Chapter 19 Introduction to the Regulation of Cardiovascular Function
Abbreviations | |
---|---|
ACh | Acetylcholine |
ANS | Autonomic nervous system |
CNS | Central nervous system |
Epi | Epinephrine |
NE | Norepinephrine |
NPY | Neuropeptide Y |
Dysfunction of the cardiovascular system is the principal cause of death and disability in middle-aged and elderly men and women in the industrialized world. In the United States in 2004, there were nearly 1 million deaths from cardiovascular disease, representing approximately 36% of all deaths. In addition, estimates of the prevalence of cardiovascular disease in 2005 indicated that more than 70 million individuals had hypertension, 16 million had coronary heart disease, and more than 5 million had congestive heart failure (Table 19-1). To best understand pharmacological approaches to the management of these disorders, an overview of the regulation of cardiovascular function is warranted.
TABLE 19–1 Prevalence of Cardiovascular Disease in the United States in 2005*
Hypertension | 73 |
Coronary heart disease | 16 |
Myocardial infarction | 8.1 |
Angina pectoris | 9.1 |
Stroke | 5.8 |
Congestive heart failure | 5.3 |
* Data from the American Heart Association; numbers represent millions of persons.
The ANS innervates the heart, blood vessels, kidney, and adrenal medulla and has the potential to modify cardiovascular function in a number of different ways (see Chapter 9).
The overall coordination and integration of organismal cardiovascular function is accomplished primarily by the ANS. Through its sympathetic and parasympathetic limbs, the ANS has powerful effects on both cardiac performance and blood vessel caliber (see Chapter 9).
The sympathetic and parasympathetic nerves innervating cardiovascular end organs are tonically active, which means that activity can be modulated by either increasing or decreasing the firing rate of these nerves. Effects of autonomic nerve activity on the mechanisms that control blood pressure are summarized in Figure 19-1. Parasympathetic effects are mediated by acetylcholine (ACh) released from postganglionic parasympathetic nerve endings, whereas sympathetic effects are mediated by norepinephrine (NE) released from postganglionic sympathetic nerve endings. Although there is no circulating ACh because of high cholinesterase activity in both tissue and blood, NE released from postganglionic sympathetic nerve endings escapes into the circulation because its degradation or reuptake is incomplete. This source of NE, in concert with the epinephrine (Epi) and NE released into the blood from the adrenal medulla, influence cardiovascular function as circulating neurohormones (see Chapter 9).
Ventricular contractile force is little influenced by parasympathetic activity but can be greatly increased by sympathetic activity, including the actions of circulating Epi and NE. Increased sympathetic activity reduces vascular caliber by contracting vascular smooth muscle. Although there are parasympathetic influences on a few vascular beds, their contribution to overall vascular resistance is insignificant. Constriction of veins in response to sympathetic activity reduces venous capacitance, thereby increasing venous return to the heart, which augments atrial and ventricular filling, resulting in increased cardiac output. Sympathetically mediated constriction of arterioles can reduce cardiac output by increasing the resistance against which the heart must pump blood. In addition, elevated sympathetic activity to the kidney increases renin release and subsequent angiotensin II formation and causes causing Na+ and H2O retention. All of these effects act in concert to elevate arterial blood pressure. Conversely, a reduction of sympathetic activity reduces blood pressure by removing the sympathetic stimulus. The receptors and signaling pathways involved are discussed in Chapter 9.
CENTRAL CONTROL OF AUTONOMIC NERVE ACTIVITY
The organization of autonomic cardiovascular control systems within the central nervous system (CNS) is summarized in Figure 19-2. The final common (preganglionic) output neurons for cardiovascular control by the parasympathetic nervous system are located principally in the nucleus ambiguus of the brainstem. The preganglionic neurons of the sympathetic nervous system are located in the intermediolateral columns of the thoracolumbar region of the spinal cord. Antecedent to these final output neurons, much of the integration of neural signals contributing to autonomic regulation of cardiovascular function occurs at other sites in the brainstem. These neurons, in turn, receive input from all levels of the CNS, some of the more important of which are diagrammed in Figure 19-2.
Origin and Regulation of Autonomic Activity
One of the principal roles of the ANS is to provide adaptive regulation and coordination of blood pressure and flow to various organs of the body in the face of an ever-changing internal environment. This is accomplished by neural circuits intrinsic to the CNS and as a response to mechanical and humoral signals originating in the periphery. Integration of these signals by the CNS produces patterns of autonomic activity that ensure adequate organ perfusion appropriate to such diverse demands as changes in posture, hemorrhage, digestion, and exercise.
Other visceral mechanosensitive and chemosensitive nerve terminals are located throughout the body. Some of these bipolar neurons, with cell bodies in the dorsal root ganglia, may initially commingle their axons within various sympathetic nerve trunks (sympathetic afferents) before synapsing on cells located in the dorsal horns of the spinal cord. Other sensory afferents do not travel with the sympathetic nerves and, instead, associate with various sensory-motor nerve trunks (somatic afferents) before synapsing in the spinal dorsal horns. All of these afferents typically encode noxious or painful chemical or mechanical stimuli, such as those associated with cardiac or visceral ischemia, visceral organ distension, or injury, and detect the metabolic products produced by exercising skeletal muscle. Activation of these afferents typically produces sympathoexcitation.
In addition to neural signals from the periphery, the brain also detects chemical signals (including drugs, such as digitalis) that circulate in the blood. A wide variety of circulating humoral substances, including catecholamines, indoleamines, and peptides, directly contact neurons within the CNS by diffusing through the fenestrated capillaries of circumventricular organs that lack a blood-brain barrier (see Fig. 19-2). Activation of circumventricular organ neurons produces integrated autonomic, endocrine, and behavioral responses that can regulate salt and H2O balance and nutrient homeostasis, in addition to cardiovascular function. The most important of the circumventricular organs for central autonomic control are the area postrema, subfornical organ, and organum vasculosum of the lamina terminalis.
Minute-to-minute control of arterial blood pressure is achieved when small pressure changes are linked to reflex alterations in autonomic nerve activity. Sensory nerve endings embedded in the wall of the carotid sinus and aortic arch (baroreceptors) are activated by wall stretch when arterial pressure increases. This leads within a few seconds to an increase in vagal (parasympathetic) activity and a reduction in sympathetic activity. Parasympathetic activation slows heart rate, and sympathetic inhibition results in passive vasodilation, thus tending to return arterial pressure toward the original level. Conversely, a decrease in arterial pressure is rapidly countered by increased sympathetic and decreased parasympathetic activity. This results in vasoconstriction and an elevated cardiac rate and force of cardiac contraction. Organization of the baroreceptor reflex is illustrated in Figure 19-3.
The influence of baroreceptors on sympathetic nerve activity can vary greatly in different vascular beds. Some beds, such as the cutaneous vasculature, are largely independent of arterial baroreceptor influence and contribute little to total peripheral vascular resistance. In contrast, the baroreceptor reflex predominates in controlling sympathetic regulation of vascular caliber in many organs that receive a significant fraction of the cardiac output, such as skeletal muscle and kidney. For this reason baroreflex regulation of sympathetic vasoconstriction plays an important role in determining total peripheral resistance. In fact, except under some special circumstances (exercise, sleep, and certain behavioral states), baroreceptors are able to override all other inputs affecting autonomic regulation of arterial blood pressure. This may reflect the importance of maintaining a stable systemic blood pressure to ensure adequate organ perfusion under diverse environmental conditions.
REGULATION OF SYMPATHETIC ACTIVITY
Under physiological conditions, the amount of NE released is influenced by various chemicals, some of which are coreleased, such as neuropeptide Y and adenosine triphosphate, whereas others are released from postjunctional tissues, including angiotensin II, or are present in the circulation such as Epi (Table 19-2). Endogenous compounds that alter Ca++, Na+, or K+ channel activity lead to alterations in vesicular NE release. In addition, the released transmitter itself, acting at prejunctional autoreceptors, and other transmitters or hormones acting at prejunctional heteroreceptors can affect NE release. Activation of prejunctional receptors modulates the probability that individual vesicles will discharge their contents by exocytosis congruent with depolarization; it does not affect the amount of transmitter released by individual vesicles. Activation of inhibitory autoreceptors by NE may function as a physiological brake on transmitter secretion during periods of high-frequency nerve discharge, thus limiting postjunctional responses. Agonists at heteroreceptors facilitating transmitter release such as angiotensin II amplify the effects of sympathetic nerve depolarization. In contrast, activation of inhibitory heteroreceptors, such as occurs with adenosine, reduces the probability of vesicular exocytosis and transmitter release. Some of these mechanisms are illustrated in Figure 19-4. It is important to remember that because local mechanisms regulating NE release differ in different tissues, similar rates of sympathetic nerve firing may produce different effects in different tissues.
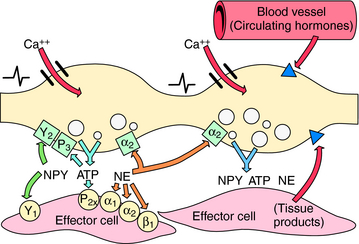
FIGURE 19–4 Prejunctional regulation at the sympathetic neuroeffector junction. The left varicosity illustrates autoinhibition of neurotransmitter release, including possible “lateral” inhibition (i.e., transmitter from one varicosity inhibiting release from an adjacent varicosity). The right varicosity illustrates prejunctional regulation of transmitter release by tissue and blood-borne chemicals. See Table 19-2 for a list of involved substances. Postjunctional receptors are shown as circles, ; prejunctional inhibitory autoreceptors are shown as squares,
; prejunctional heteroreceptors are shown as triangles,
.
Some of the factors proposed to play a causative role in hypertension as a consequence of increased sympathetic activity are listed in Box 19-1.
Pang CC. Autonomic control of the venous system in health and disease: effects of drugs. Pharmacol Ther. 2001;90:179-230.
Robertson D, Biaggioni I, Burnstock G, Low PA. Primer on the autonomic nervous system. New York: Elsevier, 2004.
Sved AF, Ito S, Sved JC. Brainstem mechanisms of hypertension: Role of the rostral ventrolateral medulla. Curr Hypertens Rep. 2003;5:262-268.
For information specific to cardiovascular diseases, see http://www.Americanheart.org.