Chapter 12 Ageing and death
Ageing and death are linked: as people age their death becomes more likely until, in extreme old age, we may be surprised more by continued life than by the event of death. In general we believe that the older an object is the more likely it is that some disaster will occur; old cars break down, old buildings fall down, many old trees succumb to storms. But this is not a universal phenomenon; in a sense, unicellular animals that reproduce by asexual division live for ever. Every amoeba alive today is in direct line of cytoplasmic and nuclear descent from the very first amoeba that ever lived. The single cells of multicellular animals do not behave like this. Some, such as neurones or heart muscle cells, stop dividing at around the time of birth and, if one cell dies, it is usually not replaced. Even those cells that can reproduce in the human body do so less efficiently with the passage of time (Fig. 12.1); thus, elderly individuals experience slower wound healing. If cells from young animals are cultured they seem to be capable of about 50 cell divisions, but cells from older individuals are capable of progressively fewer cell divisions.
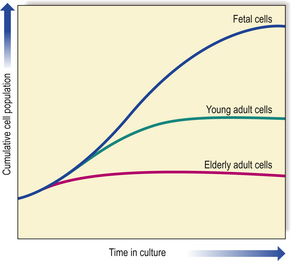
Fig. 12.1 Ageing and the replicative capacity of cells. In cell cultures, the number of mitotic divisions of which cells are capable is inversely proportional to the age of the individual from which the cells were obtained. Thus, fetal cells have considerable growth potential, whereas those from an elderly person are capable of only a few divisions. This is the ‘Hayflick limit’.
AGEING
Let us consider some of the clinical features of old age. It is often said that we are as old as our arteries, suggesting that arterial disease, which certainly increases with old age, is the cause of all the clinical signs of old age. Arterial degeneration, particularly arteriosclerosis (most frequently due to atheroma), is the commonest cause of debility and death in developed countries (Ch. 2). It would seem logical to think that many diseases might also have their roots in a progressively diminishing supply of oxygen and nutrients. However, in autopsies it is not uncommon to see people who have apparently died from ‘old age’ without significant arterial disease; this shows that at least some cases of ageing are not due to arterial problems even though this is commonly associated with ageing. In many developing societies the elderly population is not particularly afflicted by atherosclerosis and yet such individuals show all of the classic bodily features of old age. There is also a significant difference between the diseases that patients die with and the diseases that they die from, but this difference is often very difficult to establish scientifically.
THEORIES OF AGEING
Basically there are two main groups of ageing theories: inbuilt genetic mechanisms and environmental ‘wear and tear’ mechanisms. There is evidence to support both theories but like the nature/nurture arguments in other areas of biology, such as the development of intelligence or of sexual orientation, the two possibilities are not mutually exclusive.
Inbuilt genetic mechanisms (clonal senescence)
Common experience supports the idea that there is an inbuilt ‘allotted life-span’ for humans and other animals. For instance, each animal species seems to have a characteristic natural life expectancy ranging from one day for a mayfly to well over 100 years for some amphibia; not all individuals reach this—under natural conditions prevailing in the wild it may be that no individual reaches this natural limit because of the effects of predators, accidents and disease, or the younger individuals may actively drive out or kill aged members of the group or more passively neglect them when they are no longer useful or economically viable. If animals are kept under ideal conditions it does appear that they age and die at around the same time; barring accidents, there is a characteristic life-span. Most human societies reflect this in their belief that there is a natural life expectancy and that there are natural phases in life: infancy, adolescence, adulthood and ageing.
Evidence for genetic factors
From a scientific point of view, few would deny that the processes of embryogenesis, infancy, adolescence and maturity are genetically programmed, although the individual experience of these stages in life may be very highly modified by environmental conditions; the current estimate is that the more complex and variable features such as behaviour are about 60% genetic and 40% environmental. The process of ageing seems to have a genetic component: members of the same family tend to live to a similar age and they age at a similar rate, leaving aside accident and disease.
The actual inherited mechanism(s) responsible for the genetic component of ageing is still unclear but it is worth noting that longevity appears to be inherited through the female line and that all mammalian mitochondria come from the egg and none is transmitted via the sperm. Cell culture experiments suggest that some gene(s) affecting human ageing are carried on chromosome 1, but, again, the way in which they influence ageing is unclear. There are also some remarkable ‘natural experiments’ in which some human subjects with rare genetic conditions (progerias) such as Werner’s syndrome show premature ageing and die from old-age diseases such as advanced atheroma while still chronologically in their teens or early adulthood. Similarly, Down’s syndrome patients generally age more rapidly; their fibroblasts are capable of fewer cell divisions in culture than those from age-matched controls.
Two related theories of ageing—the disposable soma and antagonistic pleiotropy—are related in that they reflect the priority given to reproduction in natural selection. The optimal deployment of genetic and metabolic resources gives primacy to reproduction rather than to ensuring longevity. Consequently, ageing is the passive result of a lack of genetic drive to optimise or prolong life-span. Indeed, some genes involved in enhancing reproduction are hypothesised to have later deleterious effects.
These observations reveal that at least some features of ageing are genetically based.
Interaction with environmental factors
Social correlations with ageing and death are more difficult to interpret. Many diseases are more common in people from lower socio-economic groups; these individuals exhibit ageing changes and die earlier than age- and sex-matched people from higher socio-economic groups. The most immediate interpretation of these phenomena is that people in these groups are disadvantaged in terms of diet, housing and social welfare generally.
Wear and tear (replication senescence)
The ‘wear and tear’ theories suggest that the normal loss of cells due to the vicissitudes of daily life and the accumulation of sublethal damage in cells lead eventually to system failure of sufficient magnitude that the whole organism succumbs. This theory provides a good explanation of why it is that cardiac and central nervous system failure are such common causes of death, as the functionally important cells in these crucial tissues have very limited ability to regenerate. This theory ultimately depends upon a statistical view of ageing, suggesting that we are all exposed to roughly the same amount of wear and tear and therefore have a narrow range of life expectancy that appears to give us a characteristic life-span.
The various cellular and subcellular mechanisms that have been suggested to cause cumulative damage include:
Role of free radicals
The common pathway resulting in cellular deterioration is currently thought to be the generation of highly reactive molecular species called ‘free radicals’ (Ch. 6). Free radicals are created in neutrophils and macrophages, under carefully controlled conditions, to kill ingested infective organisms; if they are generated accidentally elsewhere there are numerous enzymatic and quenching processes in cells to dispose of them before they can do harm. However, the greater the exposure to free radical inducers (such as toxins in the diet, ionising radiation, etc.), the greater the chance that some damage will occur; these insults will accumulate until they become evident as the ageing process.
Defective repair
Natural experiments lend support to the wear and tear model. There are mechanisms in the cell that deal with damage, particularly DNA damage. These DNA repair mechanisms are numerous but very few deficiency states are well known; the best characterised of these is xeroderma pigmentosum. In this condition young children who are exposed to sunlight develop skin atrophy and numerous skin tumours that are more characteristic of elderly subjects with a long history of chronic sun exposure. This condition suggests that there are at least some mechanisms that hold many of the manifestations of ageing at bay; it is certainly possible that these mechanisms themselves could be susceptible to wear and tear, thus paving the way for more general decline.
Living systems are distinguished from most mechanical systems by their ability to regenerate. If the gastric mucosa is damaged, as it is every day by the simple process of eating, then unspecialised reserve cells at the base of the crypts divide and one of the progeny differentiates to become a new crypt cell; this mechanism is common to most tissues. However, the Hayflick phenomenon suggests that most cells have the capacity for only a limited number of divisions (unlike cancer cells which seem to be immortal) and that this is under genetic control. Therefore, in the final analysis, replicative senescence seems to be dependent upon some form of clonal senescence, and the modifications to the cell during its lifetime act upon an intrinsic life-span programme (Fig. 12.2).
Telomeric shortening
At the tip of each chromosome, there is a non-coding tandemly repetitive DNA sequence; this is the telomere. These telomeric sequences are not fully copied during DNA synthesis prior to mitosis. As a result, a single-stranded tail of DNA is left at the tip of each chromosome; this is excised and, with each cell division, the telomeres are shortened. Eventually the telomeres are so short that DNA polymerase is unable to engage in the subtelomeric start positions for transcription and the cell is then incapable of further replication. In human cells, it is only in germ cells and in embryos that telomeres are replicated by the enzyme telomerase. We might also expect telomerase to be active in cancer cells as these are immortal; recent studies have shown that this is true of many, but not all, cancers.
Telomeric shortening could explain the replication (‘Hayflick’) limit of cells. This is supported by the finding that telomeric length decreases with the age of the individual from which the chromosomes are obtained (Fig. 12.3). In progeria, there is premature telomeric shortening. Furthermore, short telomeres permit chromosomal fusion, and this correlates with the higher incidence of karyotypic aberrations in cells from elderly individuals and in senescent cells in culture.
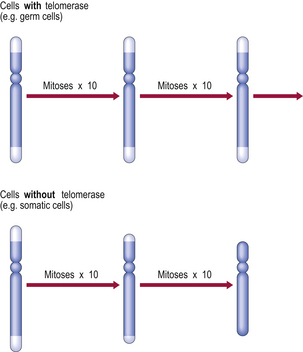
Fig. 12.3 Telomeres, telomerase and replicative capacity. Telomeres are essential for chromosomal copying during the S phase of the cell cycle. However, most somatic cells lack telomerase (the enzyme that regenerates telomeres), so the telomeres shorten with each cell division until chromosomal copying becomes impossible. Germ cells and some neoplastic cells express telomerase and thereby have extended replicative capacity.
CLINOPATHOLOGICAL FEATURES OF AGEING
The chronological age of a human subject can often be estimated to within a decade or so on the basis of physical appearances alone. This is true at all ages and is certainly true in the elderly. The processes of development merge into the processes of ageing, interrupted only by a period of maximum biological capacity commonly referred to as maturity. In most mammals maturity is the period of maximum reproductive capacity and is also the period of greatest prowess in the various ‘pecking orders’ and other social hierarchies that permit the transmission of an individual’s genetic characteristics. As old age supervenes, this complex biological peak or prime begins to deteriorate and the chances of transmitting various genetic combinations decrease. The situation is a little complicated in the human in that the accumulation of wealth in males and the manipulation of fertility in females can modify this decline, but these exceptions are rare and do not affect the general rule.
One of the consequences of a cessation of reproductive capacity in the elderly is that diseases with a genetic component, whose expression in a young adult might result in negative selection pressure, have no such effect; such diseases therefore become preponderant in the elderly. For instance, a disease with a genetic component that proves lethal before or during the reproductive phase would impair the reproductive potential of that individual and the trait would eventually die out apart from new mutations; this obviously does not affect those diseases that become manifest only in old age as these individuals will already have reproduced and passed on the defective gene(s). In any case, there is a direct limit placed on the reproductive ability of the female by the post-menopausal involution of much of the generative system.
There are also other situations in which diseases may be associated with old age but are not related to the causes of old age. Any individual who has lived for 60 years has had more opportunities for accidents than an individual who has lived for only 10 years so far. But this does not mean that accidents are part of the ageing process, although elderly individuals may be more prone to accidents because of failing eyesight and decreased agility. So we should attempt to distinguish between the process of ageing and accompaniments of ageing, and this proves very difficult to do (Fig. 12.4).
Ageing of skin
At a fairly gross level the elderly are identifiable from their wrinkled skin, loss of hair and sagging facial muscles, even if mental acuity is unimpaired (Fig. 12.5). Often the skin is also fragile, loses its youthful elastic recoil and is prone to bruising. Histologically the skin contains less collagen and less elastin, and what is still present is abnormal (Fig. 12.6), as judged by its biochemical properties. Both of these proteins are produced by fibroblasts so it is tempting to wonder whether fibroblasts alter with ageing. Research carried out on fibroblasts in culture has shown that cells from young individuals are capable of more cell divisions (about 50 in total) than are cells from elderly individuals.
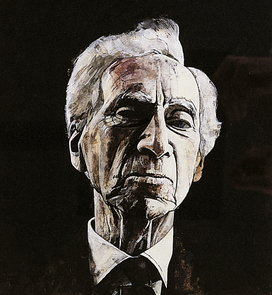
Fig. 12.5 Bertrand Russell in old age. The processes of ageing do not run synchronously. Bertrand Russell (1872–1970), an eminent philosopher, was very active intellectually into his tenth decade.
(Painting by Barry Fantoni)
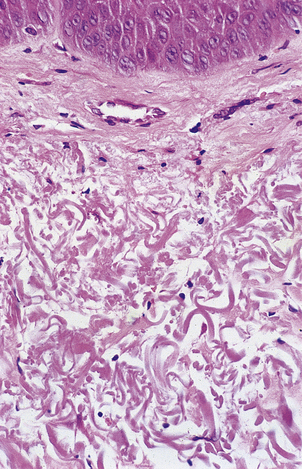
Fig. 12.6 Elastosis in skin. Skin biopsy from the face of an elderly man showing masses of thick homogeneous fibres in the dermis resulting from chronic damage to the dermal collagen by sunlight. This causes the skin to appear wrinkled.
However, it is not enough to concentrate on the obvious; as in all clinical assessments we must consider the whole patient. A full assessment of the skin reveals that the wrinkling that we took to be a cardinal sign of ageing is most pronounced on the sun-exposed areas of the skin; those areas that have remained covered for most of the patient’s life look decades ‘younger’ by this criterion. So, what are we to conclude? Is ageing an environmental phenomenon induced by sunlight? This seems unlikely as it is hard to believe that the diffuse, multi-organ phenomena that we associate with ageing could all be produced by exposing the skin to ultraviolet light.
Osteoarticular ageing
Elderly individuals are often stooped and susceptible to fractures, particularly of the femoral neck. Many post-menopausal women and elderly men have some degree of osteopenia or bone loss. In most cases this is due to osteoporosis (Ch. 25), in which the bone matrix is mineralised as normal but the trabeculae in particular are thinned; this results in fractures from relatively minor trauma and even in spontaneous fractures, commonly of the vertebral bodies leading to a stooped posture (so-called ‘dowager’s hump’). This would appear to be a clear indication that spontaneous deterioration in hormonal function (ovarian function in the case of post-menopausal women) leads to a classic ageing phenomenon; this is often treated with hormone replacement therapy (HRT). However, it is now becoming clear that the development of osteoporosis in old age is much more common in those who were inactive or who had diets low in calcium or vitamin D in youth. Epidemiological evidence is accumulating that many classic features of old age are determined by things that happen during the youth of the individual; an example that has been known for many years is the development of valvular heart disease in the elderly due to rheumatic fever in youth (Ch. 13).
Impaired immunity
The relative immune paresis of old age can result in the recurrence of infections that were contracted many years before and that have never been cleared from the body but have lain dormant. Tuberculosis may erupt again in the elderly, particularly if they become immunosuppressed due to the development of cancer or due to the therapy for cancer, both of which may be immunosuppressive. If Mycobacterium tuberculosis organisms are present, tuberculosis can also arise as a spontaneous expression of progressive decline in the immune system which has been constraining the organism, often for many years. Similarly, the chickenpox (varicella) virus can emerge from its hiding place in nerve ganglia and appear as shingles (herpes zoster) whenever the immune system is suppressed, whether by disease, chemotherapy or just old age.
The ageing of the immune system results in a partial loss of the ability to resist new infections and to continue to control old ones, but there is a paradoxical increase in autoimmune diseases with advancing age. Several possible explanations might be advanced for this: perhaps the processes that maintain immune self-tolerance age quicker than the immune system; autoimmune diseases often follow damage to the tissue concerned and the elderly have had more time to accumulate damage; autoimmune diseases often follow infections and we know that the elderly are more prone to infection. The mechanisms are discussed in Chapter 9.
Brown atrophy
Many body organs in the elderly are reduced in size (atrophy, Ch. 5) and are abnormally brown; this condition is ‘brown atrophy’. The heart and liver are affected commonly. The atrophy is due to senile involution. The brown appearance is caused by excessive amounts of lipofuscin, a granular brown intracellular pigment, often referred to as ‘wear-and-tear’ pigment because of its supposed association with excessive usage of an organ. The mere presence of excess lipofuscin does not appear to interfere with the function of the affected organ.
Cardiovascular changes
In the developed world the major cause of death in adults is the various deteriorations in the cardiovascular system, particularly heart attacks and strokes (Ch. 13). So common is this that we begin to believe that this is the final common pathway of ageing and death. In the developing world, however, these diseases are rare, although they are becoming more common as affluent lifestyles spread; although a smaller percentage of the population survives into old age, those that do still show the classical features of senescence. Interestingly, those who do survive into old age tend to die at the same age as their more affluent counterparts; it is just that considerably fewer actually survive the dangers of infancy and adulthood. Postmortem observations show that in affluent societies most people die with the features of cardiovascular disease, but otherwise identical people in both the developed and developing world die without these features and with no obvious causes for death other than that they are old.
A very common concomitant of ageing is a progressive increase in blood pressure—idiopathic hypertension. This is an interesting condition because the initial pathological event appears to be a sustained increase in small vessel resistance; the hypertension is a ‘physiological’ attempt to overcome this and to maintain the essential perfusion pressure to peripheral tissues. Unfortunately, the increased blood pressure has detrimental effects in larger vessels, such as increased atheroma with increased damage to cardiovascular function—this produces the age-associated major causes of death in affluent societies. A significant clinical dilemma arises from this situation: it is tempting to treat hypertension because of its known association with disease. However, elderly patients need at least part of their hypertension to maintain effective perfusion; pharmacologically lowering their blood pressure to that of a healthy young adult is likely to produce disastrous effects on the end organs that one is trying to protect. The treatment of idiopathic hypertension is a delicate balance of short-term and long-term clinical advantages and disadvantages.
Atheroma itself is a disease we associate with increasing age, but autopsies on young adult males often reveal fatty streaks in the aorta. This again provides evidence that the diseases of old age have unexpected roots in youth and young adulthood.
Fate of permanent cells
Neurological function often declines with age; although a part of this can be attributed to decreased cardiovascular function, many subjects show specific deteriorations and accumulations peculiar to the brain (Ch. 26). Nerve cells, like myocardial cells, are normally incapable of replication. There are no reserve cells and so damage to both brain and heart tissue is permanent. The advantage of this is that nerve cells and cardiomyocytes rarely give rise to tumours in the adult; brain tumours in the adult are derived from the various connective tissue cells of the brain or are secondary deposits from cancers elsewhere in the body. It seems strange that such cells cannot replicate, especially as this inability results in so much clinical damage, but both organs rely upon highly ordered complex electrical activity and it may be that replicating cells within such a system would create more problems than they could solve.
Neoplastic diseases
Most neoplasms are commoner in old age but some, such as neuroblastoma and retinoblastoma, occur only in children. Other tumours may have a biphasic distribution, such as osteosarcoma in which there is a peak incidence in adolescence and a second peak in old age (Ch. 25); the tumours arising in adolescents appear spontaneous whereas those arising in old age almost always occur on the basis of longstanding disease (such as osteomyelitis, malunion of a fracture or Paget’s disease) and it seems probable that these are two different tumours that are just impossible to separate morphologically. A similar situation applies with malignant melanoma of the skin; the peak incidence of melanoma on the legs of women and the backs of men (the commonest sites for melanoma) occurs around the third decade, but another type of malignant melanoma occurs on the face of the elderly (lentigo maligna melanoma). This curious age distribution appears to be related to episodic and severe sun exposure in the younger group, but to chronic long-term exposure in the elderly.
DEATH
Most definitions of death are, in general, rather unsatisfactory; death could be defined as the permanent disappearance of all signs of life, but this presupposes that we have a useful and clear definition of life, which is far from being the case. One of the prime characteristics of living systems is that they are able to maintain homeostasis in the presence of quite extreme fluctuations in the environment; our core body temperature, the concentration of ions in cells, the circulation of the blood and the level of oxygenation of tissues are all kept constant within a tight range that we recognise as physiological. Other factors within the body may vary but we can see these as attempts to bring the body back into a normal range; shivering generates heat in cold situations, sweating causes heat to be lost in hot situations—the end effect is to return the individual to the normal physiological state. Sometimes these mechanisms are overwhelmed, as in hypothermia or in heat-stroke, but we immediately recognise these situations as pathological. If the body cannot return these functions to normal then vital homeostatic control has been permanently lost and death supervenes. This is true at the level of the whole organism (death of the individual) and at the cellular level (cell death, apoptosis). In some cases death of a large group of cells (such as heart cells or brain cells) may result in death of the individual, but death of a single cell by apoptosis cannot, by itself, result in death of the whole organism.
Even though many genetically controlled factors seem to contribute to ageing and death, they do not seem to be synchronous; one individual may be physically very fit and yet develop pre-senile dementia, while another may continue to dominate some intellectual field despite being physically severely incapacitated by old age. Others may be crippled by osteoarthritic disease (an age-associated condition) and yet show no deterioration in any other system. It seems that, although ageing occurs (at least potentially) in all tissues, the final collapse and dissolution is due not to orchestrated deterioration but to the effects of one of the systems reaching a critical and catastrophic point; this then becomes the cause of death (Table 12.1).
Table 12.1 Common modes of death
Mode of death | Common causes | Clinical manifestations |
---|---|---|
Cardiac arrest or dysrhythmia | Ischaemic heart disease | Sudden and often unexpected death |
Pulmonary embolism | Sudden death after period of immobilisation causing deep vein thrombosis | |
Shock | Haemorrhage | Profound hypotension and tachycardia |
Toxaemia due to infection | Hypotension, tachycardia and pyrexia | |
Respiratory failure | Emphysema, pneumonia, asthma | Cyanosis, tachypnoea |
Stroke | Raised intracranial pressure (e.g. tumour, bleeding) | Localised neurological defects, coma |
Cerebral infarction | ||
Renal failure | Chronic renal disease | Low renal output, high blood urea and creatinine |
Liver failure | Acute hepatitis, decompensated cirrhosis, paracetamol poisoning | Jaundice, coma, bleeding |
DYING AND DEATH
Dying and death must be carefully distinguished. This is not just an interesting academic point—it also concerns many patients. People will often make the distinction by saying that they are not afraid of death, but they are afraid of dying because this may be painful, undignified or distressing to their relatives. Also the relationship between dying and death is by no means automatic: someone killed in a road traffic accident was not necessarily dying immediately beforehand; someone with a ruptured aortic aneurysm is certainly dying but in some instances may be saved and not die.
CLINICAL FEATURES OF DEATH
The collapsed elderly patient with no clinical history poses a significant problem. There are no obvious signs of life and preliminary resuscitation attempts have not altered the patient’s state. The ECG shows no complexes. There is no rigor mortis (postmortem muscular spasm) and there is no obvious wound of sufficient severity to suggest a cause of death. Is the patient dead? In practical terms there is a sequence of tests that most doctors will use because any one test is fallible. These are the so-called ‘vital’ signs:
In the absence of all such vital signs, the possibility of hypothermia and deep drug comas should be considered as well as more obscure conditions. If there is doubt early on and if there are no contraindications (such as obvious advanced cancer) then more active resuscitation techniques may be tried involving direct electrical stimulation of the heart or intravenous drugs for the same purpose. If none of these manoeuvres is effective then most doctors will be satisfied and willing to declare the subject dead. Can they still be wrong? Unfortunately in very rare cases the answer is ‘yes’.
The practical importance of accurately establishing the presence of death (or absence of life) is brought into sharp focus by the needs of organ retrieval for transplant surgery; there are strict criteria for deeming a patient to be dead (or brain dead) under these circumstances:
It is important to recognise that factors such as body temperature and the presence of drugs in the body can modify these circumstances. For practical purposes the application of these tests is restricted to doctors with a suitable level of expertise, in the presence of another, independent, doctor who must not be part of the transplant team.
All this serves to underline the difficulty in defining death and in satisfactorily demonstrating it. The definition of death that describes it as the ‘permanent loss of all signs of life’ is doubtless true, but it depends upon the term ‘permanent’—in clinical situations this is often the crucial central issue. It is obviously very unpleasant for all concerned to make a mistake over this issue and it is clinically unacceptable to allow someone to progress from a deep but reversible hypothermic coma to the permanence of death. The problem has been brought into even sharper focus by organ retrieval for transplants where the interests of establishing death, or its imminent inevitability, with certainty and the need to retrieve the tissues in as viable a condition as possible may come into conflict.
BIOLOGICAL MECHANISMS OF DEATH
Sometimes death is sudden and results from damage that exceeds the body’s ability to restore homeostasis. Such situations are common in severe trauma or in cases of system failure of great severity such as total coronary artery occlusion or massive cerebral haemorrhage. In many other cases the immediately pre-terminal state is either coma or shock (Ch. 8). In the case of shock many of the measured features are aberrant biochemical states, such as ketoacidosis, that are themselves the pathological event that, if uncorrected, may go on to cause death. Many other features are bodily mechanisms that have been activated in an attempt to limit or reverse the damage; these include the adrenergic surge leading to vasoconstriction, increased heart rate and redirection of blood flow away from non-essential sites, together within incidental effects, such as sweating, that characterise shock, exemplified by the typical cold, sweaty, ‘shut down’ patient with a rapid pulse. If these adjustments prove inadequate and effective medical intervention is not available, then the patient will progress to death. Under such circumstances it is important to work with the bodily processes that are attempting to restore a normal physiological state and not to try to correct them just because they appear ‘abnormal’.
Blood loss is a particular variant of hypovolaemic shock; here the main thrust of therapy is to identify and treat the cause while returning the circulating volume to normal. Hypovolaemic shock may also be due to infection by bacteria producing toxins that damage vascular endothelium, resulting in vascular dilatation and increased permeability and fluid loss.
Cardiogenic shock produces relative hypovolaemia by failure of the heart to pump an adequate volume into the vessels; simply increasing the circulating volume would convert the signs of acute cardiac failure into those of chronic cardiac failure. In anaphylactic shock the basis of treatment is to withdraw the precipitating cause and to give therapy aimed at reducing the symptoms. Neurogenic shock is often induced by partial abortion where some products of conception are stuck in the uterine os; treatment consists of removing these as rapidly as possible. The salient point is that treatment should be directed at the specific type and cause of shock in order to prevent its progression to death.
CAUSES OF DEATH
Natural causes
The accurate recording of the causes of death is crucial to our understanding of disease in society (Ch. 2), but what exactly caused death in a particular situation is not always easy to determine. Although many people die with widespread cancer and their deaths are quite validly recorded as being due to that cancer, it is by no means always clear what it was about the cancer that killed them. For instance, if someone is said to have died from bronchial carcinoma it is always possible to find a case in which another patient died at a much later stage with a far greater load of cancer, so the volume of disease per se cannot explain why it can kill some people and not others. In such cases we commonly fall back on rather diffuse explanations involving one person’s ‘resistance’ or ‘strength’ compared to another, but the differences can be huge. Cancers produce various substances that have body-wide effects, such as cachexin (tumour necrosis factor-alpha), and there are many other interleukins that may play a role in the disturbed metabolism of many cancer patients. Tumours may also produce various hormones, resulting in paraneoplastic syndromes (Ch. 11). Thus it is entirely possible that many cancer deaths are metabolic deaths. Careful examination of the heart in advanced cancer cases reveals a surprising number of occult deposits of metastatic tumours, and terminal dysrhythmias probably account for a significant number of cancer deaths.
Unnatural causes
Death due to disease and old age is generally regarded as ‘death from natural causes’, but a wide variety of deaths cannot be considered ‘natural’; some deaths involve a synergistic combination of natural and unnatural causes. Obvious unnatural causes include suicide, murder and accidents; less clear are suicides by the mentally ill and accidents caused by natural disease, such as a car crash resulting from a heart attack. From the point of view of the law and the issuing of death certificates, a death is natural if caused by natural disease or old age and is accidental if the accident would not have occurred in the absence of the disease. These decisions are for the medico-legal authorities, as is the decision of whether a death is suicide or murder.
All deaths in which there is an element of doubt must be referred to the appropriate legal officer (for example, the Coroner in England and Wales or the Procurator Fiscal in Scotland). The doctor’s role in these cases is to offer a medical opinion as to the ultimate cause of death. The motivation is a matter for the legal agencies; a doctor may decide that death was due to hanging or paracetamol overdose, but cannot say whether this was accident, suicide or murder.
Within the category of unnatural death we still need to be careful in our interpretation of what caused death. Pressure on the neck can cause death and it may appear to be clear from the circumstances that death was due to hanging or to strangulation. However, pressure on the neck can kill in a variety of ways: mild pressure may be enough to occlude venous return and the subject will die with a congested appearance, swollen protruding tongue and petechial haemorrhages in the eyes; firmer pressure may occlude arterial supply to the brain and the congested features will be much less marked, although the death will still be due to cerebral ischaemia; yet stronger pressure may occlude the trachea, usually breaking the hyoid bone, and death will be due to asphyxia; sudden pressure on the neck may result in instant death by vaso-vagal inhibition with no physical features of congestion at all.
Sudden infant death syndrome
The cause of deaths attributed to ‘sudden infant death syndrome’ remains obscure. The event occurs in very young children in all social classes and no overwhelming common factor has been detected. It may be a collection of disparate and as yet unrecognised disease states, possibly related to the infant’s social condition, or it is still possible that it has a genetic basis. The term ‘sudden infant death syndrome’ is used only when an exhaustive postmortem examination, including histology, fails to reveal an identifiable cause of death. Postmortems under these circumstances are often difficult to contemplate but the results of such examinations are often viewed as a great consolation by bereaved parents as they demonstrate that they were not negligent or culpable in any other way.
TERMINAL EVENTS
In many cases of terminal states that have been studied it seems that perturbations of the central nervous system are the final common pathway leading to irreversibility. Curiously, similar changes are found in elderly patients who are otherwise fit; a sudden decrease in intellectual ability often precedes spontaneous natural death, a situation referred to as the ‘terminal drop’. Perhaps it is not so surprising that a highly complex organism such as the human body should be so crucially dependent upon its co-ordinating and control system that we should come to use this system to determine death and to find that its disruption so closely precedes the end of life.
Pathology of bed rest
Terminally ill patients are often confined to bed. However, prolonged bed rest is not without complications; some are serious. Most complications can be prevented by careful nursing and active physiotherapy.
Decubitus ulcers (bed sores)
Decubitus ulcers occur over pressure points, such as the sacrum and heels in a patient lying supine. They are due to ischaemic necrosis of the skin caused by compression of the vascular supply. Emaciated patients are especially liable to develop decubitus ulcers because there is less subcutaneous fat to diffuse the pressure over bony prominences.
The skin first appears discoloured and becomes gangrenous and then sloughs to expose a raw base of connective tissue. The resulting ulcer frequently becomes infected and may lead to septicaemia.
Decubitus ulcers can be prevented by regularly turning the patient and by using special mattresses. Comatose or severely debilitated patients require highly skilled nursing care to prevent this complication.
Venous thrombosis
Venous return of blood from the legs results from the movement of the surrounding muscles combined with the effect of valves. Immobilised patients commonly develop deep leg vein thrombosis because of venous stasis; this has two consequences:
The latter is an ominous event causing either pulmonary infarction or even sudden death (Ch. 8).
Leg vein thrombosis can be prevented by anticoagulation or compression stockings in cases at risk and by physiotherapy.
Osteoporosis and muscle wasting
Osteoporosis is a condition in which there is a reduction in bone mass (Ch. 25). Patients confined to bed for prolonged periods inevitably lose some bone mass. It also occurs in astronauts in the weightless environment of space. Osteoporosis not only weakens the skeleton, but also liberates much calcium, leading to hypercalciuria and a risk of renal stone formation.
Skeletal muscle mass reduces in immobilised or bed-ridden patients. This mass can be restored when the patient recovers, but physiotherapy may be necessary to accelerate the process.
Hypostatic pneumonia
Patients lying supine in bed have a reduced respiratory excursion and, if severely ill, may have reduced cough reflexes. Furthermore, the posterior regions of the lungs become congested with blood and alveolar oedema can occur. These events combine to predispose the patient to develop a form of bronchopneumonia (Ch. 14) known as hypostatic pneumonia. Hypostatic pneumonia is a serious complication requiring vigorous physiotherapy and antibiotics, but these measures may be unjustified if the patient has some otherwise incurable disease.
Balaban R.S., Nemoto S., Finkel T.. Mitochondria, oxidants and aging. Cell. 120, 2005. 483–495
Cawthon R.M., Smith K.R., O’Brien E., Sivatchenko A., Kerber R.A.. Association between telomere length in blood and mortality in people aged 60 years or older. Lancet. 2003;361:393-395.
Cotton D.W.K.. Death: the cell. Progress in Pathology. 1995;2:1-11.
Cottrell D.A., Blakely E.L., Johnson M.A., et al. Mitochondrial DNA mutations in disease and ageing. In: Ageing vulnerability: causes and interventions. Chichester: Wiley, 2001
Crews D.E.. Senescence, aging and disease. Journal of Physiological Anthropology. 26, 2007. 365–372
Fossel M.. Cell senescence in human aging and disease. Annals of the New York Academy of Sciences. 2002;959:14-23.
Gonzalez-Crussi F.. Three forms of sudden death. London: Picador; 1987.
Goyns M.H.. Genes, telomeres and mammalian ageing. Mechanisms of Ageing and Development. 2002;123:791-799.
Hayflick L.. How and why we age. New York: Ballantine; 1996.
Holliday R.. Understanding ageing. Cambridge: Cambridge University Press; 1995.
Kirkwood T.B.. Molecular gerontology. Journal of Inherited Metabolic Disease. 2002;25:189-196.
Kirkwood T.. Time of our lives: the science of human aging. Oxford: Oxford University Press; 2002.
Lynch T.. The undertaking: life studies from the dismal trade. London: Penguin; 1998.
Nuland S.B.. How we die: reflections of life’s final chapter. London: Chatto & Windus; 1993.
Rose M.R.. Evolutionary biology of ageing. Oxford: Oxford University Press; 1991.
Steel M.. Telomerase that shapes our ends. Lancet. 1995;354:935-936.