when the postresuscitation GCS is between 3 and 5. In the civilian setting, between 38% and 81% of patients present in this condition.50,60,61,65,67 Published reports document poor rates of survival in patients with a GCS score of 3 to 5 and even lower rates of neurologically good outcomes.50,60,61,63,65 In the case of a patient who has a postresuscitation GCS score of 3 with dilated and nonreactive pupils without a mass lesion on CT, most neurosurgeons agree that no surgical intervention is warranted.58,68,69 Grahm and colleagues prospectively studied 100 patients with gunshot wounds to the head in a civilian setting.60 They aggressively treated all patients with evidence of neurologic function after resuscitation and found no satisfactory outcome in the group with a postresuscitation GCS score of 3 to 5. They concluded that no further therapy should be offered to a patient with a postresuscitation GCS score of 3 to 5 who did not have evidence of a large, surgically treatable hematoma on CT scan. In a study by Kaufman and colleagues involving two centers, the outcome of 412 patients presenting with a GCS score of 3 to 5 were evaluated. Of these, 4 had a neurologically good outcome.63 In the study by Nagib and colleagues, 1 of 28 patients presenting with a GCS score of 3 to 5 had a favorable outcome.50 Miner and colleagues, in their study of gunshot wounds in a pediatric population, found that decerebrate or decorticate posturing in children did not predict a poor outcome.70 In military series, the results in patients with a GCS score of 3 to 5 are better, with rates of good outcome reaching 15% to 34%.12,14 Thus it is important to keep in mind that, although few, some patients with a GCS score of 4 to 5, or even a GCS score of 3 with evidence of brain stem function, may have neurologically meaningful outcomes. An ethical dilemma arises when considering whether the tremendous societal costs incurred in the aggressive treatment of all patients in this group are justified given the poor outcomes and low rates of survival. No across-the-board recommendation can be made based on the current literature. Though each case will need to be considered individually, most agree that an aggressive approach to treatment is warranted as long as some evidence of motor or brain stem function is demonstrated.71
Surgical Management
The general guidelines of surgical treatment of penetrating injures of the nervous system include:
1. Adequate debridement of devitalized tissue
3. Removal of accessible in-driven bone fragments and foreign bodies
6. Complete closure of the scalp and coverage of the spinal dura
All operative procedures for penetrating head and spine injuries should begin with wide prepping and draping of the injured area to allow for adequate exposure Fig. 134-5. Thorough but gentle irrigation is used to flush out all tissues extruding from the wound site. Two strategies are acceptable in planning the initial scalp incision for head injuries. A traditional large trauma flap may be utilized, especially in cases where CT demonstrates a large hematoma. In cases where the entry site is punctuate, the skin flap may incorporate it. This allows the necrotic edges to be excised, the healthy tissue advanced, and the wound closed primarily. When there is a large laceration at the entry site, this may be extended in lieu of a traditional incision. This is preferable in cases where flap vascularity would otherwise be jeopardized. More extensive scalp injuries may require the use of rotation skin flaps for closure. Consultation with a plastic surgeon is helpful in cases of extensive scalp injuries and should be undertaken prior to the initial incision in order to avoid difficulty with wound closure at the end of operation.
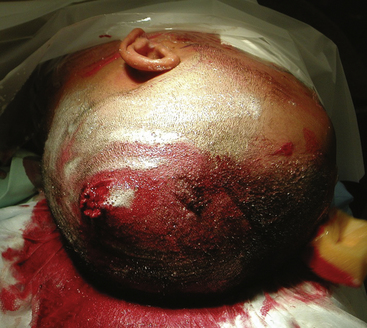
FIGURE 134-5 A large left hemisphere scalp flap is planned for this patient with a perforating frontoparietal gunshot wound.
Adequate exposure of the bony defect is essential. The underlying dural injury often extends beyond the margins of the bone injury. The bone opening should extend beyond the limits of the visible bone injury until intact dura can be visualized. When an underlying hematoma is present, the bone flap should encompass the limits of its extent to allow adequate hemostasis. At the site of penetration, adequate debridement of the bony edges should be performed. Once the dura is exposed, herniating necrotic brain tissue often extrudes from the dural defect. This can be gently irrigated free with saline. The tract of penetration through the brain is gently flushed through the dural defect, removing necrotic tissue as well as any loose foreign objects within the necrotic cavity. At this point the dural defect may need to be extended to allow for adequate debridement of the injured brain. Blood clots are aspirated. A thorough exploration of the resulting cavity is conducted. Accessible bone fragments and foreign bodies are removed. Although CT may demonstrate deeper retained fragments, exploration should not continue beyond the point of safe hemostasis. This is consistent with the approach advocating preservation of viable brain tissue over the removal of all retained fragments.12,13 Meticulous hemostasis is accomplished using bipolar coagulation. Hemostatic agents should be irrigated from the cavity to avoid adding to the mass effect Fig. 134-6.
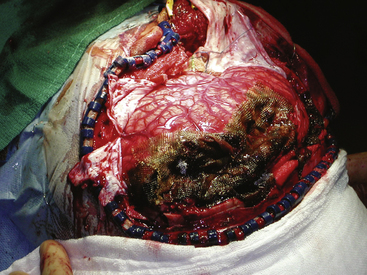
FIGURE 134-6 Intraoperative photo from patient in Fig. 134-5. High hemispheric penetrating injuries are often well tolerated but associated with significant swelling during the acute phase of the injury.
Dural repair is undertaken next. Wartime discussions argue that watertight closure may not be necessary,72–74 but Carey concludes that failure to achieve a watertight closure results in higher complication rates.75 Necrotic dural edges should be removed. Primary closure of the dura is often not possible. Autologous grafts may be utilized in order to obtain a watertight closure. Temporalis fascia or pericranium are local autologous options. If these are not available, a fascia lata graft may be harvested from the thigh or transversalis fascia may be harvested from the abdomen. Allograft dura or cadaveric pericardium may also be used when autologous options are not practical Fig. 134-7.