Chapter 174 Management of Injuries of the Cervical Spine and Spinal Cord
Upper Cervical Spine
Occipitocervical Dislocations
A number of radiographic techniques are used to diagnose AOD. The Powers ratio provides a mathematical relationship between the basion (B), the opisthion (O), the anterior arch of C1 (A), and the posterior arch of C1 (C).1 To apply this ratio, two lines are drawn: one from the basion to the posterior arch of C1 and another from the opisthion to the anterior arch of C1. The ratio of the lengths of these two lines defines Powers ratio, with a value of B×C/O×A >1 raising concern for atlanto-occipital dislocation. This ratio may be unreliable C1 fracture or posterior subluxation cases. The method of Harris et al.2 uses a combination of basion-dental interval to detect longitudinal displacement (normal, less than 12 mm) and basion-axial interval to detect anterior and posterior displacement (normal, 0 to 12 mm). Imaging studies should involve reconstructed computed tomographic scans and MRI studies, with the latter invariably showing ligamentous injury. Because of the extreme instability of occipitocervical dislocations, flexion-extension x-rays are absolutely contraindicated.
A classification scheme developed by Traynelis et al3 involves three types of injury. Type I injuries include anterior displacement of the skull in relation to the cervical spine, type II injuries involve longitudinal displacement, and type III injuries include posterior displacement. The authors suggest that traction with less than 5 lb can be used under radiographic guidance to reduce and stabilize the injury, but such traction should be immediately discontinued following realignment. With type II injuries, traction should never be used, as this may result in the progression of neurological deficits. Despite these recommendations, these injuries are considered highly unstable; thus most clinicians avoid traction but instead provide immediate halo immobilization and/or internal occipitocervical fixation.
Occipital Condyle Fractures
Such fractures have also been classified on the basis of their radiographic appearance. CT reveals that type I injuries involve comminution of the condyle without fragment displacement. Type II injuries are characterized by continuation of a basilar skull fracture into the condyle. Type III injuries involve complete avulsion of the condyle and are presumed to result from excessive loading in rotation or lateral bending.4
According to the classification scheme originally described by Anderson and Montesano,4 the fracture pattern determines treatment. Type II injuries are classified as stable and can be treated with or without a rigid external orthosis for pain control. Types I and III injuries result in ipsilateral alar ligament disruption; thus patients are treated with an external orthosis or a halo vest if the injury is more profound. Surgical intervention is rare but may be indicated when fracture fragments compromise the spinal canal or cause chronic pain as a result of presumed instability.
Atlas (C1)
C1 Burst Fracture (Jefferson Fracture)
C1 burst fractures are presumed to result from direct axial loading that causes lysis of both the anterior and posterior arches, leading to spreading of the lateral masses. Neurological injury is rare, owing to pathological enlargement of the spinal canal. Stability is determined by assessing the extent of injury to the transverse ligament of C1. On open-mouth plain film views, the Rule of Spence states that more than 6.9 mm of combined lateral displacement of the lateral masses over the C2 articular pillars represents an indirect measurement indicating that the transverse ligament has been disrupted.5 Such an injury can be confirmed by noting ligamentous disruption on MRI scans.6 With disruption, the patient may require surgical stabilization if there is purely ligamentous injury; however, some think that if the ligament is intact and the disruption occurs because of a small bony avulsion off C1, then immobilization with a rigid collar or halo vest can be used with the goal of the avulsion fracture’s re-fusing to C1.
Horizontal Fractures of the Anterior Arch
Atlantoaxial Instability
Traumatic atlantoaxial instability is secondary to rupture of the transverse ligament of C1, resulting in an increase in the atlantodental interval (ADI). The ADI is measured from the posterior aspect of the anterior arch of C1 to the anterior aspect of the odontoid process. In adults, an ADI less than 3 mm is considered normal. An ADI between 3 and 5 mm suggests transverse ligament disruption. An ADI greater than 5 mm suggests disruption of both the transverse and accessory ligaments.7 Dickman et al6 described two types of isolated transverse atlantal ligament injuries identified on MRI studies. Type I involves ligament injury without associated fracture of the atlas, and type II involves an avulsion fracture of the atlas at the insertion of the transverse atlantal ligament. The authors concluded that patients with type I injuries should be treated with early surgical fixation because of the inherent instability at C1-C2 following ligamentous disruption, whereas type II injuries require rigid external immobilization.
With such ligamentous injury, surgery is indicated to regain stability, and there exist numerous techniques to stabilize the C1-C2 complex. The original techniques of Gallie,8 Brooks and Jenkins,9 and Dickman et al10 involve placing a structural bone graft between the C1 arch and C2 spinous process and supplementing it by posterior wiring around C1 and C2. Although they are rigid in flexion-extension, these constructs are less stable in rotation and require halo immobilization. More recently, techniques involving screw fixation have been developed that provide added stability in all loading modes. C1-C2 transarticular screws directly fix the C1-C2 joints bilaterally. They were originally used in conjunction with posterior wiring techniques. Unfortunately, such screws can be challenging to place, as the course of the vertebral artery may be prohibitive in relation to the screw trajectory. More recently, posterior screw-rod constructs based on the method of Harms and colleagues11 have gained popularity In these constructs, C1 lateral mass screws are attached to screws in the C2 pedicle, the pars interarticularis, or the laminae.
Odontoid Fracture
Odontoid fractures are relatively common cervical fractures and have been classified into three types by Anderson and D’Alonzo.12 Type 1 fractures occur at the tip of the dens and are considered avulsion fractures of the alar ligaments. Although relatively rare, these injuries are considered stable and heal well with a rigid collar. Type 2 fractures occur through the odontoid process and are generally considered unstable injuries. Type 3 fractures occur in the cancellous bone of the C2 body. These are more stable than type 2 fractures and usually heal with 12 weeks of immobilization.
Operatively, anterior screw fixation leads to a higher rate of fusion than the use of an external orthosis (Figure 174-1). However, three anatomical characteristics may affect the technical success of screw placement. First, the patient cannot be significantly kyphotic in the cervical or cervicothoracic spine, as this will prevent the surgeon from obtaining the correct trajectory to place the screw. Second, the fracture fragment must be straight or angled posteriorly so that while the surgeon is reducing the fragment to the C2 body using a lag screw, the fracture planes can be opposed. Third, a comminuted fracture or a poorly angled fracture may not be satisfactorily realigned with the anterior screw and thus may never heal. In these cases, a posterior fusion may be appropriate, using a C1-C2 construct to stabilize the fractured axis.
Posterior Element and Hangman’s Fracture
A Hangman’s fracture is the result of a traumatic spondylolisthesis of C2 and can be considered a bilateral pars or pedicle fracture (Figure 174-2). This injury was initially attributed to the pathology sustained during judicial hangings, but motor vehicle accidents and falls currently account for the majority of injuries. Effendi et al.13 classified these injuries on the basis of the degree of displacement and angulation of C2 relative to C3. Type 1 fractures are not displaced and have no angulation. Type 2 injuries involve more than 3-mm translation, and a subtype, type 2A, involves significant angulation but minimal translation. Type 3 injuries involve severe angulation and translation and may involve unilateral or bilateral facet dislocation. Although surgery is indicated in cases of severe angulation, which is associated with C2-C3 disc or posterior articular disruption, types 1 and 2 may be treated effectively with external fixation (halo or rigid cervical collar).
Subaxial Spine
In adults, injuries to the subaxial cervical spine occur most frequently at C5 and below because the instantaneous moment of internal rotation between the head and the rigid thoracic spine lies in this area. Children have proportionally more massive heads and thus the moment is more cephalad, leading to a higher rate of high cervical injuries in children. Recently, Vaccaro et al.14 proposed a subaxial cervical spine injury classification system that shows early signs of validity and reliability. In their classification system, they evaluated three main parameters of injury, including morphology, disruption of the disco-ligamentous complex, and neurological status. The overall injury score is obtained by summing the scores from each category (Table 174-1). A higher score corresponds to an increased likelihood of the need for surgical intervention. The following sections describe the classic injury patterns of the subaxial cervical spine.
Table 174-1 Parameters of the Subaxial Cervical Spine Injury Classification System
Parameters | Points |
---|---|
Morphology | |
No abnormality | 0 |
Compression | 1 |
Burst | +1 = 2 |
Distraction (facet perch, hyperextension) | 3 |
Rotation and/or translation (facet dislocation, unstable teardrop or advanced-stage flexion compression injury) | 4 |
Disco-ligamentous complex | |
Intact | 0 |
Indeterminate (isolated interspinous widening, MRI signal change only) | 1 |
Disrupted (widening of disc space, facet perch or dislocation) | 2 |
Neurological status | |
Intact | 0 |
Root injury | 1 |
Complete cord injury | 2 |
Incomplete cord injury | 3 |
Continuous cord compression in setting of neurological deficit | +1 |
From Vaccaro et al.14
Unilateral Facet Dislocation
Unilateral facet dislocation occurs with flexion-rotation injury and involves the inferior articular facet of the upper vertebrae’s dislocating anterior to the superior articular process of the lower vertebrae, resulting in “locked” facets. In some cases, the inferior articular process sits directly superior to the inferior articulating process in a “perched” fashion. Radiographically, usually a less than 25% anterolisthesis of the rostral vertebral body is seen on lateral plain films, rotation of the spinous processes on anteroposterior films to one side, and the dislocation of facets noted on oblique x-rays. CT is an ideal imaging modality for determining the extent of facet fracture (Figure 174-3).
There is a great deal of debate on the utility of prereduction MRI in patients who will undergo traction. Reports in the literature include cases of patients’ conditions deteriorating following traction as a result of a previously undiagnosed herniated disc at the injured level.15 Thus it has been suggested that patients be screened by MRI prior to reduction. However, most clinicians suggest initiating traction as soon as possible in patients with a neurological deficit who can participate by following commands during the reduction process. For patients who are unable to participate because of altered mental status (e.g., head injury), an MRI study should be obtained. It should also be noted that it is often harder to reduce unilaterally locked facets than bilaterally locked facets, and a rotational component may be added during the reduction process to facilitate realignment.
Bilateral Facet Dislocation
Hyperflexion can cause severe posterior ligamentous injury, resulting in bilaterally locked facets. When the bony facets remain intact, this injury can result in severe anterolisthesis and canal compromise and thus is most commonly associated with a neurological deficit (Figure 174-4). On plain films, 50% or greater subluxation of the vertebral body may be seen, and angular deformity is common. As with unilateral facet dislocation, it is advisable to attempt traction reduction to realign the spinal column prior to attempting fixation. The same concerns apply with regard to an underlying herniated disc. Because of the severity of destabilization and commonly associated neurological dysfunction, combined anterior-posterior fixation should be considered in these patients.
Hyperextension Injury
Hyperextension injuries can take the form of dislocation, fracture-dislocation, or laminar injuries. Radiographically, spinal alignment may appear normal and bony anatomy may be intact, but severe injury to the disc and posterior ligamentous complex may be present. Prevertebral soft-tissue injures, widening of the intervertebral spaces, and irregularity or horizontal fractures of the vertebral end plates may be seen. Because of extensive disco-ligamentous disruption, such injuries are considered highly unstable. Central cord syndrome may be a common finding, especially in association with cervical spondylosis. Similarly to a nonbony Chance fracture16 in the thoracolumbar region, it is unlikely that a dislocation through the disc space will heal and fusion will be required, which may be achieved by both anterior and posterior fixation.
Clay-Shoveler’s Fracture
The classic clay-shoveler’s fracture was mechanistically described as an avulsion of the spinous process.17 Such an injury occurs while shoveling clay, which sticks to the shovel and causes the trapezius to pull strongly on the spinous process when a the shovel is thrust forward. This injury most commonly occurs at C7 and less frequently at C6 and T1 (Figure 174-5). At present, this injury typically occurs as a result of a direct blow to the spinous processes. This is considered a stable fracture and can be treated by placing the patient in an external orthosis for pain control.
Spinal Cord Injury
As stated above, there are a number of injury patterns that lead to severe spinal column instability and thus have the potential for inducing damage to the cord. In evaluating spinal cord injury, the first step is to define whether the injury is complete or incomplete. Classically, a complete spinal cord injury was thought to be a lack of conscious sensation and voluntary muscle control below the site of the injury. However, such a description is misleading because partial preservation, including lateral preservation, of function below the injury site is rather common. In addition, a delayed return of function may occur in the first few days after the injury, even after an initial determination that the injury is complete. Therefore, in the case of a complete motor injury, sensation and bladder and/or bowel dysfunction must be assessed. In 1992, the American Spinal Injury Association (ASIA)18 defined a complete spinal cord injury as one that yields no sensory or motor function in the perineal and anal regions (S4-S5). A scale has also been created that calculates a score based on motor and sensory evaluation of the entire body (Figure 174-6).19 Motor evaluation may yield a maximum of 50 points for the upper extremities and 50 points for the lower extremities. Sensation can yield a total of 112 points for both pinprick and light touch sensation. Following this evaluation, the ASIA Impairment Scale classifies the patient’s condition into one of five categories (A through E), in which A is a complete injury, B through D are incomplete, and E is normal sensory and motor function (Table 174-2).
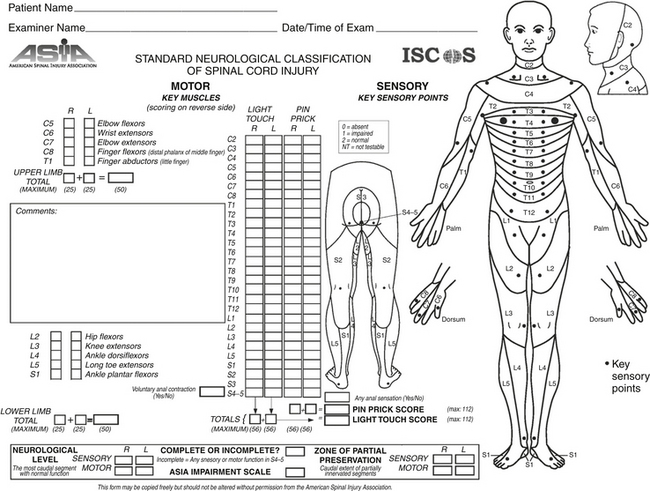
FIGURE 174-6 American Spinal Injury Association (ASIA) evaluation form. Available at http://www.asia-spinalinjury.org/publications/59544_sc_Exam_Sheet_r4.pdf.
Table 174-2 American Spinal Injury Association (ASIA) Impairment Scale
Grade | Classification | Description |
---|---|---|
A | Complete | No motor or sensory function is preserved in the sacral segments S4-S5. |
B | Incomplete | Sensory but not motor function is preserved below the neurological level and includes the sacral segments S4-S5. |
C | Incomplete | Motor function is preserved below the neurological level, and more than half of the key muscles below the neurological level have a muscle grade <3. |
D | Incomplete | Motor function is preserved below the neurological level, and more than half of the key muscles below the neurological level have a muscle grade of ≥3. |
E | Normal | Motor and sensory function are normal. |
Incomplete Spinal Cord Injury
As shown previously in describing the Vaccaro et al.14 classification system, incomplete injuries are weighted more heavily than complete injuries or normal neurological function with regard to the need for surgical intervention. This is mainly because open or closed reduction and cord decompression can allow the patient to maintain or regain neurological function if done expediently. The following sections describe some of the classic incomplete spinal cord syndromes.
Central Cord Syndrome
Central cord syndrome is the most common spinal cord injury and classically occurs following acute hyperextension in an elderly patient with preexisting spondylosis and acquired cervical stenosis. It also can occur in motor vehicle accidents or sports-related injuries following a violent blow to the forehead or face. The centermost region of the spinal cord is a vascular watershed zone, which renders it more susceptible to injury resulting from edema. As a result, the clinical syndrome is similar to that seen in patients with syringomyelia. Specifically, patients exhibit motor weakness that is greater in the upper extremities than in the lower extremities, varying degrees of sensory abnormalities, and myelopathic findings such as sphincter dysfunction and urinary retention. The gold standard for imaging spinal cord pathology is MRI, and in these cases T2-weighted images can show acute spinal cord edema in the setting of spinal stenosis (Figure 174-7). Clinically, there is often an initial phase of improvement (legs first, then bladder, then upper extremities) that is followed by a plateau phase and late deterioration. Ninety percent of patients are able to walk with assistance within 5 days.20 Surgical treatment involves spinal cord decompression if compression is persistent in patients with severe stenosis or in those with associated injuries. Controversy surrounds early versus late decompression, but there is no clear consensus in the literature regarding the timing of surgery.
Anterior Cord Syndrome
Also know as “anterior spinal artery syndrome,” cord infarction occurs in the territory supplied by the anterior spinal artery and usually results from a dislocated bone fragment or a traumatic herniated disc. Patients present with paraplegia or quadriplegia, loss of pain, and temperature sensation below the lesion, but maintain posterior column function throughout (two-point discrimination, position sense, and deep pressure sensation). It is vital to differentiate a nonsurgical condition (i.e., anterior spinal artery thrombosis and occlusion) from a surgical one (pressure from an anterior bone fragment or disc herniation). This is achieved by a combination of CT, MRI, and angiography. Unfortunately, only 10% to 20% of patients recover functional motor control.
Brown-Sequard Syndrome
Brown-Séquard syndrome usually follows spinal cord hemisection as a result of penetrating trauma, but can also occur with large disc herniation or spinal epidural hematoma. The classic description involves a dissociated sensory loss with contralateral loss of pain and temperature but preserved ipsilateral light touch and posterior column function. In addition, there is ipsilateral motor paralysis below the level of the lesion. This syndrome has the most favorable prognosis among the incomplete spinal cord syndromes, as up to 90% of patients may regain the ability to ambulate independently as well as some degree of anal and urinary sphincter control.21
Anderson L.D., D’Alonzo R.T. Fractures of the odontoid process of the axis. 1974. J Bone Joint Surg Am.. 2004;86-A:2081.
Anderson P.A., Montesano P.X. Morphology and treatment of occipital condyle fractures. Spine (Phila Pa 1976). 1988;13:731-736.
Brooks A.L., Jenkins E.B. Atlanto-axial arthrodesis by the wedge compression method. J Bone Joint Surg Am.. 1978;60:279-284.
Chen T.Y., Lee S.T., Lui T.N., et al. Efficacy of surgical treatment in traumatic central cord syndrome. Surg Neurol. 1997;48:5435-5441.
Dickman C.A., Greene K.A., Sonntag V.K. Injuries involving the transverse atlantal ligament: classification and treatment guidelines based upon experience with 39 injuries. Neurosurgery. 1996;38:44-50.
Dickman C.A., Sonntag V.K., Papadopoulos S.M., Hadley M.N. The interspinous method of posterior atlantoaxial arthrodesis. J Neurosurg. 1991;74:190-198.
Doran S.E., Papadopoulos S.M., Ducker T.B., Lillehei K.O. Magnetic resonance imaging documentation of coexistent traumatic locked facets of the cervical spine and disc herniation. J Neurosurg. 1993;79:341-345.
Effendi B., Roy D., Cornish B., et al. Fractures of the ring of the axis: a classification based on the analysis of 131 cases. J Bone Joint Surg Br. 1981;63:319-327.
Harris J.H.Jr., Carson G.C., Wagner L.K., Kerr N. Radiological diagnosis of traumatic occipitovertebral dissociation. II. Comparison of three methods of detecting occipitovertebral relationships on lateral radiographs of supine subjects. AJR Am J Roentgenol. 1994;162:887-892.
Hinck V.C., Hopkins C.E. Measurement of the atlanto-dental interval in the adult. Am J Roentgenol Radium Ther Nucl Med. 1960;84:945-951.
Powers B., Miller M.D., Kramer R.S., et al. Traumatic anterior atlanto-occipital dislocation. Neurosurgery. 1979;4:12-17.
Roth E.J., Park T., Pang T., et al. Traumatic cervical Brown-Sequard and Brown-Sequard-plus syndromes: the spectrum of presentations and outcomes. Paraplegia. 1991;29:582-589.
Spence K.F.Jr., Decker S., Sell K.W. Bursting atlantal fracture associated with rupture of the transverse ligament. J Bone Joint Surg Am.. 1970;52:543-549.
Traynelis V.C., Marano G.D., Dunker R.O., Kaufman H.H. Traumatic atlanto-occipital dislocation: case report. J Neurosurg. 1986;65:863-870. A published erratum appears in J Neurosurg. 1987;66:789
Vaccaro A.R., Hulbert R.J., Patel A.A., et alSpine Trauma Study Group. The Subaxial Cervical Spine Injury Classification system: a novel approach to recognize the importance of morphology, neurology, and integrity of the disco-ligamentous complex. Spine (Phila Pa 1976). 2007;32:2365-2374.
1. Powers B., Miller M.D., Kramer R.S., et al. Traumatic anterior atlanto-occipital dislocation. Neurosurgery. 1979;4:12-17.
2. Harris J.H.Jr., Carson G.C., Wagner L.K., Kerr N. Radiological diagnosis of traumatic occipitovertebral dissociation: 2. Comparison of three methods of detecting occipitovertebral relationships on lateral radiographs of supine subjects. AJR Am J Roentgenol. 1994;162:887-892.
3. Traynelis V.C., Marano G.D., Dunker R.O., Kaufman H.H. Traumatic atlanto-occipital dislocation: case report. J Neurosurg. 1986;65:863-870. A published erratum appears in J Neurosurg. 1987;66:789
4. Anderson P.A., Montesano P.X. Morphology and treatment of occipital condyle fractures. Spine (Phila Pa 1976). 1988;13:731-736.
5. Spence K.F.Jr., Decker S., Sell K.W. Bursting atlantal fracture associated with rupture of the transverse ligament. J Bone Joint Surg Am.. 1970;52:543-549.
6. Dickman C.A., Greene K.A., Sonntag V.K. Injuries involving the transverse atlantal ligament: classification and treatment guidelines based upon experience with 39 injuries. Neurosurgery. 1996;38:44-50.
7. Hinck V.C., Hopkins C.E. Measurement of the atlanto-dental interval in the adult. Am J Roentgenol Radium Ther Nucl Med. 1960;84:945-951.
8. Gallie W.E. Fractures and dislocations of the cervical spine. Am J Surg. 1939;46:495-499.
9. Brooks A.L., Jenkins E.B. Atlanto-axial arthrodesis by the wedge compression method. J Bone Joint Surg Am.. 1978;60:279-284.
10. Dickman C.A., Sonntag V.K., Papadopoulos S.M., Hadley M.N. The interspinous method of posterior atlantoaxial arthrodesis. J Neurosurg. 1991;74:190-198.
11. Puttlitz C.M., Melcher R.P., Kleinstück F.S., et al. Stability analysis of craniovertebral junction fixation techniques. J Bone Joint Surg Am. 2004;86-A:561-568.
12. Anderson L.D., D’Alonzo R.T. Fractures of the odontoid process of the axis. 1974. J Bone Joint Surg Am.. 2004;86-A:2081.
13. Effendi B., Roy D., Cornish B., et al. Fractures of the ring of the axis: a classification based on the analysis of 131 cases. J Bone Joint Surg Br. 1981;63-B:319-327.
14. Vaccaro A.R., Hulbert R.J., Patel A.A., et al, et al. Spine Trauma Study Group. The Subaxial Cervical Spine Injury Classification system: a novel approach to recognize the importance of morphology, neurology, and integrity of the disco-ligamentous complex. Spine (Phila Pa 1976). 2007;32:2365-2374.
15. Chance G.Q. Note on a flexion fracture of the spine. Br J Radiol. 1948;21:452-453.
16. Helms C.A. Malignant bone and soft tissue tumors. In: Brant W.E., Helms C.A. Fundamentals of Diagnostic Radiology. 3rd ed. Philadelphia, PA: Lippincott Williams & Wilkins; 2007:1107.
17. Doran S.E., Papadopoulos S.M., Ducker T.B., Lillehei K.O. Magnetic resonance imaging documentation of coexistent traumatic locked facets of the cervical spine and disc herniation. J Neurosurg. 1993;79:341-345.
18. ASIA/IMSOP. International Standards for Neurological and Functional Classification of Spinal Cord Injury. Chicago, IL: American Spinal Injury Association; Revised 1992.
19. Linassi G., Li Pi Shan R., Marino R.J. A web-based computer program to determine the ASIA impairment classification. Spinal Cord. 2010;48:100-104.
20. Chen T.Y., Lee S.T., Lui T.N., et al. Efficacy of surgical treatment in traumatic central cord syndrome. Surg Neurol. 1997;48:5435-5441.
21. Roth E.J., Park T., Pang T., et al. Traumatic cervical Brown-Sequard and Brown-Sequard-plus syndromes: the spectrum of presentations and outcomes. Paraplegia. 1991;29:582-589.