Hearing Loss and Cochlear Implantation in Children
Hearing loss is the most common congenital sensory impairment, with an incidence of 2 to 3 per 1000 live births. Approximately half of these newborns have severe-profound loss. Hearing loss can significantly hinder a child’s expressive and receptive communication and, as a result, be detrimental to cognitive, educational, and psychosocial development. Fortunately, with the advent of universal newborn hearing screening, which is now mandated in most states, hearing loss is usually discovered very early in life. This screening provides the opportunity to intervene in the nascent stages of childhood development. Early intervention has far-reaching impact not only in terms of furthering a child’s communication abilities, but also in its potential to change the direction of a child’s life.
Cochlear implantation has become a very successful treatment option for some children with severe-profound hearing loss. Over the past several decades, significant advances in implant technology, coupled with increased understanding of auditory development, have enabled many hearing impaired children to successfully become a part of the “hearing world.”
Types of hearing impairment
To understand the role of cochlear implantation, the different types of hearing impairment must first be understood. Hearing loss is primarily characterized as conductive or sensorineural in nature. Mixed hearing loss is a combination of the two. Another category of hearing impairment is auditory neuropathy spectrum disorder (ANSD).
Conductive hearing loss occurs when sound transmission is physically blocked between the opening of the external ear and the cochlear hair (receptor) cells. The most common cause of conductive hearing loss in children is otitis media and related conditions, such as middle ear effusion and Eustachian tube dysfunction. Conductive loss has numerous other causes, including cerumen impaction, tympanic membrane perforation, and ossicular fixation or disruption. In a conductive loss, no dysfunction of the cochlear hair cells or auditory nerve is present. Cochlear implantation is not used to treat conductive hearing losses.
A sensorineural hearing loss (SNHL) is caused by a neural defect in the transmission of sound at the level of the cochlea, which is where the receptor cells for sound, known as hair cells, are found. SNHL may be congenital or acquired, and both congenital and acquired losses may be hereditary (genetic) or nonhereditary in nature. Approximately 30% of hereditary hearing loss is considered syndromic. More than 400 genetic syndromes that include hearing loss have been described. The other 70% are nonsyndromic, or not associated with any visible abnormalities or medical problems. Well-recognized risk factors for SNHL in infants and children include family history of childhood SNHL, birth weight less than 1500 g, low Apgar scores, craniofacial anomalies, hypoxia, in utero infections (eg, TORCH), and history of mechanical ventilation for more than 5 days.
SNHL is also characterized as being prelingual or postlingual. Prelingual hearing loss is present before speech develops and is very often congenital; postlingual hearing loss occurs after the development of normal speech.
Auditory neuropathy was first recognized as an entity in the 1990s, and was renamed ANSD in 2008 [1]. It is diagnosed when there is a discrepancy between cochlear and neural function. In ANSD, the outer hair cells function normally, but sound is not properly transmitted from the outer hair cells to the auditory cortex. This dysfunction is thought to be caused by desynchronized action potentials in the auditory nerve. Presentation varies widely. Children with ANSD may have hearing thresholds that range from normal to profound levels on audiometric testing; however, they usually exhibit disproportionately poor speech perception abilities for their degree of hearing loss. Some patients with ANSD have only very mild impairment, whereas others present as functionally deaf. Some have impairment that fluctuates in severity. Children with ANSD typically have difficulty hearing in noise. ANSD children may hear words but have difficulty understanding what is being said.
ANSD may not be detected on newborn hearing screening if otoacoustic emissions (OAE) testing is used, because this modality only tests the function of the cochlear outer hair cells, and not the auditory nerve. Auditory brainstem response (ABR) is either absent or grossly abnormal on testing. ANSD accounts for a relatively small percentage of hearing impairment. Risk factors include prematurity, severe jaundice, low birth weight, and hypoxia. It is genetic in some cases. Some children with ANSD actually have deficient or absent cochlear nerves, whereas others have global neuropathies, such as seen in Charcot-Marie-Tooth disease or Friedreich ataxia.
Cochlear implantation is an option for some children with severe-profound sensorineural hearing loss or significant impairment caused by ANSD.
The cochlear implant
The U.S. Food and Drug Administration (FDA) approved the modern, multichannel cochlear implant in 1985 for use in adults. The FDA approved the device in 1990 for children down to the age of 2 years, and in 2002 down to the age of 12 months.
A cochlear implant is not a hearing aid. Hearing aids merely amplify sound. A cochlear implant bypasses the cochlear hair cells and directly electrically stimulates the auditory nerve. It does not restore normal hearing, but rather allows for the perception of sound sensation.
The device has internal and external parts (Figs. 1 and 2). The external parts include a microphone, speech processor, and transmitter. The microphone picks up sounds from the environment. The speech processor digitizes the sounds and then these signals are sent via the transmitter to the internal portion of the device, which is surgically implanted. The receiver, which is under the scalp, picks up the signals and delivers them to the electrode array that is inserted into the cochlea. The electrodes along the array directly stimulate the auditory nerve. The cochlea is tonotopically organized: high frequencies are picked up at the base of the cochlea, and low frequencies are detected at the apex of the snail-shaped organ.
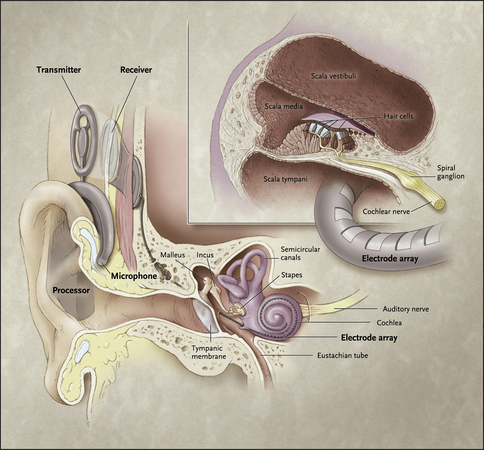
Fig. 1 This diagram shows how the internal and external portions of a cochlear implant are positioned. The microphone, which is worn on the ear, picks up sounds which are then digitized by the speech processor. The transmitter sends these signals through the scalp to the receiver, and the impulses are sent into the electrode array which directly stimulates the auditory nerve.
(Reprinted from Papsin BC, Gordon KA. Cochlear implants for children with severe-to-profound hearing loss. N Engl J Med 2007;357:2380–7; with permission.)
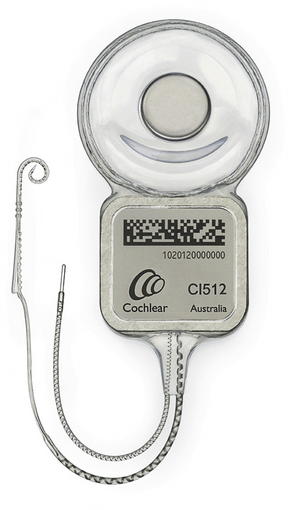
Fig. 2 The internal portion of a cochlear implant. The receiver is surgically implanted under the scalp, and has a ground electrode and a curved electrode array which is placed in the cochlea.
(Photo provided Courtesy of Cochlear Americas, 2009 Cochlear Americas; with permission.)
Currently available cochlear implants have up to 24 electrodes along the array. The speech processor contains software that transforms sounds into electrical signals. The software contains speech-encoding algorithms designed to optimize the conveyance of speech information. Different manufacturers use different processing strategies and no one strategy has consistently produced better overall results than the others. As of this writing, there are three major manufacturers in the world.
Candidacy for implantation
Before any discussion regarding candidacy for implantation, it is important to note that not all people desire cochlear implants or wish to be a part of the “hearing world.” Some view deafness not as a disability, but rather a different human experience. For this population, Deafness is a cultural identity, much like an ethnic identity, and the capital “D” is used to signify use of the term as a cultural label. American Sign Language (ASL) forms the linguistic basis for their identity. The Deaf population prides itself on being a close-knit, supportive community, with its own language and rich heritage. Thus, cochlear implantation, and the view that hearing-impaired children must be brought into an oral-hearing world, are antithetical to the Deaf identity, and have been the topic of many contentious debates. Both sides of this debate share the common goal of promoting language development in and bettering the life of deaf children. Both sides have pros and cons.
The decision of whether to implant a child is pivotal, and one of lifelong consequence, because it can profoundly influence the future direction of a child’s life. It is a complex decision based on many factors in addition to hearing status, such as available educational options and support systems. The surgical placement of the device alone does not result in improved hearing and, in fact, is only the beginning of a long and involved learning process. Success depends on motivation and a long-term commitment to therapy and education. For this reason, in many centers a team approach is used to evaluate candidates, with the involvement of otolaryngologists, audiologists, speech–language pathologists, social workers, psychologists, and educational specialists.
From a medical standpoint, a candidate must be healthy enough to withstand general anesthesia and surgery, and must have a cochlea and an auditory nerve. Rarely, deafness is the result of an absent auditory nerve or cochlear agenesis. Other cochlear and inner ear malformations are not usually contraindications to surgery but must be evaluated on a case-by-case basis.
Obtaining an accurate assessment of an infant or child’s hearing status is necessary to determine candidacy. Previously, the belief was that only children with bilateral profound (pure-tone averages >90 dB) sensorineural hearing loss, receiving little to no benefit from hearing aids, should be considered for implantation. However, as understanding of auditory development and outcomes has evolved, the criteria have broadened to include patients with greater degrees of residual hearing. The trend has been toward a more functional assessment of a candidate’s hearing, with a focus on language acquisition abilities, instead of reliance on pure-tone averages alone.
All cochlear implant candidates should receive a trial of binaural amplification, fit appropriately, according to American Academy of Audiology pediatric hearing aid fitting guidelines. A 3- to 6-month trial of hearing aid use, in conjunction with intensive therapy, is typically necessary to evaluate benefit. To determine whether the hearing aids are providing enough useful auditory input to allow for communication and language acquisition, open-set aided speech recognition testing is performed in older children. In younger children, closed-set speech recognition tests may provide useful information. The FDA criteria vary slightly for different manufacturers’ devices, but speech discrimination scores of 50% to 60% or below with optimal amplification are currently required for candidacy. Receiving benefit from amplification no longer disqualifies one from receiving a cochlear implant, because most patients who are able to recognize approximately 50% of words using hearing aids have now been shown to generally have even higher speech recognition scores postimplantation, compared with their best preoperative scores [2,3]. For children with asymmetric hearing loss, the literature supports continued use of a hearing aid in the better-hearing, nonimplanted ear, with implantation of the worse-hearing ear. Greater residual hearing at implantation and shorter duration of deafness seem to be associated with improved language outcomes; conversely, poorer outcomes seem to be associated with prolonged use of hearing aids before implantation [4,5].
For infants and very young children who cannot undergo speech perception testing, the assessment relies heavily on a team approach. Audiometric testing and appropriately fit hearing aids are the first requirement, and then evidence of benefit, or lack thereof, is based on regular audiologic evaluations, results of outcome questionnaires, and reports from parents and early intervention providers. A speech-language pathologist who is familiar with this population works collaboratively with the implant audiologist to assess progress in auditory skills. Because the evaluation of infants is not straightforward, a hearing aid trial of at least 6 months, with appropriate early intervention and speech-language services, is usually desired before making a decision regarding implantation.
A situation in which the decision to implant is often expedited is when deafness occurs secondary to bacterial meningitis, particularly if Streptococcus pneumoniae is the pathogen. In these cases, because of the powerful inflammatory response triggered by the pneumococcal cell wall, rapid fibrosis and ossification of the cochlea may occur, often beginning within several weeks of the infection [6]. Ossification can lead to a more difficult surgery, incomplete electrode insertion, and poorer outcomes. For this reason, many advocate a more aggressive time line for surgery, including implantation under the age of 12 months if necessary. This recommendation is a matter of some debate, because hearing recovery in postmeningitic patients has been reported; for advocates of the watchful waiting approach, serial MRI scanning is performed, with urgent cochlear implantation for signs of ossification.
The age of implantation continues to decrease. Understanding of auditory development and brain plasticity has given physicians the impetus to implant children at a younger age, and earlier implantation has been correlated with improved communication outcomes [4,7–12]. A narrow window of time exists during development, known as a sensitive period, within which the central auditory cortex must be stimulated by sound if it is to develop normally. If the auditory cortex is deprived of stimulus during this period, it will reorganize to be used for other purposes. For example, studies using functional MRI have shown that in deaf individuals, areas of the auditory cortex were activated by visual stimuli; this was not seen in normal hearing controls [13]. Because of this reallocation of brain function, once the sensitive period has passed, auditory development becomes much more challenging.
Sharma and colleagues [13,15] looked to assess the effect of age at cochlear implantation on auditory development and found that the brain is maximally plastic for the first 3.5 years, and that the optimal time for implantation is within this period. Plasticity then declined, but was found to remain in varying degrees until approximately 7 years of age, which marked the end of the sensitive period. Some studies have shown that different language skills may have different sensitive periods. For example, one study concluded that there might be an earlier sensitive period for vocabulary-building skills than for speech perception skills [7].
Research has been directed toward further narrowing down the optimal age for implantation. No consensus has been reached, but most literature supports that younger is better [7,10,16]. The FDA currently approves cochlear implantation down to 12 months of age, but some centers have been implanting even younger infants.
As understanding of ANSD has grown, cochlear implants have also become recognized as a viable option for some children with this disorder. One might question the efficacy of a cochlear implant in patients with abnormal auditory nerve conduction, but it is postulated that the discrete electrical signals produced by a cochlear implant may be more effective at eliciting a synchronous neural response than acoustic stimuli. Several studies report that children with ANSD derive benefit from cochlear implantation, and some report outcomes comparable to those of implanted children with SNHL [17–20]. In 2008, a consensus panel recommended that cochlear implants should be considered in children with ANSD, regardless of audiometric threshold, if progress is not being made in language development, despite appropriate amplification.
However, children with ANSD are a heterogeneous group, and not all patients with ANSD should be implanted. Research has shown that for individuals with a cochlear nerve deficiency or hypoplastic cochlear nerve, outcomes are much poorer [21,22]. This does not mean that this group should automatically be excluded from consideration, but they should be carefully assessed for any auditory sensation, and expectations should be tempered.
There is also a chance that auditory function may improve in ANSD. This has been documented in several studies. In a 2002 study, 50% of children with ANSD and evidence of severe SNHL spontaneously improved, with a mean improvement time of 5.8 months. The children whose ANSD was attributed to hyperbilirubinemia had a greater tendency to improve. These children achieved a stable audiogram at a mean age of 18 months [23]. Thus, caution must be exercised before proceeding with implantation in infants and very young children who carry this diagnosis.
Cochlear implant programs in the past tended to exclude deaf children with other handicaps, but the number of children with other disabilities being implanted is increasing rapidly, and accounts for a large percentage of implant recipients at some centers. For most children, the goal of implantation is spoken language. For children with developmental delays or other disabilities, the goals of implantation may be different. Speech and language outcomes may be limited by cognitive deficits, but these children may still derive benefit from improved use of language, as well as sound awareness, and increased environmental and social connectedness. Communication by nonverbal modalities may still be supported by sound. Mere sound awareness can be useful in terms of social attachment, attention, and safety. Thus, these children should not automatically be excluded from implant candidacy [24,25].
In addition to audiometric, speech, language, and medical considerations, candidacy evaluation must also include assessments of a child’s support systems, psychosocial situation, and educational environment. The importance of these aspects cannot be underestimated. A cochlear implant recipient must have access to appropriate therapy and educational services to learn to understand speech and develop spoken language. This may be difficult in some rural areas or in areas with a low population of deaf or implanted children. Efforts must be made to line up these services during the candidacy process. The potential recipient must be motivated and have adequate family/caregiver support to participate in intensive therapy and education. A psychological evaluation may be needed to assess whether a child has met general and cognitive developmental targets and whether he/she will be able to use the device effectively. It may also identify behavioral issues or other areas of concern that may affect candidacy.
It is critical that potential implant recipients and their families have realistic expectations regarding the implant and outcomes, and that they be willing to revise their expectations throughout the process. While a cochlear implant may allow a child to “hear” sounds, there are always limitations, and there is no guarantee that a child will be able to function as a hearing child. Numerous factors, some intrinsic and others extrinsic to the child, will influence outcome. A cochlear implant does not cure deafness, and the recipient and family must understand that hearing with a cochlear implant is different from how most people perceive sound. Perhaps most important is the understanding that language comprehension and the ability to speak will not develop without intensive, long-term therapy and education, and significant effort on both the part of the recipient, his/her family, and the educational system.
The Children’s Implant Profile, often referred to as ChIP, was devised in 1991 as a systematic method for determining implant candidacy, and has been widely used around the world. ChIP examines 11 factors that contribute to successful implantation and rates them on a 3-point scale. These include age, duration of deafness, medical or radiologic anomalies, secondary handicaps, functional hearing level, speech-language abilities, family support, expectations, educational environment, availability of support services to monitor implant use, and cognitive learning style [26]. Several modifications of the protocol have been made since then [27,28].
Surgical and medical considerations
The surgeon assesses the candidate in terms of medical risk and surgical feasibility. Comorbid medical conditions, cognitive status, and otologic history are all taken into account. The patient must be stable enough to undergo general anesthesia and withstand surgery and the potential, realistic benefit for that individual must outweigh the risks.
All cochlear implant candidates must be evaluated with radiographic imaging. High resolution computed tomography (CT) scanning of the temporal bone is obtained to evaluate any anatomic abnormalities of the inner ear structures, such as a dysplastic cochlea or enlarged vestibular aqueduct. Use of magnetic resonance imaging (MRI) is becoming more widespread for preoperative evaluation because of its superior ability to reveal soft tissue abnormalities of the inner ear, such as cochlear nerve hypoplasia or agenesis, cochlear fibrosis, or dilated endolymphatic sac [29]. Findings such as a cochlear nerve asymmetry or cochlear obstruction might influence the decision to implant one side over the other. In all cases of ANSD, MRI should be obtained to evaluate for cochlear nerve hypoplasia or absence. Lack of a cochlear nerve would be a contraindication to surgery. MRI of the inner ear and brain should also be obtained in children with other neurologic pathologies, at least as a baseline, since an MRI cannot easily be obtained once a child has been implanted due to potential damage to the device and compromise of the image due to artifact.
All cochlear implant recipients are at increased risk for bacterial meningitis. An opening is created in the cochlea during surgery to accommodate insertion of the electrode. Although the opening is plugged with soft tissue, it remains a potential route through which bacteria, such as those causing acute otitis media, may enter the inner ear and cerebrospinal fluid. Some inner ear malformations associated with hearing loss also inherently place patients at increased meningitis risk. Therefore, all cochlear implant candidates and recipients should receive all age-appropriate doses of pneumococcal conjugate and Haemophilus influenza type b conjugate vaccines, in addition to an annual influenza vaccination. At age 24 months, the 23-valent pneumococcal polysaccharide vaccine should also be administered. The tetravalent meningococcal vaccine should be considered, but there is no evidence that individuals with cochlear implants are at any increased risk for meningitis caused by N. meningitidis. Because of the meningitis risk, acute otitis media at the time of planned implant surgery is a contraindication to proceeding. Tympanostomy tube placement should be considered before implantation in patients with recurrent acute otitis media or chronic middle ear effusions [30].
With large numbers of children being implanted during their most otitis-prone years, primary care providers are becoming more likely to encounter children with cochlear implants and acute otitis media. Acute otitis media must be treated aggressively in this population, without delay. The risk of complications from acute otitis media may be higher in the first few months after implantation. Empiric treatment with an oral antibiotic such as high-dose amoxicillin or amoxicillin-clavulanate is appropriate if the patient is at least 2-months post-implant surgery, he/she does not have an inner ear malformation, he/she does not appear severely ill, and the cochlear implant does not have a spacer/positioner. (This was found in an implant model which was available from 1999 through 2002, when it was voluntarily recalled from the market.) For patients who do not meet these criteria, parenteral antibiotics, such as ceftriaxone or cefotaxime, are recommended as initial treatment. Referral to an otolaryngologist is appropriate if symptoms worsen despite 24 hours of antibiotic therapy [30].
Concerns have been raised about the safety of implanting very young infants. Issues unique to this population, from a surgical standpoint, include thinner and more delicate tissues, lower circulating blood volume, large amounts of calvarial bone marrow, decreased pneumatization of the temporal bone, and more superficial position of the facial nerve. However, studies support the safety and efficacy of the procedure, and preliminary data seem to indicate language growth rates comparable to normal hearing children [31,32].
The implant procedure
The typical cochlear implantation procedure is described here. The surgery is performed in the supine position under general anesthesia. Paralytic agents are not used, as intraoperative facial nerve monitoring is performed. (The facial nerve traverses the surgical area and is therefore at some risk.) Perioperative intravenous antibiotics are administered to cover normal skin flora. The facial nerve monitor electrodes are placed, the postauricular area is injected with local anesthetic with epinephrine, and then the patient is prepped and draped in sterile fashion. A skin incision is created in the postauricular area and carried down to expose the mastoid area and cranium. Posterior and superior to the ear, most surgeons drill a depression or well in the skull in which the implant receiver will sit. Next, a mastoidectomy is performed: the mastoid bone, immediately behind the ear, is opened using a surgical drill, down to a depth where the lateral semicircular canal and incus can be seen; the middle ear space is accessed from here (Fig. 3). An opening called the facial recess is created, and through this, one can see the bone overlying the cochlea. A cochleostomy, or hole, is created into the scala tympani of the cochlea through which the implant electrode will be threaded (Fig. 4). A trough is drilled to connect the well with the mastoidectomy, providing a channel for the electrode. The cochlear implant is then positioned and secured in the well, the electrode array is threaded into the cochlea, and the cochleostomy is plugged with small pieces of soft tissue such as temporalis muscle or fascia (Figs. 5 and 6). The incision is then closed in layers and a head-wrap dressing is applied. After the implant has been placed, many centers perform intraoperative testing to measure implant electrode impedances and to verify and record neural responses to electrical stimuli. Plain films or fluoroscopy may also verify electrode placement. Cochlear implantation is frequently performed in an ambulatory surgery setting.
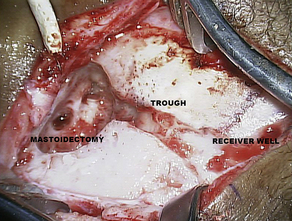
Fig. 3 This photograph shows the temporal bone in the postauricular area after the receiver well, mastoid, and trough have been drilled. This is a left ear.
The cochlear implant surgical procedure has evolved over time. In the past, generous areas of hair were shaved as part of the surgical preparation, large incisions and scalp flaps were created, and large wells were drilled in the skull to accommodate the receiver, often exposing dura. Holes were drilled in the skull through which sutures were passed to tie down the implant. As with many other surgeries, the trend has been toward making cochlear implantation minimally invasive. The implants themselves have become smaller over time, making possible some recent changes in technique. Cochlear implants are now being placed through significantly smaller skin incisions, often without any hair being shaved. Some surgeons do not drill a well, but rather just create a pocket under the pericranium to hold the receiver. Some do not secure the implants with sutures. Others feel that without one or both of these steps, the device is at significantly increased risk for displacement.
The potential major and minor risks of cochlear implant surgery which are typically stated on a surgical consent form include the following: facial nerve injury, meningitis, cerebrospinal fluid leak, perilymphatic fluid leak, vertigo, hematoma, seroma, wound infection, tinnitus, taste disturbance, ear numbness, and device failure. The published data on rates of complications in cochlear implant surgery is somewhat limited, but the overall rate of major perioperative complications in the pediatric population is low and compares favorably with that of the adult population [33]. The revision surgery rate in the pediatric population, however, is higher than is seen in adults, with a quoted rate of 8–13%, as compared with 5.4–6.9% in adults [34,36]. Cited reasons for revision implantation surgery included internal device failure (“hard failure”), scalp flap complications, device migration, infection, electrode extrusion, technology upgrade, unexplained deterioration of performance (“soft failure”), and facial nerve stimulation. In all articles reviewed, “hard failure” of the device accounted for the most revision surgeries. Head trauma was noted to precede almost half of the pediatric hard failures in one study [37]. For this reason, some implant centers discourage participation in contact sports among recipients. Fortunately, studies report excellent outcomes for reimplantation surgery, with equal or improved performance in most cases [38].
After cochlear implant surgery
After cochlear implant surgery, most centers will wait approximately 2–4 weeks before activating the device to allow time for healing. There is no ability to hear with the implant during this period. At the time of activation, the audiologist will place the external device (transmitter) directly over the receiver which is implanted under the scalp; this is held in place by a magnet. The internal portion of the cochlear implant is then checked by computer to ensure that it is working properly; communication between the external and internal devices is checked, along with each individual electrode. The speech processor is then programmed or “mapped.” Each electrode is turned on and a current is delivered until the patient hears a tone; this is the threshold. The loudness is then increased until a comfortable listening level is achieved. After each electrode has been mapped, this information is downloaded into the speech processor and the implant is activated. For infants, mapping is primarily based on objective measurement of neural responses to stimulation, but also relies on behavioral responses as observed by the parents and audiologist. The reaction at switch-on is different for each child, but common reactions include crying or a look of bewilderment in infants and toddlers, and excitement, happiness or anxiety in older children. At the beginning, sounds may seem garbled and unfamiliar, as the brain must adapt to processing the new input, but over time, sounds become clearer and gain meaning. As the recipient becomes familiar with and is better able to use the implant, the audiologist will adjust mapping and fine-tune the settings to enhance the quality of sound for the individual.The initial activation visit usually takes several hours and may take place over two visits. Mapping visits occur frequently early-on, and then once a stable map is achieved, typically after about 2 months of use, visits may be spaced out to every 3 months, and then every 6 months to yearly. Stimulation thresholds change over time, so monitoring and adjustments are necessary throughout life. In addition, a typical therapy schedule for infants and young children is twice a week for the first 1–2 years after implantation, and then weekly until school begins, at which point, therapy usually takes place through school.
Once a patient has a cochlear implant, there are some important precautions and restrictions which must be remembered. The external device is not waterproof, so recipients can swim but must first remove the external portion of the device. MRI is contraindicated because the powerful magnetic field created by MRI scanners can damage the implant. Some newer implant models are MRI compatible and can withstand up to 1.5 Tesla, and there are also some models with removable magnets in case MRI scanning should be necessary. A brief surgical procedure is necessary to remove the magnet. Monopolar electrosurgical instruments should not be used in the head or neck of a cochlear implant recipient, as the currents produced could flow through the electrode array and cause damage to the implant or cochlear tissue. A common scenario in which this can be a factor is when a cochlear implant patient requires a tonsillectomy or adenoidectomy. In general, bipolar cautery may be safely used. Ionizing radiation therapy can damage the implant. The sound from a cochlear implant can be distorted when in close proximity to sources of electromagnetic interference which include radio or television transmission towers, airport metal detectors and some types of mobile communications equipment, including some mobile phones. Static electricity can potentially damage the device or, more commonly, erase the speech processor program. Certain activities known to create significant electrostatic discharge, such as sliding down a plastic slide, should either be avoided, or the processor should first be removed.
Bilateral implantation
While unilateral cochlear implant users appreciate significant benefit as compared with severe-to-profoundly deaf patients without an implant, they still experience the problems associated with unilateral deafness, such as difficulty with localization of a sound source and listening in background noise. Some still benefit from hearing aid use in the contralateral ear, but for many this provides no recognizable benefit. In recent years, with the backing of research which has examined auditory outcomes, safety, efficacy and cost-utility, bilateral implantation has become a standard medical practice where clinically appropriate [39,40].
The advantages of bilateral implantation which have been reported in multiple studies include improved speech perception, not only in noisy environments, but also in quiet settings. Bilateral implant users have shown improved abilities in localizing the direction from which a sound originates. Another benefit cited is the fact that with two implants, it is less likely that a recipient will have to spend time “off the air” if the batteries should lose charge, or if one of the devices should fail [39]. There is also evidence of specialization of the auditory cortices in each hemisphere [41]. Thus, it seems reasonable to conclude that input from two implants may complement each other, each providing slightly different information.
Bilateral implantation can be performed simultaneously or sequentially. Advantages of simultaneous implantation include the need for only one surgery and anesthetic, as well as the ability to activate both devices at the same time. Disadvantages include longer operative time on a given day, with greater blood loss and potentially increased anesthetic risk. The blood loss factor may be significant in infants who inherently have a lower circulating blood volume and a marrow-filled temporal bone. If sequential bilateral implantation is chosen, it is recommended that the second implant be placed with minimal delay [41,42]. In a 2009 study, Gordon and Papsin reported better speech perceptions scores for children in whom the inter-implant delay was less than 12 months, as compared with those in whom the delay was more than 2 years. It is postulated that there are sensitive periods for development of binaural hearing, so this may be, at least in part, a function of age. Peters and colleagues [43] found that children younger than age 8 who had received a sequential second implant had a faster rate of improvement and achieved a higher mean speech perception score than children in the 8–13 year age group.
Outcomes
The goal of most cochlear implant recipients is to develop age-appropriate expressive and receptive language abilities; however, there is significant variation in performance. In general, the best outcomes are seen in cognitively normal children who were implanted at an early age, who have motivated families and educators, and who are in an environment rich in oral communication. Many children are able to achieve open-set speech recognition within the first year of implantation [44]. A 10-year follow up study out of France reported that with cochlear implants, many profoundly hearing-impaired children are able to develop functional speech perception and production and achieve satisfactory performance in school [9]. In this study, 79% of implant recipients were able to use the telephone, 67% developed intelligible speech, and 78% were able to attend mainstream schools. Recipients averaged 72% open-set word recognition in quiet settings and 45% in noise. These children had more difficulty with receptive language, with 75% scoring below the median value of their normal-hearing peers. A 2005 English study showed similar findings 10–14 years post-implant, with 87% able to understand conversations without visual cues such as lip-reading, 60% able to speak on the telephone with a familiar speaker, and 77% able to produce intelligible speech [45].
Despite the significant benefits seen in word recognition and speech intelligibility, however, many children with cochlear implants still experience difficulties with elements of communication, such as syntax, semantics, and pragmatics [25]. And because of the multifaceted and integrated nature of language and communication development in the brain, deafness affects nonauditory skills, such as attention, reading, and written language. The average 18-to-19 year old deaf student reads at the level of an average normal-hearing 9 year old. Several factors have been identified as contributing to this deficiency, including the fact that most normal-hearing children learn to read by drawing upon their knowledge of spoken vocabulary and grammar. Early implant recipients with normal cognition may have less impairment, but most implanted children still lag behind normal hearing children in reading skills [11,25,46,47].
Several factors influence outcomes in children. Shorter duration of deafness before cochlear implantation was an important positive predictor of better outcome [12]. This generally correlates with younger age at time of implantation. It is also suspected that with a shorter duration of deafness, it is likely that more of the auditory system is intact and better able to process the signals produced by a cochlear implant. This supports the idea of implantation in the setting of residual hearing [5].
As discussed earlier in this article, early implantation minimizes the period of sensory deprivation and takes advantage of sensitive periods in auditory development. Numerous studies correlate younger age at implantation with improved outcome, but as of yet, there is no clear agreement on the optimal age for implantation. In several studies, the best outcomes are seen in recipients age 2 years and under [4,10,48]. Promising results have been reported in children implanted under 12 months of age but other studies have found no significant language outcome advantage in children implanted before 12 months of age as compared with children implanted at 13–24 months [16,32].
The recipient’s method of communication and educational environment also influence outcome. Although children using both total communication (oral and sign language) and oral communication make gains in language acquisition after implantation, oral communicators overall outperform total communicators [49,51]. Long-term follow up of a large group of cochlear implant recipients found that those who relied on spoken language early on fared better in speech perception, speech intelligibility, verbal rehearsal skills, and literacy [50].
Other factors noted to influence language outcomes include socioeconomic status and strength of parent-child interactions [4]. Perhaps not surprisingly, one of the strongest influences on outcome, after correcting for other factors, was found to be the recipient’s nonverbal intelligence [49,52].
For children with ANSD, studies indicate that cochlear implantation is beneficial and most studies report outcomes that are not significantly different from those seen in non-ANSD implanted children [17,18,20]. The exception was in children who had preoperative MRI evidence of central nervous system pathology and/or cochlear nerve hypoplasia; this group had poorer language-acquisition outcomes [21,22].
Children with inner ear malformations frequently undergo cochlear implant surgery, and despite more frequent intraoperative difficulties such as perilymphatic leaks and aberrant facial nerve anatomy, outcomes are often very successful [53,54]. However, the various types of inner ear malformations have different prognoses. Buchman and colleagues found that children with an incompletely partitioned cochlea, enlarged vestibular aqueduct (EVA) and dilated vestibule (Mondini malformation), as well as children with isolated EVA or partial semicircular canal aplasia had better outcomes in terms of speech perception than children with total semicircular canal aplasia, isolated incomplete partitioning, cochlear hypoplasia or common cavity deformity. Papsin also found poorer performance in children with narrowed internal auditory/cochlear canals, common cavity deformity, and a hypoplastic cochlea. However, both authors noted that these deformities should not automatically preclude a candidate from implantation, and that individual evaluation is necessary.
Cost effectiveness and quality of life
In the current health care environment, with economic pressures discouraging the use of expensive technologies, much attention has turned toward cost-effectiveness studies of new innovations. Cost-effectiveness and quality of life outcomes have been difficult to assess in children, as they often rely on parental reporting, and normative data in this population, with its diverse developmental phases, is scarce [55]. However, available studies support cochlear implantation as a cost-effective intervention with significant societal cost-savings [56,57]. One study estimates the lifetime cost of profound deafness to exceed $1 million, and estimates that cochlear implantation may save $53,198 per child when reduced educational costs and increased earnings are taken into account. The pediatric cochlear implant fares well in terms of cost-utility when compared with several other common medical interventions [40,57].
Summary
In the mid-1980s, it was discovered that birds, unlike humans, have the ability to produce new cochlear hair cells after acoustic trauma or ototoxic drugs had damaged existing hair cells. Since then, research in hair cell regeneration has been one of the most active areas of inquiry in the hearing sciences. Many scientists are working furiously to unlock the key to human hair cell regeneration and to thereby make hearing restoration possible. This, or another breakthrough, may one day render the cochlear implant obsolete. But until that time comes, we work to advance implant technology and to better understand how to get the most out of these devices.
Children have now been receiving cochlear implants for approximately the past two decades. Implantation has become a standard option for many patients with severe-profound hearing loss and has become a possibility for populations who were not previously considered appropriate for this technology. Cochlear implants provide these children with the opportunity to become a part of the hearing world, or at least to allow sound perception to enrich their lives. Studies to date support the idea of sensitive periods of auditory development, and indicate that implantation at a very early age provides recipients with the best language acquisition outcomes, particularly in an environment of oral communication. Bilateral implantation offers the advantages of binaural hearing and should be considered when implantation is being evaluated.
The first pediatric multichannel cochlear implant recipients are just now entering adulthood. Research on these subjects is giving us insight into how this technology can impact recipients across the lifespan, from infancy into later years. We still have much to learn about auditory development, cognition and how these and other factors influence outcome.
Acknowledgments
Thank you to Jennifer Kolb, M.Ed., CCC-A and Susan Dreith, Au.D., CCC-A for their audiologic expertise.
References
[1] A. Starr, T.W. Picton, Y. Sininger, et al. Auditory neuropathy. Brain. 1996;119:741-753.
[2] R.C. Dowell, R. Hollow, E. Winton. Outcomes for cochlear implant users with significant residual hearing. Arch Otolaryngol Head Neck Surg. 2004;130:575-581.
[3] S.J. Dettman, W.A. D’Costa, R.C. Dowell, et al. Cochlear implants for children with significant residual hearing. Arch Otolaryngol Head Neck Surg. 2004;130:612-618.
[4] J.K. Niparko, E.A. Tobey, D.J. Thal, et al. Spoken language development in children following cochlear implantation. JAMA. 2010;303(15):1498-1506.
[5] B.J. Gantz, T. Rubenstein, R.S. Tyler, et al. Long-term results of cochlear implants in children with residual hearing. Ann Otol Rhinol Laryngol Suppl. 2000;185:33-36.
[6] D. Philippon, F. Bergeron, P. Ferron, et al. Cochlear implantation in postmeningitic deafness. Otol Neurotol. 2010;31:83-87.
[7] D.M. Houston, R.T. Miyamoto. Effects of early auditory experience on word learning and speech perception in deaf children with cochlear implants: implications for sensitive periods of language development. Otol Neurotol. 2010;31:1248-1253.
[8] T.P. Nikolopoulos, G.M. O’Donoghue, S. Archbold. Age at implantation: its importance in pediatric cochlear implantation. Laryngoscope. 1999;109:595-599.
[9] A.S. Uziel, M. Sillon, A. Vieu, et al. Ten-year follow-up of a consecutive series of children with multichannel cochlear implants. Otol Neurotol. 2007;28:615-628.
[10] L. DeRaeve. A longitudinal study on auditory perception and speech intelligibility in deaf children implanted younger than 18 months in comparison to those implanted at later ages. Otol Neurotol. 2010;31:1261-1267.
[11] A.E. Geers, H. Hayes. Reading, writing, and phonological processing skills of adolescents with 10 or more years or cochlear implant experience. Ear Hear. 2011;32:49S-59S.
[12] A.E. Geers, A.L. Sedey. Language and verbal reasoning skills in adolescents with 10 or more years of cochlear implant experience. Ear Hear. 2011;32:39S-48S.
[13] A. Sharma, P.M. Gilley, M.F. Dorman, et al. Deprivation-induced cortical reorganization in children with cochlear implants. Int J Audiol. 2007;46:494-499.
[14] A. Sharma, M.F. Dorman, A. Kral. The influence of a sensitive period on central auditory development in children with unilateral and bilateral cochlear implants. Hear Res. 2005;203:134-143.
[15] A. Sharma, M.F. Dorman, A.J. Spahr. A sensitive period for the development of the central auditory system in children with cochlear implants: implications for the age of implantation. Ear Hear. 2002;23:532-539.
[16] R.F. Holt, M.A. Svirsky. An exploratory look at pediatric cochlear implantation: is earliest always best? Ear Hear. 2008;29:492-511.
[17] E. Buss, R.F. Labadie, C.J. Brown, et al. Outcome of cochlear implantation in pediatric auditory neuropathy. Otol Neurotol. 2002;23:328-332.
[18] W.P. Gibson, H. Sanli. Auditory neuropathy: an update. Ear Hear. 2007;28:102S-106S.
[19] G. Rance, E.J. Barker. Speech perception in children with auditory neuropathy/dyssynchrony managed with either hearing aids or cochlear implants. Otol Neurotol. 2008;29:179-182.
[20] C. Madden, L. Hilbert, M. Rutter, et al. Pediatric cochlear implantation in auditory neuropathy. Otol Neurotol. 2002;23:163-168.
[21] J. Walton, W.P. Gibson, H. Sanli. Predicting cochlear implant outcomes in children with auditory neuropathy. Otol Neurotol. 2008;29:302-309.
[22] H.F. Teagle, P.A. Roush, J.S. Woodard, et al. Cochlear implantation in children with auditory neuropathy spectrum disorder. Ear Hear. 2010;31:325-335.
[23] C. Madden, M. Rutter, L. Hilbert, et al. Clinical and audiological features in auditory neuropathy. Arch Otolaryngol Head Neck Surg. 2002;128:1026-1030.
[24] R.F. Holt, K.I. Kirk. Speech and language development in cognitively delayed children with cochlear implants. Ear Hear. 2005;26:132-148.
[25] A. Kral, G.M. O’Donoghue. Profound deafness in childhood. N Engl J Med. 2010;363:1438-1450.
[26] J.K. Niparko, C. Lingua, R.M. Carpenter. Assessment of candidacy for cochlear implantation. In: J.K. Niparko, editor. Cochlear implants: principles and practices. 2nd edition. Philadelphia: Lippincott Williams and Wilkins; 2009:137-146.
[27] E. Lazaridis, M. Therres, R.R. Marsh. How is the children’s implant profile used in the Cochlear implant candidacy process? Int J Pediatr Otorhinolaryngol. 2010;74:412-415.
[28] L. Edwards, F. Thomas, K. Rajput. Use of a revised children’s implant profile (GOSHChIP) in candidacy for paediatric cochlear implantation and in predicting outcome. Int J Audiol. 2009;48:554-560.
[29] D.A. Parry, T. Booth, P.S. Roland. Advantages of magnetic resonance imaging over computed tomography in preoperative evaluation of pediatric cochlear implant candidates. Otol Neurotol. 2005;26:976-982.
[30] L.G. Rubin, B. Papsin, Committee on Infectious Diseases and Section on Otolaryngology Head and Neck Surgery. Cochlear implants in children: surgical site infections and prevention and treatment of acute otitis media and meningitis. Pediatrics. 2010;126:381-391.
[31] J.T. Roland, M. Cosetti, K.H. Wang, et al. Cochlear implantation in the very young child: long-term safety and efficacy. Laryngoscope. 2009;119:2205-2210.
[32] S.J. Dettman, D. Pinder, R.J. Briggs, et al. Communication development in children who receive the cochlear implant younger than 12 months: risks versus benefits. Ear Hear. 2007;28:11S-18S.
[33] D.L. Tucci, T.M. Pilkington. Medical and surgical aspects of cochlear implantation. In: J.K. Niparko, editor. Cochlear implants: principles and practices. 2nd edition. Philadelphia: Lippincott Williams and Wilkins; 2009:161-186.
[34] A.L. Marlowe, J.E. Chinnici, A. Rivas, et al. Revision cochlear implant surgery in children: the Johns Hopkins experience. Otol Neurotol. 2009;31:74-82.
[35] M. Cote, P. Ferron, F. Bergeron, et al. Cochlear reimplantation: causes of failure, outcomes, and audiologic performance. Laryngoscope. 2007;117:1225-1235.
[36] L. Migirov, R. Taitelbaum-Swead, M. Hildesheimer, et al. Revision surgeries in cochlear implant patients: a review of 45 cases. Eur Arch Otorhinolaryngol. 2007;264:3-7.
[37] R.D. Cullen, J.N. Fayad, W.M. Luxford, et al. Revision cochlear implant surgery in children. Otol Neurotol. 2008;29:214-220.
[38] J.T. Roland, T.C. Huang, N.L. Cohen. Revision cochlear implantation. Otolaryngol Clin North Am. 2006;39:833-839.
[39] G.J. Basura, R. Eapen, C.A. Buchman. Bilateral cochlear implantation: current concepts, indications, and results. Laryngoscope. 2009;119:2395-2401.
[40] B.G. Bichey, R.T. Miyamoto. Outcomes in bilateral cochlear implantation. Otolaryngol Head Neck Surg. 2008;138:655-661.
[41] K.A. Gordon, D.D. Wong, B.C. Papsin. Cortical function in children receiving bilateral cochlear implants simultaneously or after a period of interimplant delay. Otol Neurotol. 2010;31:1293-1299.
[42] K.A. Gordon, B.C. Papsin. Benefits of short interimplant delays in children receiving bilateral cochlear implants. Otol Neurotol. 2009;30:319-331.
[43] B.R. Peters, R. Litovsky, A. Parkson, et al. Importance of age and postimplantation experience on speech perception measures in children with sequential bilateral cochlear implants. Otol Neurotol. 2007;28:649-657.
[44] K.I. Kirk, S. Choi. Clinical investigations of cochlear implant performance. In: J.K. Niparko, editor. Cochlear implants: principles and practices. 2nd edition. Philadelphia: Lippincott Williams and Wilkins; 2009:191-222.
[45] E.A. Beadle, D.J. McKinley, T.P. Nikolopoulos, et al. Long-term functional outcomes and academic-occupational status in implanted children after 10–14 years of cochlear implant use. Otol Neurotol. 2005;26:1152-1160.
[46] S. Archbold, M. Harris, G.O. O’Donoghue, et al. Reading abilities after cochlear implantation: the effect of age at 5 and 7 years after implantation. Int J Pediatr Otorhinolaryngol. 2008;72:1471-1478.
[47] B. Schopmeyer. Reading and deafness. In: J.K. Niparko, editor. Cochlear implants: principles and practices. 2nd edition. Philadelphia: Lippincott Williams and Wilkins; 2009:263-265.
[48] H. Hayes, A.E. Geers, R. Treiman, et al. Receptive vocabulary development in deaf children with cochlear implants: achievement in an intensive auditory-oral educational setting. Ear Hear. 2009;30:128-135.
[49] E.A. Tobey, A.E. Geers, C. Brenner, et al. Factors associated with development of speech production skills in children implanted by age five. Ear Hear. 2003;24:36S-45S.
[50] A.E. Geers, M.J. Strube, E.A. Tobey, et al. Epilogue: factors contributing to long-term outcomes of cochlear implantation in early childhood. Ear Hear. 2011;32:84S-92S.
[51] A.M. Robbins. Rehabilitation after cochlear implantation. In: J.K. Niparko, editor. Cochlear implants: principles and practices. 2nd edition. Philadelphia: Lippincott Williams and Wilkins; 2009:269-312.
[52] A.E. Geers, J.G. Nicholas, A.L. Sedey. Language skills of children with early cochlear implantation. Ear Hear. 2003;24:46S-58S.
[53] B.C. Papsin. Cochlear implantation in children with anomalous cochleovestibular anatomy. Laryngoscope. 2005;115(Suppl):1-26.
[54] C.A. Buchman, B.J. Copeland, K.K. Yu, et al. Cochlear implantation in children with congenital inner ear malformations. Laryngoscope. 2004;114(2):309-316.
[55] F.R. Lin, J.K. Niparko, H.W. Francis. Outcomes in cochlear implantation: assessment of quality-of-life impact and economic evaluation of the benefits of the cochlear implant in relation to costs. In: J.K. Niparko, editor. Cochlear implants: principles and practices. 2nd edition. Philadelphia: Lippincott Williams and Wilkins; 2009:229-244.
[56] C. O’Neill, G.M. O’Donghue, S.M. Archbold, et al. A cost-utility analysis of pediatric cochlear implantation. Laryngoscope. 2000;110:156-160.
[57] A.K. Cheng, H.R. Rubin, N.R. Powe, et al. Cost-utility analysis of the cochlear implant in children. JAMA. 2000;284:850-856.