Limb Development
Limbs are remarkable structures that are designed almost solely for mechanical functions: motion and force. These functions are achieved through the coordinated development of various tissue components. No single tissue in the limb takes shape without reference to the other tissues with which it is associated. The limb as a whole develops according to a master blueprint that reveals itself sequentially with each successive stage in limb formation. Many of the factors that control limb development cannot be seen by examining morphology alone, but rather must be shown by experimental means or through the localization of molecules. Despite remarkable progress in understanding the molecular basis of the tissue interactions that control limb development, many fundamental questions remain poorly understood. Limb anomalies are common and highly visible. Many of these anomalies are now known to be reflections of disturbances in specific cellular or molecular interactions that are fundamental to limb development. These are discussed in Clinical Correlation 10.1 at the end of the chapter.
Initiation of Limb Development
Limb formation begins relatively late in embryonic development (at the end of the fourth week in humans) with the activation of a group of mesenchymal cells in the somatic lateral plate mesoderm (Fig. 10.1). The initial stimulus for limb development is incompletely understood. Experimental evidence suggests that signals from the paraxial mesoderm (probably based on the Hox code and ultimately dependent on retinoic acid signaling) initiates a level-specific expression of two T-box transcription factors in the lateral plate mesoderm. Tbx5 in the area of the future forelimb and Tbx4 (along with Pitx-1) in the area of the future hindlimb stimulate the expression and secretion of fibroblast growth factor-10 (FGF-10) by the local mesodermal cells (Fig. 10.2A). FGF-10 stimulates the overlying ectoderm to produce FGF-8. Soon a feedback loop involving FGF-10 and FGF-8 is established, and limb development begins.
The Tbx transcription factors appear to be the initial local driving forces of limb development. If Tbx5 expression in a mouse is prevented, forelimbs fail to develop (Fig. 10.2B). Similarly, in FGF-10 knockout mice, limbs (and lungs) do not form. Conversely, if a bead soaked with FGF-10 is implanted within the future flank region of a chick embryo, a supernumerary limb develops at the spot. Once the interaction between ectoderm and mesoderm has begun, the limb primordium contains sufficient developmental information to form a limb even if isolated from the rest of the body (a so-called self-differentiating system).
In rare instances, individuals are born without one or sometimes all limbs (amelia) (Fig. 10.3). In some cases, this situation may reflect a disturbance in the production of the transcription factors or signaling molecules that initiate limb development or the cellular receptors for these molecules.
Regulative Properties and Axial Determination
The early limb primordium is a highly regulative system, with properties similar to those described for the cleaving embryo (see p. 45). These properties can be summarized with the following experiments (Fig. 10.4):
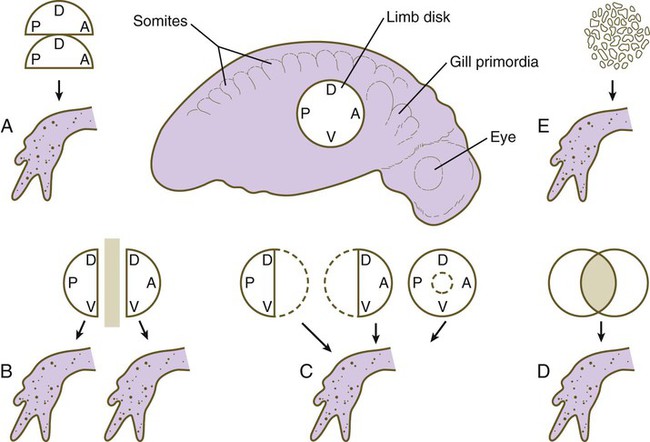
A, Combining two identical halves of limb disks results in a single limb. B, Separation of two halves of a limb disk by a barrier causes each half to form a normal limb of the same polarity. C, After various types of tissue removal, the remaining limb tissue regulates to form a normal limb. D, Combining two disks results in the formation of a single normal limb. E, Mechanical disruption of a limb disk is followed by reorganization of the pieces and the formation of a normal limb. A, anterior; D, dorsal; P, posterior; V, ventral. (Data from Harrison RG: J Exp Zool 32:1-136, 1921; and Swett FH: Q Rev Biol 12:322-339, 1937.)
1. If part of a limb primordium is removed, the remainder reorganizes to form a complete limb.
2. If a limb primordium is split into two halves, and these are prevented from fusing, each half gives rise to a complete limb (the twinning phenomenon).
3. If two equivalent halves of a limb primordium are juxtaposed, one complete limb forms.
4. If two equivalent limb disks are superimposed, they reorganize to form a single limb (see the section on tetraparental embryos [p. 45]).
5. In some species, disaggregated limb mesoderm can reorganize and form a complete limb.
The organization of the limb is commonly related to the Cartesian coordinate system. The anteroposterior* axis runs from the first (anterior) to the fifth (posterior) digit. The back of the hand or foot is dorsal, and the palm or sole is ventral. The proximodistal axis extends from the base of the limb to the tips of the digits.
Experiments involving the transplantation and rotation of limb primordia in lower vertebrates have shown that these axes are fixed in a sequential order: anteroposterior to dorsoventral to proximodistal. Early fixation of the anteroposterior axis may result from the expression of the transcription factors Gli-3 in the anterior and Hand-2 in the posterior part of the early limb field (see Fig. 10.2A). These two molecules mutually oppose each other’s actions. Before all three axes are specified, a left limb primordium can be converted into a normal right limb simply by rotating it with respect to the normal body axes. These axes are important as reference points in several aspects of limb morphogenesis. Evidence indicates a similar sequence of axial specifications in certain other primordia, such as those of the retina and inner ear.
Outgrowth of Limb Bud
Shortly after its initial establishment, the limb primordium begins to bulge from the body wall (late in the first month for the human upper extremity [Fig. 10.5]). At this stage, the limb bud consists of a mass of similar-looking mesodermal cells covered by a layer of ectoderm. Despite its apparently simple structure, the limb bud contains enough intrinsic information to guide its development because if a mammalian limb bud is transplanted to another region of the body or is cultured in vitro, a recognizable limb forms.
A distinctive feature is the presence of a ridge of thickened ectoderm (apical ectodermal ridge [AER]) located along the anteroposterior plane of the apex of the limb bud (Fig. 10.6). During much of the time when the AER is present, the hand-forming and foot-forming regions of the developing limb bud are paddle shaped, with the apical ridge situated along the rim of the paddle (Fig. 10.7). Experiments have shown that the AER interacts with the underlying limb bud mesoderm to promote outgrowth of the developing limb. Other aspects of limb development, such as morphogenesis (the development of form), are guided by information contained in the mesoderm.
Apical Ectodermal Ridge
The earliest limb bud begins to form before an AER is present, but soon a thickened AER appears along the border between dorsal and ventral limb ectoderm. Molecular studies have shown that the position of the AER corresponds exactly to the border between dorsal ectoderm, which expresses the signaling molecule radical fringe, and ventral ectoderm, which expresses the transcription factor Engrailed-1 (En-1) (see Fig. 10.17A).
Although the AER had been recognized for years, its role in limb development was not understood until it was subjected to experimental analysis. Removal of the AER results in an arrest of limb development, thus leading to distal truncation of the limb (Fig. 10.8). In the limbless mutant in chickens, early limb development is normal; later, the AER disappears, and further limb development ceases. If mutant ectoderm is placed over normal limb bud mesoderm, limb development is truncated, whereas combining mutant mesoderm with normal ectoderm results in more normal limb development. These findings suggest that the ectoderm is defective in this mutant.
The power of the AER is shown by experiments or mutants that result in the formation of two AERs on the limb bud. This situation leads to a supernumerary limb, as is illustrated by the mutants eudiplopodia in chickens and diplopodia in humans (Fig. 10.9).
Mesoderm of Early Limb Bud
Structure and Composition
It is impossible to distinguish different cell types within the early limb bud mesenchyme by their morphology alone. Nevertheless, mesenchymal cells from several sources are present (Fig. 10.10). Initially, the limb bud mesenchyme consists exclusively of cells derived from the lateral plate mesoderm. These cells give rise to the skeleton, connective tissue, and some blood vessels. Mesenchymal cells derived from the somites migrate into the limb bud as precursors of muscle and endothelial cells. Another population of migrating cells is that from the neural crest; these cells ultimately form the Schwann cells of the nerves, sensory nerves, and pigment cells (melanocytes).
Mesodermal-Ectodermal Interactions and the Role of Mesoderm in Limb Morphogenesis
Polydactyly is a condition characterized by supernumerary digits and exists as a mutant in birds. Reciprocal transplantation experiments between mesoderm and ectoderm have shown that the defect is inherent in the mesoderm and not the ectoderm. Polydactyly in humans (Fig. 10.11) is typically inherited as a genetic recessive trait and is commonly found in populations such as certain Amish communities in the United States in which the total genetic pool is relatively restricted (see Clinical Correlation 10.1 for further details).
Zone of Polarizing Activity and Morphogenetic Signaling
During experiments investigating programmed cell death in the avian limb bud, researchers grafted mesodermal cells from the posterior base of the avian wing bud into the anterior margin. This manipulation resulted in the formation of a supernumerary wing, which was a mirror image of the normal wing (Fig. 10.12). Much subsequent experimentation has shown that this posterior region, called the zone of polarizing activity (ZPA), acts as a signaling center along the anteroposterior axis of the limb. The signal itself has been shown to be sonic hedgehog (shh) (see Fig. 10.16), a molecule that mediates a wide variety of tissue interactions in the embryo (see Table 4.4). As seen later in this chapter, shh not only organizes tissues along the anteroposterior axis, but also maintains the structure and function of the AER. In the absence of the ZPA or shh, the apical ridge regresses.
Shh induces the expression of the signaling molecule gremlin, which has two inhibitory functions. Gremlin inhibits the action of mesodermal bone morphogenetic protein-2 (BMP-2), which in itself inhibits the expression of FGF-4 in the AER. Such an inhibition of a BMP inhibitor is reminiscent of the sequence of events involved in primary neural induction (see p. 84). In contrast, gremlin, which is localized in the posterior part of the limb bud, inhibits the action of Gli-3 so that Gli-3 functions only in the anterior part. Within the anterior part of the limb bud, Gli-3 inhibits the expression of shh. In Gli-3 mutants, shh becomes expressed ectopically in the anterior limb bud, and preaxial polydactyly results.
Morphogenetic Control of Early Limb Development
Control of Proximodistal Segmentation
As a limb (e.g., an arm) grows out from a simple bud, it eventually forms three structural segments: the stylopodium (upper arm), the zeugopodium (forearm), and the autopodium (hand). Over the years, several hypotheses concerning the control of proximodistal segmentation have been proposed, but only more recently has a hypothesis been supported by strong experimental data. During development, more proximal segments differentiate first, followed successively by the more distal segments. The mesenchymal cells at the distal tip of the limb bud are kept in a proliferative state through the actions of FGFs and Wnts, whereas cells in the proximal part of the limb bud, under the influence of retinoic acid and possibly other molecules, undergo differentiation into proximal components of the limb (Fig. 10.13). The balance between retinoic acid and the FGFs and Wnts is thought to determine the course of segmental differentiation. In the early limb bud, the proximal mesenchymal cells are exposed to a high concentration of retinoic acid because they are near the source (somites), and they differentiate into tissues of the stylopod. As the limb bud grows out, the remaining undifferentiated cells are exposed to a lesser concentration of retinoic acid because outgrowth has taken them farther from the source of retinoic acid. Thus, those remaining mesenchymal cells in later limb buds first differentiate into the zeugopodial segment and finally, in the late limb bud, into the autopodial segment. This balance between the differentiation-promoting effects of retinoic acid and the proliferation-maintaining effects of FGF is similar to that occurring in the posterior end of the early embryo (see Figs. 6-5 and 6.9A).
Cells in the distal mesenchyme are characterized by their expression of Msx-1, a marker of undifferentiated cells, and as they leave that region, expression of that gene ceases (Fig. 10.14A). Something about the distal mesenchymal environment stimulates Msx-1 expression because if mesenchymal cells that have left that region (and consequently cease production of Msx-1) are transplanted back into the distal region, they express that molecule again (Fig. 10.14B).
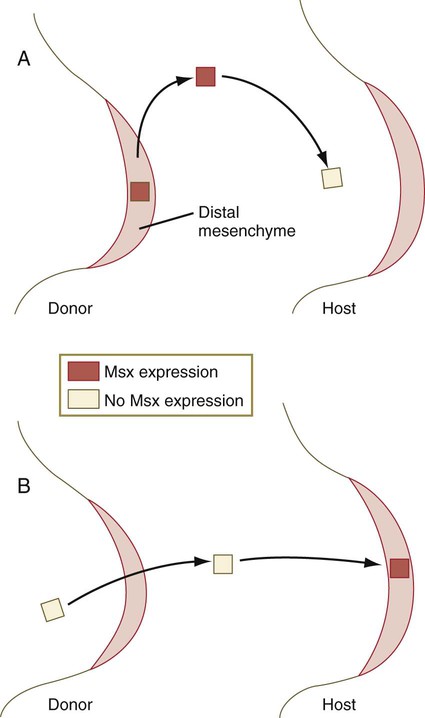
A, If Msx-1–expressing tissue from the distal mesenchyme is transplanted into more proximal regions of the limb bud, it soon stops expressing that molecule. B, If proximal mesenchyme that has already stopped expressing Msx-1 is transplanted back into the distal mesenchymal region, it expresses the molecule again.
Molecular Signals in Limb Development
As discussed earlier, initial development of the limbs involves the establishment of a limb field by the actions of a Hox gene combinatorial code that acts through yet unidentified axial signals to stimulate the expression of Tbx5 in the area of the future forelimb and Tbx4 in the hindlimb. Even in later development, Tbx5 is expressed exclusively in the forelimb, and Tbx4 is expressed exclusively in the hindlimb (Fig. 10.15). Because of these exclusive expression regions, it was originally assumed that these two genes determined the identity of the forelimb and hindlimb. More recent research has shown this not to be the case, however, and the search for the factors that determine limb identity continues. Pitx-1, which is also expressed in the hindlimb, may play a more important role than Tbx-4 as a determinant of hindlimb identity. The main functions of Tbx-4 and Tbx-5 appear to be the initiation of development in a limb-specific manner.
The second major signaling center, this time along the anteroposterior axis, is the ZPA, and the signaling molecule is shh (Fig. 10.16). Although shh is a diffusible molecule, it functions through its effects on BMP-2 and the inhibitor of BMP-2, gremlin (Fig. 10.17). Gremlin has two major functions. First, it antagonizes Gli-3, confining Gli-3 activity to the anterior part of the limb bud, where it represses the expression of posterior limb genes. As mentioned earlier, gremlin also inhibits the inhibitory action of BMP-4 on the AER, thus promoting the activity of FGF-4. FGF-4 is necessary for maintaining the activity of the ZPA.
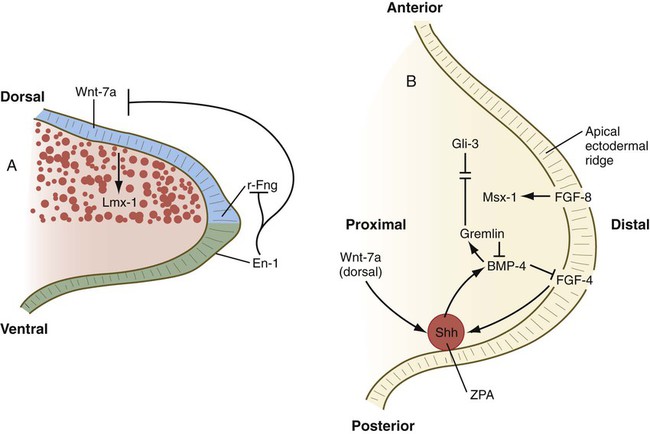
A, Molecular control of the dorsoventral axis. En-1 inhibits Wnt-7a and r-Fng. B, Molecular control along the anteroposterior and proximodistal axes. BMP-2, bone morphogenetic protein-2; En-1, Engrailed-1; FGF, fibroblast growth factor; r-Fng, radical fringe; Shh, sonic hedgehog; ZPA, zone of polarizing activity.
Organization of the dorsoventral axis of the limb begins when the dorsal ectoderm produces the signaling molecule, Wnt-7a, which stimulates the underlying limb bud mesenchyme to express the transcription factor, Lmx-1b, a molecule that imparts a dorsal character to the mesoderm underlying the dorsal ectoderm. Ventral ectoderm produces En-1, which represses the formation of Wnt-7a and consequently the formation of Lmx-1b in what will become ventral limb mesoderm, possibly by a default pathway (see Fig. 10.17A). The AER marks the border between dorsal and ventral limb bud ectoderm, and this border is characterized by an expression boundary between another signaling factor, radical fringe, secreted by dorsal ectoderm, and the En-1 formed in the ventral ectoderm. Further ventral spread of radical fringe expression is held in check by En-1.
All three axial signaling centers (Table 10.1) interact in the early limb bud. Wnt-7a from the dorsal ectoderm has a stimulating effect on the ZPA (see Fig. 10.17B), whereas shh from the ZPA is required for the production of FGFs from the AER that provide additional positive feedback to the ZPA.
Table 10.1
Axial Control in the Developing Limb
Axis | Signaling Center | Molecular Signal |
Proximodistal | Apical ectodermal ridge | FGF-2, FGF-4, FGF-8 |
Anteroposterior | Zone of polarizing activity | Sonic hedgehog |
Dorsoventral | Dorsal ectoderm | Wnt-7a (dorsal) |
Ventral ectoderm | En-1 (ventral) |
Simultaneous with the establishment of the ZPA, an orderly sequence of the homeobox-containing genes Hoxd9 to Hoxd13 (Fig. 10.18) and certain of the Hoxa genes occurs in the early limb bud. This sequence represents a second wave of Hox gene expression after that involved in the initiation of limb development. Shh stimulates the expression of the Hox genes in the limb, and Gli-3 is involved in confining Hox gene expression to the more posterior parts of the limb bud. The Hox genes are involved in patterning the proximodistal axis of the limb (Fig. 10.19). Studies on mice and the analysis of certain human mutants have shown that certain defects in limb regions correspond to absent expression of specific Hox gene paralogues. For example, mutations of Hoxa 13 and Hoxd13 cause characteristic reduction defects of the digits that result from shortness of the phalanges (Fig. 10.20).
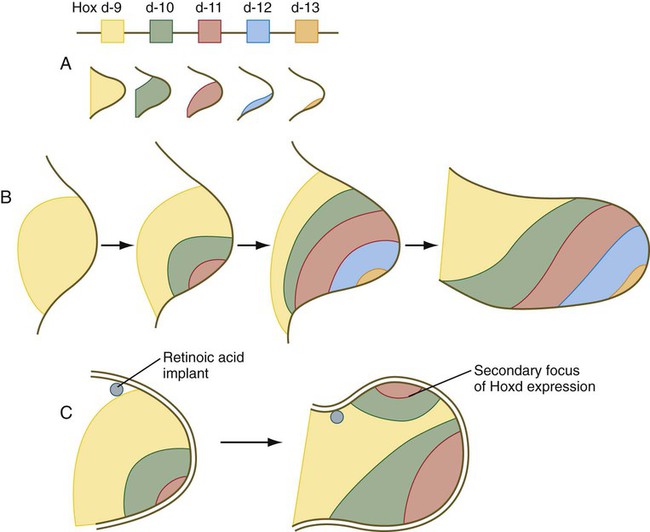
A, Map of this gene family and distribution of individual gene products. B, Development of the aggregate pattern of Hoxd gene expression over time in the normal limb bud. C, The development of a secondary focus of Hoxd gene expression in the area of supernumerary limb formation caused by an implant of retinoic acid. (Based on Tabin CJ: Development 116:289-296, 1992.)
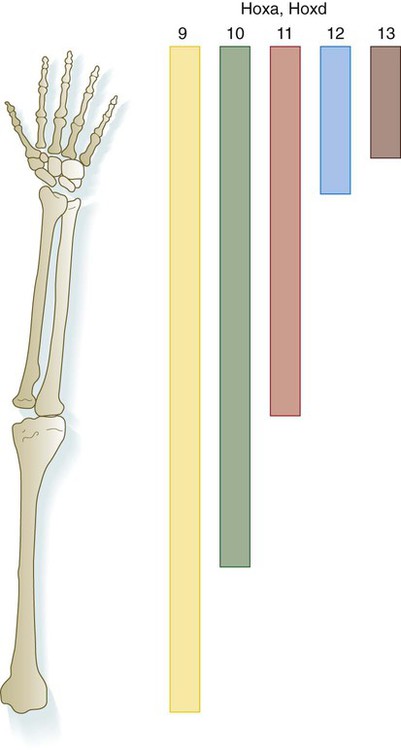
Molecular data from the mouse are superimposed on the human limb skeleton.
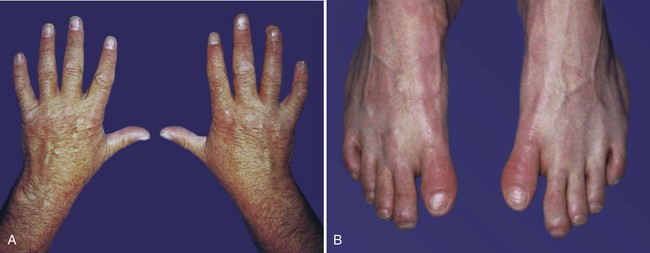
Both thumbs and great toes are more proximally situated than normal. In addition, some phalanges are shortened, and the nails are hypoplastic. (Courtesy of J.W. Innis, Ann Arbor, Mich.)
An interesting, but little explored question in the development of many structures is what causes development to cease. In the case of the limb, the answer may lie in the relationship between shh-producing cells in the ZPA and the expression of gremlin, which depends on exposure to shh. As the limb develops, a zone of cells that had produced shh, but no longer do, forms around the ZPA (Fig. 10.21). These cells are not themselves able to produce gremlin. As more of these cells accumulate, the distance between the shh-producing cells of the ZPA and the cells that can express gremlin increases, ultimately to the point at which these cells no longer receive a sufficient stimulus to produce gremlin. When this happens, the gremlin-based maintenance of FGF-4 production of the AER ceases, and the entire feedback system between the ZPA and AER winds down; this results in cessation of limb development. If the intervening wedge of formerly shh-producing cells is removed from the distal tip of the mature limb bud, the more anteriorly located mesodermal cells are again exposed to above-threshold concentrations of shh, and they can again produce gremlin. This reconstitutes the ZPA-AER axis through a regulative mechanism, and limb development continues past the point at which it usually ceases. The result is the formation of digits with more than the normal number of phalangeal segments.
Cell Death and Development of Digits
Although it may seem paradoxical, genetically programmed cell death (apoptosis) is important in the development of many structures in the body. In the forelimb, it is prominently manifested in the anterior limb margin, in the future axillary region, between the radius and ulna, and in the interdigital spaces (Fig. 10.22). Experiments on avian embryos showed that, to a certain stage, mesodermal cells scheduled to die could be spared by transplanting them to areas in which cell death did not normally occur. After a certain time, however, a “death clock” was set (an example of determination), and the cells could no longer be rescued.
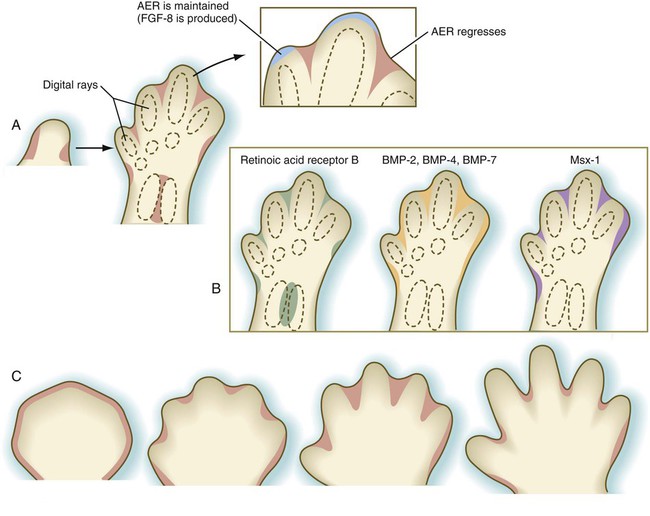
A, Cell death in the chick limb bud. B, Gene expression in zones of cell death of the chick embryo. C, Cell death in the developing human hand. AER, apical ectodermal ridge; BMP, bone morphogenetic protein; FGF-8, fibroblast growth factor-8.
As limb development proceeds, changes become apparent in the AER. Instead of remaining continuous around the entire apex of the limb, the ridge begins to break up, leaving intact segments of thickened ridge epithelium covering the emerging digital rays (cartilaginous models of the digital bones). Between the digits, the ridge regresses (see Fig. 10.22A). As the digital primordia continue to grow outward, cell death sculpts the interdigital spaces (see Fig. 10.22C). BMP-2, BMP-4, and BMP-7 and the transcription factors Msx-1 and Msx-2 are strongly expressed in the interdigital spaces. The exact mechanism of interdigital cell death is still unclear, but the BMPs, especially BMP-4 acting under the mediation of Msx-2, are the prime movers in initiating interdigital cell death. The FGFs produced by the AER seem to play a dual role in interdigital cell death. Although FGF-2 antagonizes the death-inducing effects of the BMPs, FGFs promote the production of Msx-2, which cooperates with the BMPs in inducing interdigital cell death.
If interdigital cell death does not occur, a soft tissue web connects the digits on either side. This is the basis for the development of webbed feet on ducks and the abnormal formation of syndactyly (Fig. 10.23A) in humans. BMP is not found in the interdigital mesoderm in developing duck feet, although it is found in other regions of cell death in the duck limb.
There is more, however, to the development of digits than simply sculpting the interdigital spaces by cell death. Well before cell death becomes evident, other events specify the nature of each digit. A future digit is first recognizable as a longitudinal condensation of mesenchyme, which soon begins to lay down a precartilaginous matrix. The early digital ray then undergoes segmentation (see Fig. 10.26) to form specific phalangeal segments. Each digit develops its own character, as determined by the number of phalangeal segments or its specific size and shape. The underlying basis for the development of digital form is just becoming understood.
All human digits contain three phalangeal segments except for the first digits (thumb and great toe), which consist of only two segments. Rarely, an individual is born with a triphalangeal thumb (see Fig. 10.23B). Why digits have different numbers of phalangeal segments is still not understood.
Development of Limb Tissues
Skeleton
Differentiation of the cartilaginous skeleton occurs in a proximodistal sequence, and in mammals the postaxial structures of the distal limb segments differentiate before the preaxial structures. For example, the sequence of formation of the digits is from the fifth to the first (Fig. 10.24). The postaxial skeleton of the arm is considered to be the humerus, ulna, digits 2 through 5, and their corresponding carpal and metacarpal elements. The preaxial portion of the limb bud becomes progressively reduced during limb outgrowth and contributes only to the radius and possibly the first digital ray. Certain limb defects, sometimes called hemimelias, are characterized by deficiencies of preaxial or postaxial limb components (Fig. 10.25).
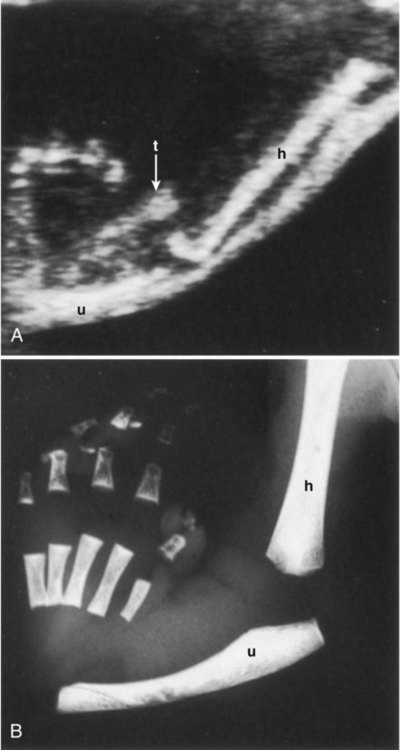
A, Ultrasound image showing a thumb (t, arrow) but no radius. B, Postnatal x-ray image confirming the absent radius. h, humerus; u, ulna. (From Nyberg D and others: Diagnostic ultrasound of fetal anomalies, St. Louis, 1990, Mosby.)
A characteristic feature in differentiation of the limb skeleton is the formation of joints. Joint formation occurs by the transverse splitting of precartilaginous rods, rather than by the apposition of two separate skeletal elements. Joint formation is first apparent when transverse strips of highly condensed cells cross a precartilaginous rod (Fig. 10.26). Formation of the zone of cell density is induced by Wnt-14, which stimulates the formation of growth differentiation factor-5, a member of the BMP family, in the region of the future joint. BMP activity, which is strongly involved in cartilage formation, must be excluded from the region of the developing joint. Noggin, an antagonist of BMP, plays an important role in joint formation because in its absence, BMP is expressed throughout the region where the joint should form, and the digital rays develop into solid rods of cartilage without joints. The roles of noggin and BMP in joint formation are very similar to those seen in the formation of the sutures between the cranial bones (see p. 177).
Musculature
A later function of the T-box transcription factors Tbx-5 and Tbx-4, which play earlier important roles in initiating the development of the forelimbs and hindlimbs, respectively (see p. 193), is the regulation of muscle patterning. Mutations of these genes result in abnormal limb muscle patterning.
Although limb muscles assume their definitive form in the very early embryo, they must undergo considerable growth in length and cross-sectional area to keep up with the overall growth of the embryo. This growth is accomplished by the division of the satellite cells (see p. 183) and the fusion of their progeny with the muscle fibers. The added satellite cell nuclei increase the potential of the muscle fiber to produce structural and contractile proteins, which increase the cross-sectional area of each muscle fiber. Accompanying this addition to the nuclear complement of the muscle fibers is their lengthening by the addition of more sarcomeres, usually at the ends of the muscle fibers. The formation of new muscle fibers typically ceases at or shortly after birth. Although the muscles are capable of contracting in the early fetal period, their physiological properties continue to mature until after birth.
Innervation
Motor axons originating in the spinal cord enter the limb bud at an early stage of development (during the fifth week) and begin to grow into the dorsal and ventral muscle masses before these masses have split up into primordia of individual muscles (Fig. 10.27). Tracing studies have shown a high degree of order in the projection of motoneurons into the limb. Neurons located in medial positions in the spinal cord send axons to the ventral muscle mass, whereas neurons located more laterally in the spinal cord supply the dorsal muscle mass. Similarly, a correlation exists between the craniocaudal position of neurons in the cord with the anteroposterior pattern of innervation of limb muscles within the common muscle masses. For example, the rostralmost neurons innervate the most anterior muscle primordia.
Local cues at the base of the limb bud guide the entering pathways of nerve fibers into the limb bud. If a segment of the spinal cord opposite the area of limb bud outgrowth is reversed in the craniocaudal direction, the motoneurons change the direction of their outgrowth and enter the limb bud in their normal positions (Fig. 10.28). If larger segments of spinal cord are reversed, and the neurons are at considerable distances from the level of the limb bud, their axons do not find their way to their normal locations in the limb bud. The muscles themselves apparently do not provide specific target cues to the ingrowing axons because if muscle primordia are prevented from forming, the main patterns of innervation in the limb are still normal.
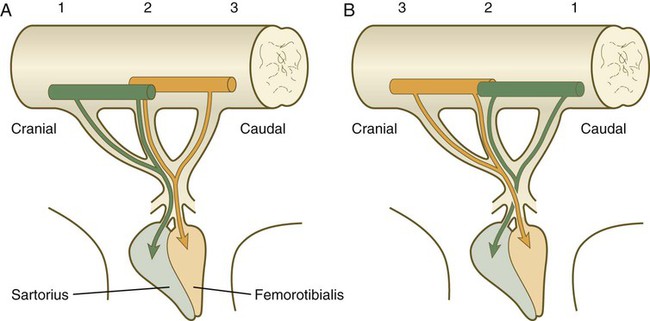
A, Normal limb. B, After reversal of three segments of the embryonic spinal cord, axons originating from the spinal cord pass through abnormal pathways to innervate the muscles that they were originally destined to innervate. (Adapted from Brown M and others: Essentials of neural development, Cambridge, 1990, Cambridge University Press.)
Vasculature
The earliest vasculature of the limb bud is derived from endothelial cells arising from several segmental branches of the aorta and the cardinal veins and, to some extent, from angioblasts (endothelial cell precursors) arising from the somites or endogenous to the limb bud mesoderm. Initially, the limb vasculature consists of a fine capillary network, but soon, some channels are preferentially enlarged, resulting in a large central artery that supplies blood to the limb bud (Fig. 10.29). From the central artery, the blood is distributed to the periphery via a mesh of capillaries and then collects into a marginal sinus, which is located beneath the AER. Blood in the marginal sinus drains into peripheral venous channels, which carry it away from the limb bud.
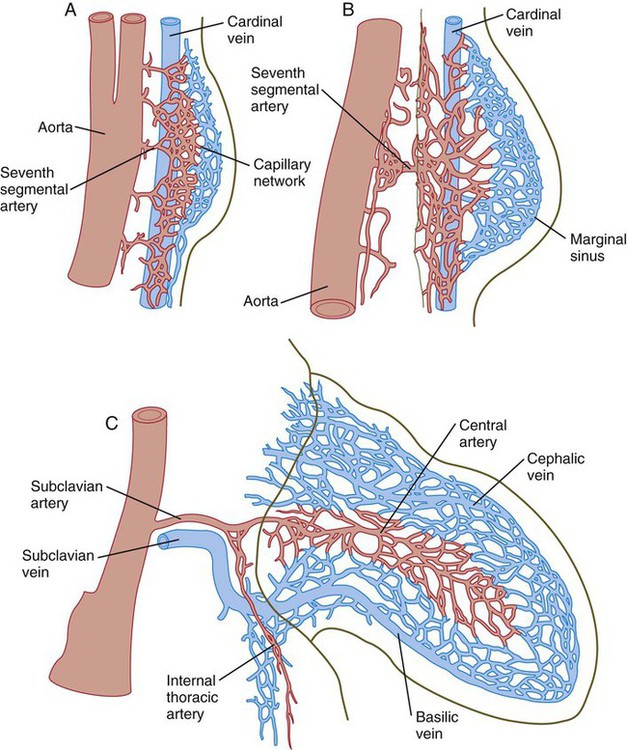
A, Equivalent of a 4-week human embryo. B, Equivalent of a 5-week human embryo. C, Equivalent of a 6-week human embryo.
Even in the earliest limb bud there is a peripheral avascular zone of mesoderm within about 100 µm of the ectoderm of the limb bud (Fig. 10.30A). The avascular region persists until the digits have begun to form. Angioblasts are present in the avascular zone, but they are isolated from the functional capillaries. Experimental studies have shown that the proximity of ectoderm is inhibitory to vasculogenesis in the limb bud mesoderm. If the ectoderm is removed, vascular channels form to the surface of the limb bud mesoderm, and if a piece of ectoderm is placed into the deep limb mesoderm, an avascular zone forms around it (Fig. 10.30B). Degradation products of hyaluronic acid, which is secreted by the ectoderm, seem to be the inhibitory agents.
The pattern of the main vascular channels changes constantly as the limb develops, probably from the expansion of preferred channels within the capillary network that perfuses the distal part of the developing limb. With the establishment of the digital rays, the apical portion of the marginal sinus breaks up, but the proximal channels of the marginal sinus persist into adulthood as the basilic and cephalic veins of the arm (see Fig. 10.29C).
Similar major changes occur in the arterial channels that course through the developing limb (Fig. 10.31). Preferred channels connected to the primary axial artery ultimately take ascendancy, especially in the forearm, thus leaving the original primary axial artery a relatively minor vessel (the interosseous artery) in the forearm.
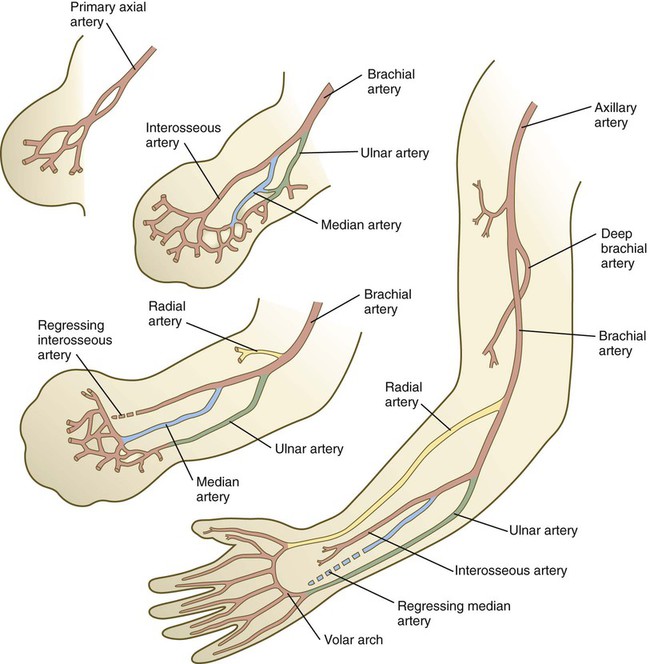
Clinical Correlation 10.1 discusses limb anomalies.
Summary
Limbs arise from the lateral mesoderm and the overlying ectoderm under the influence of an inductor working through the Tbx transcription factors. The early limb bud is a highly regulative system that can compensate for a variety of surgical disturbances and still form a normal limb. The axes of the limb are fixed in an anteroposterior, dorsoventral, and proximodistal sequence.
The early limb bud mesoderm stimulates the overlying ectoderm to form an AER that stimulates the outgrowth of the limb through proliferation of the underlying mesodermal cells. FGF-4 and FGF-8 are secreted by the apical ridge and can induce outgrowth in the absence of the ridge. The overall morphogenesis of the limb is determined by properties of the mesoderm, whereas the ectoderm acts in a more permissive sense.
Cell death is an important mechanism in normal limb development. Regions of programmed cell death include the axillary region and the interdigital spaces. In the absence of interdigital cell death, syndactyly results.
A ZPA located in the posterior mesoderm acts as a biological signaler and plays an important role in the anteroposterior organization of the limb by releasing shh. Wnt-7a, which is released by dorsal ectoderm, is the organizer of dorsoventrality in the limb bud. According to the concept of positional information, cells in the developing limb are exposed to positional cues (e.g., the signal from the ZPA) that allow them to determine their relative position within the limb bud. The cells process this information and differentiate accordingly. Proximodistal control of morphogenesis may reside in the progress zone, a narrow band of mesoderm beneath the AER.
Digits 2 through 5 form on the basis of their increasing exposure to shh (time and concentration), whereas the formation of the first digit is independent of shh.
Retinoic acid exerts a profound effect on limb morphogenesis and can cause the formation of a supernumerary limb if applied to the anterior border of the limb bud, but its exact role in limb development remains obscure. Expression of a variety of homeobox-containing genes follows well-defined patterns in the normally developing limb. Some patterns of gene expression are profoundly altered in limbs treated with retinoic acid.
The skeleton of the limb arises from lateral plate mesoderm. The ectoderm of the limb bud inhibits cartilage formation in the mesoderm cells immediately beneath it. This could explain the reason the skeleton of the vertebrate limb forms in a central position.
Limb muscles arise from cells derived from somitic mesoderm. These cells express Pax-3 during their migration into the limb bud. Myogenic cells first form dorsal and ventral common muscle masses, which later split into primordia of individual muscles. Morphogenetic control of muscles resides in the associated connective tissue, rather than in the muscle cells themselves. Later stages in muscle development may involve cell death, the fusion of muscle primordia, and the displacement of muscle primordia to other areas.
Tendons arise from lateral plate mesoderm and initially form independently of the muscles. Later differentiation of tendons requires interactions with contracting muscles.
Nerves grow into the developing limb bud and become associated with the common muscle masses as they split into individual muscles. Local cues are important in guiding growing axons into the developing limb.
The vasculature of the limb bud arises from cells budding off the aorta and cardinal veins and from endogenous mesodermal cells. The early vascular pattern consists of a central artery, which drains into a peripheral marginal sinus and then into peripheral venous channels. Blood vessels do not form beneath the ectoderm or in the central cartilage-forming regions.
Limb anomalies can form as the result of genetic mutations, drug effects, disturbed tissue interactions, and purely mechanical effects.
*Because of different conventions in the use of axial terms, some human embryologists would take exception to the axial terminology presented here. Specifically, according to strict human terminology, anterior means “ventral,” and posterior means “dorsal.” However, the axial terminology used in this chapter (anterior means “cranial,” and posterior means “caudal”) is so uniformly used in the experimental and comparative embryological literature that a student referring to the original literature in the field of limb development would find it confusing to use human axial terminology.