5 Malignant Gliomas in Adults
Introduction
Malignant gliomas (MG) account for more than 75% of the approximately 20,500 newly diagnosed malignant primary brain tumors in the United States each year.1 They represent the most common type of malignant primary brain tumor in adults. More than half of MGs are glioblastomas (GBM), the most aggressive subtype. The majority of the remainder include anaplastic astrocytomas (AA), anaplastic oligodendrogliomas (AO), and anaplastic oligoastrocytomas (AOA).1,2 Other MG subtypes are rare. Glioblastoma is a disease of middle age, with a median age at diagnosis of 64 years. Anaplastic gliomas (AG) affect a younger adult population with a median age of 45 years.1,3 Despite being relatively uncommon, MGs are incurable and are responsible for a disproportionate share of cancer-related morbidity and mortality.4 With optimal treatment, median survival is only 12 to 15 months for GBM and 2 to 5 years for AG. Established adverse prognostic factors in MG include increasing age, GBM histology, poor performance status, and unresectable tumor.5 Recently, there have been important advances in the molecular pathogenesis of MG, some of which may provide additional prognostic information that will help in therapeutic decision-making.6–12 This chapter summarizes the pathology, pathogenesis, diagnosis, and management of adult MGs with a focus on therapy and recent therapeutic advances. This topic has been recently reviewed elsewhere.4,13
Epidemiology
The only established risk factors for MG are exposure to ionizing radiation and rare familial syndromes such as neurofibromatosis types 1 and 2, Li-Fraumeni syndrome, and Turcot syndrome.3,14 Approximately 5% of patients with malignant gliomas have a family history of gliomas. The genetic basis for most of these tumors is unknown. An international consortium, GLIOGENE, has been established to study the genetic basis of familial gliomas.15 Exposures to various carcinogens, infections such as cytomegalovirus, diet, elevated IgE, electromagnetic radiation, and cellular phones have not been conclusively linked to MG risk.3 Malignant glioma epidemiology is reviewed in detail elsewhere in this volume.
Pathology
MGs are infiltrative tumors with a presumptive glial cell of origin. The World Health Organization (WHO) classification scheme defines four histologic grades for astrocytomas. These include grade I (pilocytic astrocytoma), grade II (diffuse astrocytoma), grade III (AA), and grade IV (GBM).2 Grade I tumors occur primarily in children. Grade II tumors are considered low-grade gliomas, while grade III and IV tumors are MGs. Typical histologic features of AAs include hypercellularity, nuclear atypia, and mitoses. The presence of microvascular proliferation and/or necrosis often indicates progression to GBM. Oligodendrogliomas are classified as well-differentiated oligodendrogliomas (grade II) or AOs (grade III). Mixed tumors, or oligoastrocytomas, are also classified as well-differentiated (grade II) or AOA (grade III). Tumors with oligodendroglial components may have distinctive features such as perinuclear clearing, giving rise to a “fried-egg” appearance, and a reticular pattern of blood vessel growth.
Molecular Pathogenesis
Recent studies have begun to elucidate the molecular pathogenesis of MGs.10–12,16 Such information may help to improve tumor classification and prognostication.8,9,17–19 As has been observed for many cancers, increasing malignancy in glial tumors is associated with an accumulation of genetic abnormalities that results in impairment of cell cycle control, DNA repair, and signaling.12,20 On the basis of differences in molecular pathogenesis and natural history, GBMs are often classified as primary or secondary tumors (Figure 5-1).12,20 Primary GBMs arise de novo in older patients, and have a characteristic molecular genetic profile that includes epidermal growth factor receptor (EGFR) amplification and mutations, loss of heterozygosity (LOH) of chromosome 10 q, phosphatase and tensin homolog deleted on chromosome 10 (PTEN) mutations, and p16 deletion. Secondary GBMs are much rarer, evolve from lower-grade gliomas, and typically occur in younger patients. Typical genetic abnormalities include TP53 mutations,21 platelet-derived growth factor (PDGFR) overexpression, abnormalities in the p16/retinoblastoma (Rb) pathway, and LOH 10 q.12,22 These tumors may also have mutations of the iso- citrate dehydrogenase gene.23 None of these features allow for definitive GBM classification. Primary and secondary GBMs cannot be distinguished histologically and have not been shown to respond differentially to treatment.
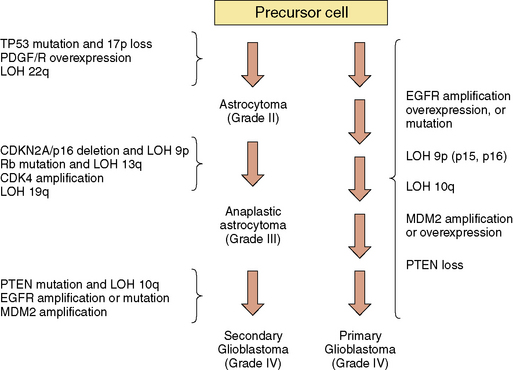
Figure 5-1 Molecular genetic changes associated with glioma progression.
Abbreviations: CDK, cyclin-dependent kinase; EGFR, epidermal growth factor receptor; LOH, loss of heterozygosity; MDM2, murine double minute 2; PDGF/R, platelet-derived growth factor/receptor; PTEN, phosphate and tensin homolog deleted on chromosome 10; Rb, retinoblastoma.
(Adapted from Wen PY, Kesari S, Drappatz J. Expert Rev Anticancer Ther 2006;6:733–54.)
Abnormalities in EGFR signaling are common in MGs.12 EGFR amplification and overexpression are observed in about 40% of primary GBMs. EGFRvIII is a constitutively active EGFR mutant that is expressed by approximately 30% of GBMs, and represents a potentially important therapeutic target because of its exclusive expression by tumor cells.12,18 Activating mutations in the extracellular domain of the EGFR are also present in a subset of GBMs.24 Activation of the PDGFR promotes tumor growth as well, and MGs frequently coexpress PDGF and PDGFR.12 Signaling through these and other growth factor receptors activates fundamental signal transduction pathways such as the Ras/mitogen-activated protein kinase (MAP-kinase) pathway and the phosphoinositide 3′-kinase (PI3K)/Akt/mammalian target of rapamycin (mTOR) pathway, both of which promote cell proliferation.12 PTEN is an endogenous inhibitor of PI3K signaling that is mutated or lost in up to 50% of GBMs.12,25 Downstream targets of these growth factor signaling pathways activate transcriptional programs for cell survival, proliferation, and invasion. Additionally, many of these pathways serve to upregulate vascular endothelial growth factor (VEGF) and angiogenesis, which has an emerging pathogenic role in MGs that is discussed in greater detail below.26,27
For cancer in general and MG specifically, there is growing interest in “personalized medicine,” which refers to the effort to individualize therapy based on a particular tumor’s molecular genetic profile.7,9 Examples of molecular predictors with probable therapeutic value are chromosomes 1 p/19 q, the loss of which may confer chemosensitivity in oligodendroglial tumors,28–30 and MGMT promoter methylation, which may predict temozolomide sensitivity in GBM.8,31 Advances in gene sequencing and expression profiling are helping to define molecular subtypes of MG that will allow for optimal selection of targeted therapeutics for individual patients.32,33
Cellular Origins
Despite recent advances, the cell of origin for MGs remains unknown. Emerging evidence suggests that neural stem cells, or related progenitor cells, may undergo malignant transformation and give rise to these tumors.16,34–38 Additionally, glioma stem cells contribute to the treatment resistance of MGs38,39; a recent study found that expression of stem cell markers such as CD133 in MG specimens may predict resistance to both radiation and chemotherapy.40 The radioresistance of stem cells is mediated by robust activation of DNA damage response pathways.41 Mechanisms that contribute to stem cell chemoresistance are different and include increased production of O6-methylguanine-DNA-methyltransferase (MGMT), overexpression of multi-drug resistance genes, and apoptosis inhibition.42–44
Diagnosis
Like other space-occupying lesions, MGs often present with a combination of general- ized and focal symptoms. Generalized symptoms include headaches, mood changes, altered mental status, and psychomotor slowing. Focal symptoms reflect the location of the lesion in the brain and may include seizures, hemiparesis or hemisensory loss, and aphasia. Among the generalized symptoms, headaches are common and are frequently indistinguishable from tension headaches.45 The classical pattern of headaches that are worse in the morning and with recumbency is only occasionally reported. In the face of suspicious neurological symptoms, the possibility of MG is often suggested by findings on contrast-enhanced magnetic resonance imaging (MRI) or computerized tomography (CT). A typical appearance of MG on either MRI or CT is an irregularly enhancing mass with associated edema and mass effect. Although GBMs often have a central necrotic cavity and more peritumoral edema than AGs,46 pathological confirmation of the diagnosis is crucial, as radiographic features are currently inadequate to reliably determine histology and tumor grade.
Medical Management
Common medical and neurological problems in MG patients include seizures, peritumoral edema, venous thromboembolic disease, fatigue, mood disorders, and cognitive dysfunction.47 Patients who experience seizures require treatment with antiepileptic drugs (AED). Many of the older AEDs (e.g., phenytoin, carbamazepine, and phenobarbital) are inducers of hepatic cytochrome P450, the same enzyme system that metabolizes many chemotherapeutic agents. For this reason, newer AEDs that neither induce nor inhibit the cytochrome P450 system (e.g., gabapentin, levetiracetam, and lamotrigine) are recommended. Although the American Academy of Neurology suggests that AEDs not be routinely prescribed to brain tumor patients who have not had seizures,48 many practitioners continue to administer AED prophylaxis.49
Because many MG patients experience symptomatic peritumoral edema, corticosteroids such as dexamethasone are often required.50 Adverse effects such as Cushing syndrome and steroid myopathy frequently develop due to prolonged corticosteroid administration. This treatment also increases the risk of Pneumocystis jirovecii pneumonia. Prophylactic antibiotics are generally prescribed for these patients,47 although the value of this practice is unproven.51 Late complications of corticosteroid use, such as osteoporosis with vertebral body compression fractures, are increasingly observed as new treatments prolong survival. Routine prophylaxis with calcium and vitamin D supplementation and bisphosphonates is an important consideration. Emerging antiangiogenic therapies such as bevacizumab (a humanized monoclonal antibody against VEGF) and VEGFR inhibitors effectively treat peritumoral edema and may permit reduction in corticosteroid doses.47,50,52
Venous thromboembolism from leg and pelvic veins is an important risk for MG patients, with a lifetime incidence of 20% to 30%.47,53 Anticoagulation in MG patients with venous thromboembolism confers a low risk of intratumoral hemorrhage.47,54 Therefore, in most circumstances, anticoagulation is appropriate treatment for venous thromboembolism in a MG patient. When a MG patient has recently had brain surgery or has intracerebral hemorrhage, inferior vena cava filters are a reasonable alternative, although they have high complication rates.55 Results from a large randomized clinical trial performed in cancer patients with venous thromboembolism indicate that treatment with low molecular weight heparin confers a lower risk of recurrent thromboembolic disease than does treatment with warfarin (9% versus 17%), without a difference in bleeding rates or mortality.56 On the basis of these data, which included 27 brain tumor patients, low molecular weight heparin is recommended by many physicians for treatment of venous thromboembolism in MG patients.
Fatigue is a common complaint among MG patients, and may respond to treatment with modafinil or methylphenidate.57 Methylphenidate is also used to treat apathy or abulia. Limited evidence supports the use of donepezil58 and memantine for memory impairment. Depression and anxiety respond to standard psycho-pharmacological interventions59 and are likely underdiagnosed in this population.
Tumor-Directed Therapy (See Table 5-1)
SURGERY
Standard therapy for newly diagnosed MGs begins with a surgical procedure, as a definitive diagnosis cannot be made based on radiographic features alone. Although the infiltrative nature of gliomas precludes surgical cure, maximal surgical resection is recommended in all newly diagnosed patients. Benefits of surgical resection include improvement of symptoms related to mass effect, reduction of tumor volume remaining to be treated with other modalities,60 and removal of the necrotic tumor core which may be poorly accessible to circulating chemotherapy and resistant to radiation therapy (RT). Surgical resection also increases diagnostic accuracy, as biopsy provides a small specimen for pathological review that may not be representative of the entire lesion.61 Mounting evidence of variable quality suggests that surgical resection confers a modest survival benefit as compared to biopsy.62,63 Advances including MRI-guided neuro-navigation, intraoperative MRI, functional MRI, intraoperative mapping,64 and fluorescent-guided surgery65 have reduced surgical complication rates and allowed more complete tumor resections.66 Patients with inoperable tumors located in eloquent areas require stereotactic biopsies for tissue diagnosis.
TABLE 5-1 Summary of Therapeutic Options for Malignant Gliomas
Setting | Histology | Treatment Options |
---|---|---|
Newly Diagnosed Tumor* | Glioblastoma |
Astrocytoma**
Abbreviations: PCV, procarbazine, lomustine (CCNU), and vincristine; RT, radiation therapy; SRS, stereotactic radiosurgery; TMZ, temozolomide.
* Treatment should always begin with maximal surgical resection.
** No standard of care has been defined.
*** Clinical trial enrollment should be offered to recurrent malignant glioma patients whenever possible.
Adapted from: Wen PY, Kesari S. Malignant gliomas in adults. N Engl J Med 2008;359:492–507.
Surgery may also be considered in recurrent MG patients with good performance status when the tumor is accessible, symptomatic, and distant from eloquent areas. Surgical resection in the recurrent setting may improve quality of life and allow time for additional therapy.67 The impact of resection on survival in recurrent MG patients is uncertain.
RADIATION THERAPY
Radiation therapy is generally considered to be the most important treatment modality for MGs. The addition of RT to surgery provides a robust survival benefit for GBM patients, increasing median survival from 3 to 4 months to 7 to 12 months.68,69 Standard RT for MG is involved-field external beam irradiation to a total dose of 60 Gy, delivered in 1.8 Gy to 2.0 Gy fractions over approximately 6 weeks. The definition of the involved field varies, but it generally includes the area of T2 hyperintensity on MRI plus a 1 cm to 2 cm margin, often with a boost to the area of contrast enhancement. Conformal planning is used to reduce radiation exposure to surrounding brain. Although MG is an infiltrative disease, 90% of conventionally irradiated tumors recur locally at the original site.70
Many variations on standard RT have been investigated in an attempt to increase efficacy. Randomized studies with higher total radiation doses have confirmed the early impression that doses greater than 60 Gy yield no additional benefit.71 A number of studies using altered fractionation schemes have failed to show any benefit over conventional fractionation.72 Various radiosensitizing agents have been administered to patients with MGs, though none has demonstrated substantial efficacy. Strategies designed to provide additional radiation dose to the resection cavity, such as brachytherapy73 and stereotactic radiosurgery (SRS),74,75 have neither improved local control nor survival. Newer approaches including chemotherapy,13 targeted molecular agents,76 and antiangiogenic agents77 may potentially work synergistically with RT and improve outcomes and are under investigation.
Patients over the age of 70 represent a challenging subgroup of MG patients with an extremely poor prognosis. In this population, the addition of RT to supportive care alone increases median survival from 16.9 weeks to only 29.1 weeks.78 Furthermore, older patients are at increased risk for both acute and delayed radiation toxicity. Common manifestations include disabling fatigue that may last weeks to months,79 nausea, and headache. Treatment options include an abbreviated course of RT (40 Gy in 15 fractions over 3 weeks)80 or chemotherapy alone81; in elderly patients, both approaches produce similar outcomes to conventional RT regimens.
Additional involved-field RT is rarely offered to patients with recurrent MG, as doses higher than 60 Gy offer marginal benefit and increase the risk of radiation necrosis.82 Small nonrandomized studies have demonstrated a survival benefit for MG patients treated with SRS at recurrence.75 However, much of the data is subject to selection bias, and this approach is not routinely utilized. Fractionated stereo-tactic RT has also been evaluated for treatment of recurrent MG.83 Fractionation allows for increased radiation doses to the tumor bed with less risk of injury to surrounding brain, but its efficacy is also unproven.
CHEMOTHERAPY
The importance of chemotherapy in MG treatment has grown in recent years. Early studies of adjuvant nitrosourea therapy for MGs were methodologically flawed and failed to demonstrate a significant survival advantage.68,84 Subsequent meta-analyses confirmed that adjuvant chemotherapy produces a modest survival benefit (6% to 10% increase in 1-year survival).85,86 In 2005, the European Organization for Research and Treatment of Cancer (EORTC) and the National Cancer Institute of Canada (NCIC) reported the results of a large randomized phase III trial in newly diagnosed GBM, comparing RT alone (60 Gy over 6 weeks) with RT and concomitant daily temozolomide (Temodar®, Schering-Plough, Kenilworth, New Jersey; 75 mg/m2/d), followed by adjuvant temozolomide therapy (150 to 200 mg/m2/day for 5 consecutive days out of every 28-day cycle, for 6 cycles).69 Grade 3 or 4 toxicity in the chemotherapy group was modest and included fatigue (13%), nausea (2%), thrombocytopenia (12%), and neutropenia (7%). The addition of temozolomide to RT increased median survival compared to RT alone (14.6 mo vs. 12.1 mo; p< 0.0001). At two years, the proportion of surviving patients in the chemotherapy group was 26.5% as compared to 10.4% in the RT alone group. On the basis of these results, RT with concomitant and adjuvant temozolomide became the standard of care for newly diagnosed GBM (Figure 5-2). Recently, updated results from this study showed that the survival benefit with temozolomide was maintained even at 5 years (9.8% were alive at 5 years with temozolomide, versus 1.9% with radiotherapy alone (hazard ratio 0.6, 95% CI 0.5–0.7; p<0.0001).87
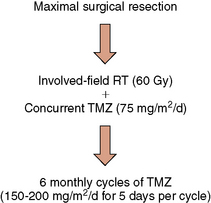
Figure 5-2 Standard treatment algorithm for newly-diagnosed glioblastoma.
Abbreviations: RT, radiation therapy; TMZ, temozolomide
In a companion study, tumor specimens from the EORTC/NCIC study were evaluated for methylation status of the MGMT gene promoter.8 MGMT is an endogenous DNA repair enzyme that removes alkyl groups from DNA and thus confers resistance to temozolomide and other alkylating agents. Methylation is an epigenetic modification that results in decreased gene transcription. Therefore, tumor cells with methylated MGMT promoters have less MGMT enzyme, resulting in less effective DNA repair and increased temozolomide sensitivity. As predicted, the benefit of temozolomide was more dramatic in patients with MGMT promoter methylation. Among GBM patients with MGMT promoter methylation who were treated with temozolomide, median survival was 21.7 months and 2-year survival, 46%. Temozolomide-treated patients with unmethylated MGMT promoters had a significantly shorter median survival of only 12.7 months, and a 2-year survival of 13.8%.8 Because this study was conducted retrospectively in a relatively small sample of patients, temozolomide remains the standard of care for newly diagnosed GBM patients, regardless of MGMT promoter methylation status. A randomized phase III trial sponsored by the Radiation Therapy Oncology Group (RTOG 0525) will definitively evaluate the utility of MGMT promoter methylation in determining temozolomide sensitivity. In the future, patients whose tumors have unmethylated MGMT promoters may be offered alternatives to the standard temozolomide regimen. Investigational approaches to suppress MGMT activity include dose-intense temozolomide regimens (e.g., 21 days on/7 days off,88 7 days on/7days off,89 or continuous dosing90), which may deplete the enzyme,91 and combination therapy with O6-benzylguanine or other MGMT inhibitors.92–94 Inhibitors of other DNA repair enzymes such as poly-(ADP-ribose)-polymerase (PARP) may also improve the efficacy of temozolomide.95
An alternative to systemic chemotherapy involves the implantation of carmustine-containing biodegradable wafers (Gliadel® wafers, MGI Pharma, Bloomington, Minnesota) into the resection cavity following tumor debulking. These wafers gradually release carmustine over several weeks, allowing for a high local chemotherapy concentration with minimal systemic toxicity. In a double-blind, randomized, phase III trial of 240 newly diagnosed MG patients, patients received either conventional therapy (surgery followed by RT) with placement of a carmustine wafer or conventional therapy with placement of an identical placebo wafer. Carmustine wafer treatment increased median survival from 11.6 to 13.9 months (P = 0.03).96 Carmustine wafers were FDA-approved for treatment of newly-diagnosed GBM on the basis of these data.
Although chemotherapy is often considered in treating recurrent MGs, the benefit of standard chemotherapy has been modest in this setting. Phase II trials of temozolomide for recurrent glioblastomas have demonstrated response rates of only 5%, with stable disease in 40% of patients and 6-month progression-free survival (PFS6) in the range of 21%.97–99 Alternative temozolomide dosing regimens may be more effective. Other agents such as carmustine, carboplatin, etoposide, irinotecan, and PCV (procarbazine, lomustine [CCNU], and vincristine) combination therapy produce low response rates and no significant survival benefit.100 In selected patients with recurrent glioblastomas who can undergo resection, carmustine-containing biodegradable wafers (Gliadel® wafers, MGI Pharma, Bloomington, Minnesota) produces a modest survival advantage of approximately 8 weeks.101 In light of limited data, treatment decisions for patients with recurrent glioblastomas must be made on an individual basis. Factors to consider include tumor histology, prior therapy, time to relapse, and performance status. In general, patients with recurrent disease should be enrolled in clinical trials whenever feasible.