Chapter 43 Interbody Cages
Anterior cervical discectomy and cervical corpectomy for decompression of degenerative disease, trauma, tumor, and infection are commonly performed spinal operations. Options for reconstruction of the ventral column include structural autografts and allografts, as well as an evolving genre of prosthetic devices. The objectives of reconstruction are to restore a stable load-bearing ventral column, to maintain intervertebral height, and to establish an anatomic cervical lordosis when possible. The ultimate goal is for the construct to become biologically integrated into the native spine and to be replaced by living bone over time. For simple discectomy and single-level corpectomy, these end points are usually achievable with tricortical autografts harvested from the iliac crest or commercially available prefabricated cadaveric bone grafts. Complications such as graft collapse or extrusion are occasionally encountered. The primary objection to the use of structural autografts, however, is the relatively high rate of morbidity at the donor site from chronic pain, numbness, infection, hematoma, and cosmetic deformity. Such complications are reported to occur in 10% to 25% of cases.1–4 A common observation among spine surgeons is that the morbidity associated with the bone graft harvest frequently exceeds the morbidity related to the primary procedure. Pain at the bone graft harvest site has traditionally been reported by patients to be worse than that at the primary surgical site. This high rate of donor site morbidity has spurred the search for alternative reconstructive possibilities. Cadaveric prefabricated allografts have gained popularity over the past several years because of concerns over this donor site morbidity. Recently, several authors have advocated use of donor site alternatives to iliac crest (e.g., manubrium)5; however, long-term follow-up after using such an alternative is lacking. Structural allografts eliminate donor site problems but are associated with a slightly increased risk of pseudarthrosis and graft resorption, especially in smokers and diabetics. Although highly processed, lingering patient concerns remain about potential risk for disease transmission from allografts.
Reconstruction of a multilevel corpectomy bed poses yet a greater challenge. In fact, in a recent study by Uribe et al., the rate of early hardware failure and pseudarthrosis was higher after cervical corpectomy than after anterior cervical discectomy, suggesting that in the absence of specific pathology requiring removal of a vertebral body, multilevel anterior cervical discectomy and fusion (ACDF) using interbody cages and autologous bone graft could result in lower morbidity.6 Iliac crest and fibula are the best options available for autograft, but harvest of suitably long struts contributes significantly to patient morbidity. It can also be very difficult to match the graft to the cross-sectional and longitudinal geometry of the recipient site. These obstacles have prompted many surgeons to substitute fibular allograft as a simpler expedient. Fibular allografts are very straight and have a much smaller diameter than does material from the cervical corpus. These features enable it to fit readily into the recipient bed, but may not indicate optimal load transfer characteristics. Fibular allograft is composed almost exclusively of hard cortical bone. The mismatch in cross-sectional diameters and physical characteristics between the graft and recipient bone contributes to “pistoning” of the fibular strut through the adjacent central end plates, a condition commonly observed as the reconstructed segment foreshortens during graft incorporation, with resultant loss of lordosis.7 An additional concern is the heightened risk of nonunion that accompanies long-segment allograft constructs.
Interbody Cages
A variety of prosthetic interbody cages are now available for use in the cervical spine, both for disc space arthrodesis and to bridge the larger voids created by single- or multilevel corpectomy. Current devices are fabricated, either from titanium alloy or polymer, and can be classified into four categories: (1) screw-in, (2) box-type, (3) interbody fusion cage with integrated ventral fixation, or (4) a cylindrical design.8,9 Devices approved by the U.S. Food and Drug Administration (FDA) include hydroxyapatite spacer grafts, bioabsorbable implants, and artificial discs; they are now widely used and are not the primary focus of this chapter.
Interbody cages are intended to confer immediate structural integrity to the ventral spine and to provide instant support through the instantaneous axis of rotation. Although some surgeons have placed these as naked implants,10 more typically, they are employed as carriers for osteoinductive or osteoconductive materials whose purpose is to secure long-term stability through biologic integration with the recipient spine. In our experience, interbody cages are usually loaded with morselized autograft obtained from the cervical spine itself or from cancellous harvest from the sternum or iliac crest with negligible added morbidity. Alternatives such as allograft bone, hydroxyapatite, and “biologics,” including recombinant human bone morphogenetic protein (rhBMP), may even obviate this need.
Devices designed for disc space arthrodesis come prefabricated in a variety of sizes for direct implantation. Cages used for vertebral body replacement are provided as stock material, which can be rapidly modified to match an individual patient’s unique anatomic need. Many of these implants remain in development and have not been released by the FDA for clinical application in the United States at this time. The literature that bears on these devices includes animal-testing data,11 biomechanical studies,8,12,13 and clinical reports2,10,14–29; it is comparatively sparse and is devoid of any class 1 evidentiary material.
Screw-in Devices
The Bagby and Kuslich cervical cage (BAK/C, Sulzer Spine-Tech, Minneapolis, MN) is the prototypic example of a screw-in design. The BAK/C cage is fundamentally an adaptation of a spinal instrumentation system already validated for disc space arthrodesis in the lumbar spine. After successful completion of a prospective randomized multicenter trial, it received FDA approval for use in the cervical spine.20 Similar to the larger lumbar devices, a BAK/C implant consists of a hollow, threaded cage with multiple side-wall fenestrations. The cages are manufactured from a titanium alloy and are provided in a 12-mm length, with a choice of 6-, 8-, 10-, and 12-mm diameters. Using a modification of the Cloward technique,30 it is usually inserted as a single, midline cage after reaming and tapping of the adjacent central end plates (Fig. 43-1). Alternatively, two smaller (6- or 8-mm) cages may be applied in a side-by-side fashion, although this necessarily limits the height of disc space distraction that can be achieved (Fig. 43-2). The cages are loaded with locally derived bone shavings, sometimes supplemented with allograft, according to volumetric needs. Sofamor Danek (Memphis, TN) has recently released the Affinity cage in North America. This is similar in concept to the BAK/C, but has a conically tapered configuration whose purpose is to help establish cervical lordosis.
In the BAK/C study a single-level “fusion rate” of nearly 100% was reported. No device-related failures or complications occurred, and other measures of clinical outcome appeared comparable to the noninstrumented ACDF “control” patients.20 Furthermore these outcomes were achieved without the morbidity associated with iliac crest bone graft harvest observed in the control group. However, certain concerns linger about the consequences of violating the central vertebral end plates, as is required for insertion of this device. Hacker observed a 20% incidence of postoperative “sagittal alignment abnormalities” at follow-up.2 Lordotic reaming and tapping techniques and a tapered expansile cage (Varilift, Advanced Spine, Irvine, CA) have been developed in an effort to address this issue.
Because screw-in cages have a cylindrical cross section, the contact surface available for load transfer (and for fusion) is a comparatively narrow trough whose width is less than half of the cage diameter. This difference is mechanically disadvantageous in resisting lateral flexion, and the potential for subsidence of the implant with concomitant loss of cervical lordosis may be magnified if two or more adjacent segments are instrumented. Disc space restoration to an average height of 6 to 8 mm requires implantation of 8- to 10-mm diameter cages. When this is attempted at two or more adjacent levels, the resultant encroachment into the intervening body can be substantial and may necessitate offset placement of the devices and result in significant subsidence (Fig. 43-3). When Wang et al.31 studied 64 patients who underwent ventral decompression and interbody fusion with BAK/C, they found that the ventral height of intervertebral space decreased significantly after 1 year, when compared with the ventral height immediately after surgery. BAK/C subsidence was observed in nine patients, including five with single-level fusion, one with two-separated-level fusion, and three with double-adjacent-level fusion, according to the standard of loss of intervertebral height of more than 3 mm. BAK/C fusion was generally effective; however, neck pain tended to reoccur in the patients with cage subsidence and two of them needed revision surgery because of the recurrence of myelopathy with progressive neck pain. Although BAK/C was generally effective, the pitfalls in the design of the device have resulted in the observation of clinical subsidence, which, in some cases, required reoperation for the recurrence of symptoms.
Box Cages
In Smith-Robinson–type interbody fusions, the bony end plates are left intact, and a block-shaped structural graft with large, flat, superior and inferior contact surfaces is countersunk into the disc space.32 From a biomechanical standpoint this construct is superior because it retains the integrity of the end plates and provides a large surface area for load transfer and arthrodesis. Several box-type implant designs have been explored as alternatives to the more traditional tricortical autograft or structural allografts for Smith-Robinson fusion. These share a more or less rectangular configuration with a hollow core and fenestrations in their superior and inferior surfaces to allow the through growth of bone. Both titanium and polymer cages have been produced. The Rabea cage (Signus Medizintechnik, Alzenau, Germany), the Syncage (Synthes, Davos, Switzerland), and the Tibon cage (Biomet-Merck, Berlin, Germany) are representative of titanium box designs.
With FDA approval, the Rabea cage is currently available as a “cement restrictor.” It is made of forged titanium to ensure MRI compatibility and is prefabricated in a 12-mm width, 12- and 14-mm depths, and in heights ranging from 4 to 8 mm (Fig. 43-4). The Rabea is offered as a parallel end-plate design and as a “lordosed” version, with 5 degrees of divergence built in. The rostral and caudal surfaces also bear retentive serrations to engage the end plates and resist implant extrusion during neck flexion. Published experience with the Rabea cage is limited, but preliminary biomechanical studies and clinical reports are both favorable.10,12,15 As with other metallic devices, it is hard to judge fusion according to criteria of radiographically demonstrable bridging bone across the disc space and end plates. Alternative criteria including less than 2 degrees of angulation in dynamic radiographs and absence of any peri-implant bony lucency are substituted.
Carbon fiber box cages have found favor as intervertebral implants in the lumbar spine.33 These devices appear to be largely inert biologically; they exhibit good strength in all axes of applied stress, they are impact and fatigue resistant, their elastic modulus is purported to match well with that of the cortical bone of the recipient spine, and because they are radiolucent, they afford an opportunity for direct graft visualization and fusion assessment. Extending these advantages to a cervical interbody application, box-type polymer cages have been under active development abroad and are poised to enter the U.S. market. Most designs have been fabricated from polyetheretherketone (PEEK), some also including a carbon fiber component (Cervical IF Cage, DePuy AcroMed, Raynham, MA). The Rabea device is now offered as a PEEK cage, again with approval in the United States for use as a cement restrictor. Other major vendors, including Stryker Instruments (Kalamazoo, MI [Solis PEEK cage]), Medtronic Sofamor Danek (CornerStone-SR), and Synthes (CR), have polymer cages already in place in overseas markets (Fig. 43-5). Like the Rabea cage, they are offered in a variety of sizes and configurations, including parallel end-plates, lordotic, and superiorly convex designs to conform to individual disc space anatomic requirements. Shared features include a large central opening to contain graft material, ridged end plates to resist implant extrusion, and imbedded radiopaque markers to allow radiographic visualization of the device.
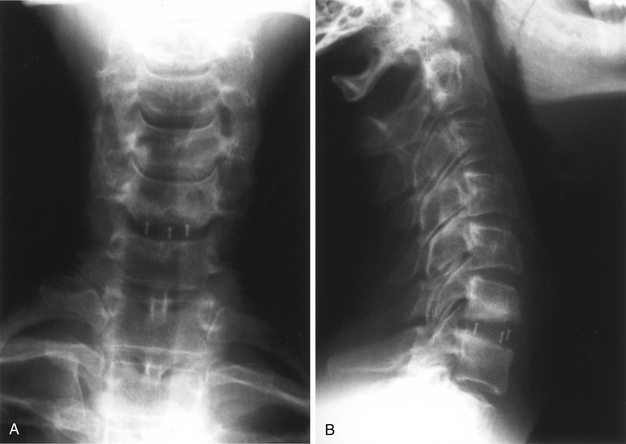
FIGURE 43-5 A, Single-level Synthes CR cage, anteroposterior view. B, Single-level Synthes CR cage, lateral view.
Cho et al.18 have recently reported on their preliminary experience with the Solis PEEK cage loaded with cancellous autograft in 40 patients undergoing mostly one- and two-level cervical discectomy and fusion.18 Although the term of follow-up is unclear, the authors reported good functional outcomes, no device-related complications or failures, increased cervical lordosis, enlargement of neural foraminal cross-sectional area, and a 100% fusion rate. The complication rate was 2.5% in PEEK cage patients compared with a rate of 17.5% in a concurrent control group undergoing conventional discectomy and structural autografting in whom problems with graft collapse, dislodgement, and donor site morbidity were encountered. Other reports34 have found a high subsidence tendency of box cages into predominantly C7; this illustrates the need for a modified box cage design that improves and extends contact with the inferior surface. Radiolucent polymer cages are easily inserted; they appear to be biomechanically sound, and they hold much promise as interbody prostheses in the cervical spine, pending the outcome of more comprehensive clinical studies.
Interbody Fusion Cages with Intergrated Ventral Fixation
Recently, newly developed interbody spacers with integrated ventral fixation components have been gaining popularity in clinical use.9 The low profile of these devices allows the surgeon to treat ventral pathology without having to disrupt indwelling hardware. An example of the clinical utility of such a device is an adjacent-level disc herniation after an anterior cervical discectomy. These devices can often be inserted at the adjacent site, after the discetomy is performed, without the need to disrupt the indwelling ventral cervical plate at the previously fused adjacent level. The anchored spacer provides similar biomechanical stability to that of the established ventral fusion technique and has potentially lower associated perioperative and postoperative morbidity, such as dysphagia or swallowing difficulties.9 Two of these FDA-approved devices are the ROI-C VerteBRIDGE (LDR Inc., Austin, TX) interbody device and the Mosaic cervical implant system (Spinal Elements, Inc., Carlsbad, CA). See Figure 43-6. To date, more than 2500 of these devices have been used in the United States. Studies on the long-term efficacy of these devices are in progress.
Cylindrical Mesh Cages
There is a paucity of literature pertaining to the use of mesh cages for cervical disc space arthrodesis.19,23 Our own experience with these devices in single- and multilevel discectomy operations suggests a significant potential for subsidence when these devices are used in a stand-alone mode. We attribute this to the relatively small contact area at the bone-implant interface in small-diameter disc space cages, despite the use of end caps.23 To retard subsidence, we came to augment almost all ventral mesh cage reconstructions for degenerative disc disease with a dynamic ventral cervical plate. Although the addition of a ventral plate contributes only minimally to the duration and risk of operation, it does constitute a significant added expense. It is hoped that some of the newer box cage designs may yield satisfactory clinical results without a need for supplemental ventral fixation.
Mesh Cage Reconstruction of Corpectomy Defects
A more distinct advantage of using cylindrical mesh cage constructs is in the reconstruction of corpectomy defects. Indications for cervical corpectomy can include spondylosis, ossification of posterior longitudinal ligament, trauma, tumor, deformity correction, and occasional cases of infection. We and others have come to favor it in treatment of adjacent segment cervical disc disease as well.21,24 Removal of a cervical corpus and the adjoining discs, and any associated osteophytes, can frequently be accomplished more expeditiously than two separate microdiscectomies (Fig. 43-7). The quality of the decompression is unsurpassed, and ample bone is acquired from the corpectomy material to satisfy any need for graft.
As an alternative to long-segment corpectomy or multilevel discectomy in patients who need decompression/fusion at three or more motion segments, we have performed corpectomy plus adjacent-level discectomy (three motion segments), or “discontinuous corpectomies” with retention of an intervening body (four motion segments). A ventral plate is thereby afforded at least two extra intermediary points of fixation (Figs. 43-8 and 43-9). The advantage of this technique over multilevel discectomy is the speed and completeness of the decompression and the provision of locally derived autograft. We have experienced no instances of plate loosening or graft migration in this group of patients.
Reconstruction of three- and four-level corpectomies is more problematic. The potential for graft migration, plate loosening, and end-plate fracture is considerably greater. Frequently, such patients are being operated on for correction of a kyphotic deformity, and the bone quality can be suboptimal. Long-segment ventral plates fixated only at their rostral and caudal ends are very prone to failure. Failure typically occurs at the inferior end of the construct where the hardware either fractures or levers off of the caudal body (Fig. 43-10). Simple “kick plates” fitted to the vertebral bodies at the rostral and caudal ends of the construct are worthy of consideration in this circumstance. They do not contribute to the inherent stability of the construct other than to prevent ventral extrusion of the implant. Immediate stability is secured by supplemental, instrumented dorsal spinal fusion and/or halo immobilization (Fig. 43-11).
Summary
Structural autografts and allografts have proved themselves generally satisfactory in single-level disc space arthrodesis and in the reconstruction of single-level corpectomy defects. The risk of graft fracture, resorption, extrusion, and nonunion increases as fusion is extended over an increasing number of motion segments. A variety of interbody devices has been developed with shared advantages of strength, resistance to collapse and extrusion, and an ability to function as carriers of bone graft “generators” while averting the morbidity associated with structural autograft harvest. Polymer cages have the additional benefits of radiolucency and a more physiologically elastic modulus. Recently, ventral interbody cages with integrated ventral fixation systems have been gaining popularity in clinical use.
Kandziora F., Pflugmacher R., Schaefer J. Biomechanical comparison of cervical spine interbody fusion cages. Spine (Phila Pa 1976). 2001;26:1850-1857.
Saunders R.L., Traynelis V.C. Interbody strut techniques. In: Benzel E.C., editor. Spine surgery. New York: Churchill-Livingstone; 1999:241-248.
Uribe J.S., Sangala J.R., Duckworth E.A., Vale F.L. Comparison between anterior cervical discectomy fusion and cervical corpectomy fusion using titanium cages for reconstruction: analysis of outcome and long-term follow-up. Eur Spine J. 2009;18(5):654-662.
1. Castro F.P., Holt R.T., Majd M., Whitecloud T.S.III. A cost analysis of two anterior cervical fusion procedures. J Spinal Disord. 2000;13:511-514.
2. Hacker R.J. A randomized prospective study of an anterior cervical interbody fusion device with a minimum of 2 years of follow-up results. J Neurosurg. 2000;93(2 Suppl):222-226.
3. Savolainen S., Rhine J., Hernesniemi J. A prospective randomized study of anterior single-level cervical disc operations with long-term follow-up: surgical fusion is unnecessary. Neurosurgery. 1998;43:51-55.
4. Sawin P.D., Traynelis V.C., Menezes A.H. A comparative analysis of fusion rates and donor-site morbidity for autogenic rib and iliac crest bone grafts in posterior dorsal cervical fusions. J Neurosurg. 1998;88:255-265.
5. Peelle M.W., Rawlins B.A., Frelinghuysen P. A novel source of cancellous autograft for ACDF surgery: the manubrium. J Spinal Disord Tech. 2007;20(1):36-41.
6. Uribe J.S., Sangala J.R., Duckworth E.A., Vale F.L. Comparison between anterior cervical discectomy fusion and cervical corpectomy fusion using titanium cages for reconstruction: analysis of outcome and long-term follow-up. Eur Spine J. 2009;18(5):654-662.
7. Saunders R.L., Traynelis V.C. Interbody strut techniques. In: Benzel E.C., editor. Spine surgery. New York: Churchill-Livingstone; 1999:241-248.
8. Kandziora F., Pflugmacher R., Schaefer J. Biomechanical comparison of cervical spine interbody fusion cages. Spine (Phila Pa 1976). 2001;26:1850-1857.
9. Scholz M., Reyes P.M., Schleicher P., et al. A new stand-alone cervical anterior interbody fusion device: biomechanical comparison with established anterior cervical fixation devices. Spine (Phila Pa 1976). 2009;34(2):156-160.
10. Lange M., Philipp A., Fink U., Oeckler R. Anterior cervical spine fusion using RABEA-Titan-Cages avoiding iliac crest spongiosa: first experiences and results. Neurol Neurochir Pol. 2000;34:64-69.
11. Zdeblick T.A., Ghanayem A.J., Rapoff A.J., et al. Cervical interbody fusion cages: an animal model with and without bone morphogenetic protein. Spine (Phila Pa 1976). 1998;23:758-766.
12. Shimamoto N., Cunningham B.W., Dmitriev A.E., et al. Biomechanical evaluation of stand-alone interbody fusion cages in the cervical spine. Spine (Phila Pa 1976). 2001;26:E432-E436.
13. Shono Y., McAfee P.C., Cunningham B.W., Brantigan J.W. A biomechanical analysis of decompression and reconstruction methods in the cervical spine: emphasis on a carbon-fiber composite cage. J Bone Joint Surg [Am]. 1993;75:1674-1684.
14. Agrillo U., Mastronardi L., Puzzilli F. : Anterior cervical fusion with carbon fiber cage containing coralline hydroxyapatite: preliminary observations in 45 consecutive cases of soft-disc herniations. J Neurosurg. 2002;96(3 Suppl):273-276.
15. al-Hami S. Cervical monosegmental interbody fusion using titanium implants in degenerative, intervertebral disc disease. Minim Invasive Neurosurg. 1999;42:10-17.
16. Bartels R.H., Donk R., van Azn R.D. Height of cervical foramina after anterior discectomy and implantation of a carbon fiber cage. J Neurosurg. 2001;95(1 Suppl):40-42.
17. Brooke N.S., Rorke A.W., King A.T., Gillian R.W. Preliminary experience of a carbon fibre cage prosthesis for treatment of cervical spine disorders. Br J Neurosurg. 1997;11:221-227.
18. Cho D.-Y., Liau W.-R., Lee W.-Y., et al. Preliminary experience using a polyetheretherketone (PEEK) cage in the treatment of cervical disc disease. Neurosurgery. 2002;51:1343-1349.
19. Das K., Couldwell W.T., Sava G., Taddonio R.F. Use of cylindrical titanium mesh and locking plates in anterior cervical fusion: technical note. J Neurosurg. 2001;94(1 Suppl):174-178.
20. Hacker R.J., Cauthen J.C., Gilbert T.J., Griffith S.L. A prospective randomized multicenter clinical evaluation of an anterior cervical fusion cage. Spine (Phila Pa 1976). 2000;25:2646-2655.
21. Hodges S.D., Humphreys S.C., Eck J.C., et al. A modified technique for anterior multilevel cervical fusion. J Orthop Sci. 2002;7:313-316.
22. Kaden B., Schramm J., Fuhrmann G., Hoffmann C.H. Titanium intervertebral disc and instrumentation for fusion in anterior cervical discectomy: technical note. Neurosurg Rev. 1995;18:25-29.
23. Kale S., Cahill D.W. Current anterior techniques for single-level cervical disc disease. Contemp Neurosurg. 2002;24(6):1-8.
24. Majd M.E., Vadhva M., Holt R.T. Anterior cervical reconstruction using titanium cages with anterior plating. Spine. 1999;24:1604-1610.
25. Matge G. Anterior interbody fusion with the BAK-cage in cervical spondylosis. Acta Neurochir (Wien). 1998;140:1-8.
26. Matge G., Leclercq T.A. Rationale for interbody fusion with threaded titanium cages at cervical and lumbar levels. Results on 357 cases. Acta Neurochir (Wien). 2000;142:425-433.
27. McAfee P.C., Cunningham B.W., Lee G.A., et al. Revision strategies for salvaging or improving failed cylindrical cages. Spine (Phila Pa 1976). 1999;24:2147-2153.
28. Papavero L., Zwoenitzer R., Burkard I., et al. A composite bone graft substitute for anterior cervical fusion: assessment of osseointegration by quantitative computed tomography. Spine. 2002;27:1037-1043.
29. Profeta G., de Falco R., Iannociello G., et al. Preliminary experience with anterior cervical microdiscectomy and interbody titanium cage fusion (Novus CT-Ti) in patients with cervical disc disease. Surg Neurol. 2000;53:417-426.
30. Cloward R.D. Treatment of acute fractures and fracture dislocations of the cervical spine by vertebral body fusion. J Neurosurg. 1961;18:205.
31. Wang X., Chen Y., Chen D., et al. Anterior decompression and interbody fusion with BAK/C for cervical disc degenerative disorders. J Spinal Disord Tech. 2009;22(4):240-245.
32. Smith G.W., Robinson R.A. Treatment of certain spine disorders by anterior removal of the intervertebral disc and interbody fusion. J Bone Joint Surg [Am]. 1958;40:607-624.
33. Brantigan J.W., Steffee A.D., Geiger J.M. A carbon fiber implant to aid interbody lumbar fusion. Mechanical testing. Spine (Phila Pa 1976). 1991;16(6 Suppl):S277-S282.
34. van Jonbergen H.P., Spruit M., Anderson P.G., et al. Anterior cervical interbody fusion with a titanium box cage: early radiological assessment of fusion and subsidence. Spine J. 2005;5(6):645-649.