Chapter 8 Ischaemia, infarction and shock
Blood suffers the various pathological processes that occur in all tissues (neoplasia, nutritional defects, etc., see Ch. 23) but because blood is a tissue that circulates there is also a specific set of pathologies related to defects in flow.
Thrombo-embolic events are major causes of morbidity and mortality in the UK and other developed countries. The majority of readers (and authors) of this book will die from events somewhere along the sequence described in this chapter. Common and serious disorders in which thrombo-embolic mechanisms participate include:
However, no organ is exempt from the harmful consequences of an impaired blood supply, although some (e.g. brain, myocardium), because of their nutritional requirements and inability to regenerate, are more vulnerable than others.
Ischaemia is the result of impaired vascular perfusion, depriving the affected tissue of vital nutrients, especially oxygen. The effects on the tissue can be reversible, but this depends on:
Infarction is death (necrosis) of tissue as a result of ischaemia. Infarction is irreversible, but tissues vary in their ability to repair and replace the loss. Infarction usually results from thrombo-embolic phenomena completely occluding the artery supplying the affected tissue.
Shock (pathophysiological rather than psychological surprise) is a state of circulatory collapse resulting in impaired tissue perfusion. Ischaemia, infarction and shock are, therefore, interrelated phenomena. The cellular responses to impaired nutrition are summarised in Chapter 6.
Although the most common causes of ischaemia and infarction are thrombo-embolic phenomena, vascular insufficiency can also result from other causes (Fig. 8.1).
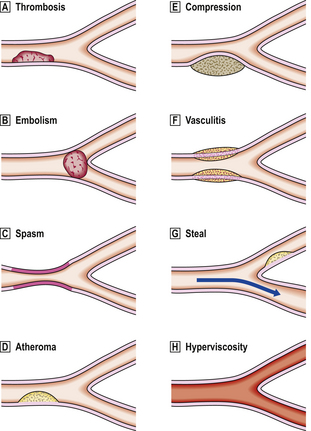
Fig. 8.1 Vascular lesions causing ischaemia. Thrombosis: initiated by either abnormal flow (e.g. stasis, turbulence), damage to vessel wall (e.g. denudation of endothelial lining) or abnormal blood constituents.
Embolism.
Spasm: due to contraction of smooth muscle in media of vessel, for example due to lack of nitric oxide from endothelium.
Atheroma: occurs only in arteries and may in turn be complicated by thrombosis and embolism.
Compression: veins are more susceptible because of their thinner walls and lower intraluminal pressure.
Vasculitis: inflammation of vessel wall narrows lumen and may be complicated by superimposed thrombosis.
Vascular steal: for example, an artery may be narrowed by atheroma but flow is still sufficient to maintain viability of perfused territory; however, flow may be compromised by increased demands of a neighbouring territory.
Hyperviscosity: increased viscosity, for example in hypergammaglobulinaemia resulting from myeloma, causes impaired flow and predisposes to thrombosis.
NON-THROMBO-EMBOLIC VASCULAR INSUFFICIENCY
Vascular flow can be impeded by abnormalities other than thrombo-embolic phenomena.
In arteries, the commonest lesion is atheroma, which in turn may be complicated by thrombo-embolism. In medium-sized arteries, atheromatous plaques (Ch. 13) often narrow the lumen, causing ischaemia and sometimes atrophy of tissues in the hypoperfused territory. Serious consequences include the symptom of angina due to myocardial ischaemia, often heralding the development of irreversible infarction, and hypertension due to renal artery narrowing and hypoperfusion of a kidney, which responds physiologically by increased renin secretion.
Transient arterial narrowing can result from spasm of the smooth muscle in the vessel wall. This can be due to a decrease in nitric oxide (endothelium-derived relaxing factor) production by the vascular endothelium due to cellular injury or loss. Spasm of coronary arteries can lead to angina and both may be relieved by glyceryl trinitrate. Arterial spasm is also responsible for the transient ischaemia of the fingers in Raynaud’s phenomenon.
Blood vessels can be partially or totally occluded by external compression. This is done intentionally during surgery by ligation to prevent haemorrhage from severed vessels, although the results can be disastrous if, accidentally, the wrong vessel is tied off! Because of their thin walls and low intraluminal pressure, veins are more susceptible to occlusion by external compression. This occurs commonly in strangulated hernias, testicular torsion and torsion of ovaries containing cysts or tumours.
‘Steal’ syndromes occur when blood is diverted (‘stolen’) from a vital territory. This results when, proximal to an area of atheromatous narrowing insufficient on its own to produce ischaemia, the arterial stream is diverted along another branch vessel to meet the increased demands of a competing territory or lesion; the territory supplied by the atheromatous vessel then becomes ischaemic. This is a relatively uncommon cause of ischaemia, but often the most challenging diagnostically.
Ischaemia at the arteriolar, capillary and venular level can result from increased whole blood viscosity. Viscosity effects contribute relatively little to the flow characteristics of blood in vessels of large calibre, but in small vessels they are a major factor. Hyperviscosity of blood can occur in myeloma, a tumour of plasma cells, as a result of the abnormally high concentration of gammaglobulin in the plasma and rouleaux formation by red cells.
THROMBO-EMBOLIC VASCULAR OCCLUSION
Clot
When blood stagnates due to the cessation of the pumping action of the heart, or if blood is allowed to stand in a bottle or test tube, then the clotting process is set in motion. A complex series of enzymatic steps (Ch. 23) is activated, resulting in the formation of a fibrin meshwork that entraps the cells into a solid but elastic clot. When this process occurs in the body after death, the red cells tend to settle out before the clot forms, so that these postmortem clots have two layers: a lower, deep red layer (resembling redcurrant jelly) and an upper, clearer layer (resembling chicken fat), with platelets evenly distributed throughout. Since these clots have formed within the body and represent the blood content of the vessel during life, they are moulded to the shape of the vessels in which they have formed. Some time after death, the various blood cells and the cells of the vessel wall begin to release their hydrolytic enzymes and the clot is dissolved.
The sequence of enzymatic reactions involved in the clotting cascade and abnormalities of this system are discussed in Chapter 23.
Thrombosis
Thrombus is a solidification of blood contents that forms within the vascular system during life and is therefore different in concept from a clot. Its mode of formation, its structure and its appearance are all different from those of a clot and the two should never be confused.
Role of platelets
The mechanism for closing small gaps in vessel walls brought about by trauma involves the platelets. Platelets are smaller than red blood cells, rather angular in appearance, and have no nucleus. They are derived from large, multinucleated cells in the bone marrow called megakaryocytes. Although platelets have no nucleus, they are highly structured internally and contain a variety of organelles, some of which are specific to them. As well as mitochondria and the various cytoskeletal elements found in most cells, platelets also contain alpha granules and dense granules. The alpha granules contain several substances involved in the process of platelet adhesion to damaged vessel walls (fibrinogen, fibronectin, platelet growth factor and an antiheparin as well as less well defined substances), and the dense granules contain substances such as adenosine diphosphate (ADP) that cause platelets to aggregate.
Platelets are activated and the contents of their granules are released when the platelets come into contact with collagen, as may be found in damaged vessel walls, or with polymerising fibrin. The platelets change shape and extend pseudopodia; their granules release their contents and the platelets form a mass that covers the vessel wall defect until the endothelial cells have regenerated and repaired the vessel permanently. However, if this process is activated within an intact vessel, it results in a thrombus.
Thrombus formation
There are three predisposing situations that may result in thrombus formation. These were described originally by Virchow and are known as Virchow’s triad. The three factors are:
Not all three are needed for thrombosis to occur, but any one of them may result in thrombosis in a particular case.
If we consider the sequence of events involved in the formation of thrombus on the basis of an atheromatous plaque (Ch. 13), this will serve as a very good example of the factors listed by Virchow.
Arterial thrombosis
In its earliest phase the atheromatous plaque may consist of a slightly raised fatty streak on the intimal surface of any artery, such as the aorta (Fig. 8.2). With time, the plaque enlarges and becomes sufficiently raised to protrude into the lumen and cause a degree of turbulence in the blood flow. This turbulence eventually causes loss of intimal cells, and the denuded plaque surface is presented to the blood cells, including the platelets. The turbulence itself will predispose to fibrin deposition and to platelet clumping; the bare luminal surface of the vessel will have collagen exposed and platelets will settle on this surface. Thus we have two of the factors described in Virchow’s triad operating in an atheromatous plaque. If this plaque exists in the aorta of a smoker or someone with a high cholesterol level and a high level of low density lipoprotein—common risk factors for atheroma—then the third of Virchow’s factors is introduced, since these changes in blood constituents are well known to predispose to thrombus formation. This process, once begun, may be self-perpetuating, as it has been shown that platelet-derived growth factor, which is contained in the alpha granules, causes proliferation of arterial smooth muscle cells, which are an important constituent of the atheromatous plaque.
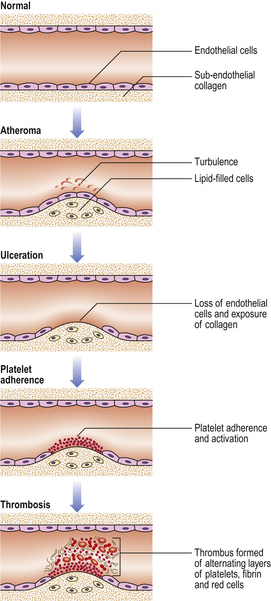
Fig. 8.2 Thrombosis. Thrombosis is exemplified by its occurrence on an atheromatous plaque, a particularly common event. Important steps in this sequence include: loss of endothelial cells and exposure of the underlying collagen; platelet adherence and activation; partial or complete arterial occlusion by the multilayered thrombus.
Thus, the first layer of the thrombus is a platelet layer. Formation of this layer in turn causes the precipitation of a fibrin meshwork, in which red cells are trapped, and a layer of this meshwork is developed on top of the platelet layer. The alternating bands of white platelets and red blood cells in thrombi were first described by Zahn and are called the lines of Zahn. This complex structure now protrudes even further into the lumen, causing more turbulence and forming the basis for further platelet deposition. The normal flow of blood within the vessels is laminar; the cells move in the swifter central lane and the plasma runs along the walls. Therefore, the greatest degree of turbulence occurs at the downstream side of arterial thrombi, as the blood passes over the thrombus, and on the upstream side of venous thrombi for the same reason. Thrombi will therefore grow in the direction of blood flow; this process is known as propagation.
Venous thrombosis
In veins, however, the blood pressure is lower than in arteries and atheroma does not occur; so what initiates venous thrombus formation? Most venous thrombi seem to begin at valves. Valves naturally produce a degree of turbulence because they protrude into the vessel lumen and they may be damaged by trauma, stasis and occlusion. However, thrombi can also form in veins of young, active individuals with no predisposing factors that can be identified. Once they begin, the thrombi grow by successive deposition in the manner described previously and this process may produce a highly patterned, coralline growth (Fig. 8.3). Since normal flow within the vessels is laminar, most of the blood cells are kept away from diseased walls or from damaged vein valves. However, if the blood pressure is allowed to fall during surgery or following a myocardial infarction, then flow is slower through the vessels and thrombosis becomes a likely event. Similarly, the venous return from the legs is very reliant upon calf muscle contraction and relaxation, which massages the veins and, because of the valves, tends to return the blood heartwards. So, if elderly subjects are immobilised for any reason, they are at great risk from the formation of deep leg vein thromboses. The frequency with which deep vein thrombosis is found to occur following surgery is directly related to the enthusiasm with which it is sought (e.g. by the pathologist at postmortem examination) and the sensitivity of the methods used to demonstrate it. Postmortem studies on unselected medical and surgical patients show significant deep vein thrombosis in 34% of the former and 60% of the latter, regardless of the cause of death.
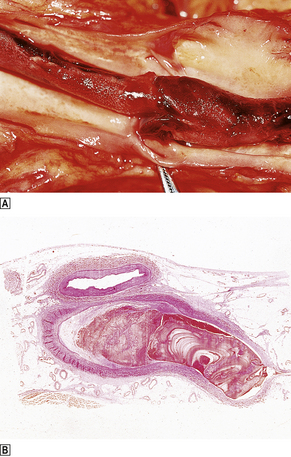
Fig. 8.3 Venous thrombus. Femoral vein opened at autopsy to reveal a thrombus.
Histological section showing the characteristic laminated or coralline structure of a thrombus.
When a vein becomes thrombosed it evokes an inflam-matory reaction, a phenomenon known as thrombophlebitis; the opposite process also occurs—a vein that is inflamed will often thrombose and this is known as phlebothrombosis. The end effect is the same, a thrombosed and inflamed vein, but clearly if there is a predisposing cause then the cause needs to be recognised and treated.
Clinical effects
The effects of thrombosis are apparent only if the thrombus is sufficiently large to affect the flow of blood significantly. Arterial thrombosis results in loss of pulses distal to the thrombus and all the signs of impaired blood supply: the area becomes cold, pale and painful, and eventually the tissue dies and gangrene results. In venous thromboses, 95% of which occur in leg veins, the area becomes tender, swollen and reddened, as blood is still carried to the site by the arteries but cannot be drained away by the veins. The tenderness is due to developing ischaemia in the vein wall initially, but there is also general ischaemic pain as the circulation worsens. The more specific clinical effects of thrombosis depend on the tissue that is affected.
Myocardial infarction is often associated with thrombus formation in coronary arteries and is responsible for numerous sudden deaths (Ch. 13).
Strokes may be due to the formation of thrombus in a cerebral vessel, although they may be also the result of haemorrhage or embolism (Ch. 26).
Thrombophlebitis migrans occurs in previously healthy veins in any area of the body. The thromboses appear and disappear, changing site all the time, and the condition may persist for months or even years. It is extremely ominous and usually indicates the presence of visceral cancer, commonly of the pancreas. The mechanism remains obscure.
Fate of thrombi
Various fates await the newly formed thrombus (Fig. 8.4). In the best scenario it may resolve; the various degradative processes available to the body may dissolve it and clear it away completely. It is not known what proportion of thrombi follow this course, but the total number is likely to be large. A second possibility is that the thrombus may become organised