Chapter 49 Hearing Prosthetics
Surgical Techniques
Hearing Loss
Hearing loss is usually evaluated through audiologic testing. A typical audiogram has two parts: pure tone audiometry and speech audiometry. In pure tone audiometry, air- and bone-conduction thresholds are tested at different frequencies (Fig. 49-1). In conductive hearing loss, the disparity between air- and bone-conduction thresholds results in an air–bone gap. In sensorineural hearing loss, both air- and bone-conduction thresholds are elevated. In speech audiometry, the ability to perceive and discriminate words and sentences is tested. The speech reception threshold is a measure in decibels (dB) of the speech perception threshold, whereas the speech discrimination score (SDS) is a measure as a percentage of words from a standardized list presented at suprathreshold levels that are recognized and repeated by the patient.
Bone-Anchored Osseointegrated Implants
Osseointegrated implants were first introduced into clinical practice in Scandinavia in the late 1960s, ostensibly for intraoral rehabilitation.1 Since then, osseointegrated implants have gained widespread acceptance in the fields of dental, oral/maxillofacial, craniofacial, and orthopedic surgery. Application of this technology to bone-anchored hearing devices represents a refinement of conventional bone-conduction hearing aids. In bone-conduction hearing aids, sound is transmitted through vibration of the skull to the cochlea. However, the utility of conventional bone-conduction devices is now considered limited due to patient discomfort and limited sound fidelity secondary to soft tissue attenuation. Coupling the bone vibrator to an osseointegrated implant, as originally performed by Tjellström and his team at the Institute of Applied Biotechnology in Sweden in 1977, averts many of these limitations of conventional bone conducting devices.2
The success of this technology relies on two basic principles: the creation of a permanent percutaneous connection and the placement of an osseointegrated titanium abutment upon which a transducer is coupled. The cutaneous-implant interface as originally conceived by Brånemark3 is based on biologic principles observed throughout nature. Teeth and nails (as well as talons, tusks, or claws as found in other species) all interface with a thin, firmly attached cutaneous or mucosal border with little to no hair. This tissue architecture limits tissue mobility and preserves stability of tissue planes while inhibiting the penetration of microbes and subsequent inflammation or infection.4,5 By mimicking these attributes in the skull, the surgeon creates a permanent cutaneous-implant border that the patient may easily maintain.
Advances in metallic biomaterials facilitate the creation of permanent, well-tolerated implant fixtures upon which the transducer is placed. Titanium is the most notable among several materials that have found clinical application in anchoring dental prostheses. This is because of its ability to create a corrosion-resistant oxide layer on the surface of the implant that confers osseointegration potential.6,7 Because the implant may be worn for several decades or longer, the toxicity and carcinogenicity of the oxide coating takes on particular importance.8 Reports have shown that titanium is superior to stainless steel, lacking steel’s high potential for corrosion or the toxicity of its components.9,10 To date, pure titanium appears free of the adverse sequelae seen with other metals and thus continues to represent an ideal implant material.7
Currently, the most widely used osseointegrated hearing device is the Baha implant (Cochlear Corporation, Englewood, CO), shown in Fig. 49-2. The Baha consists of a pure titanium implant and a sound processor. The processor couples directly to the titanium implant via a skin penetrating abutment, utilizing a force-fit, plastic coupling.
Indications
Baha implants were first developed for patients with conductive hearing loss and chronically draining ears, those with discomfort from the sound levels required from a traditional hearing aid, and patients unable to tolerate a hearing aid because of a large mastoid bowl or meatoplasty following chronic ear surgery. Patients with aural atresia are also candidates for Baha implants. Patients who have undergone external auditory canal closure following extensive skull base surgery with preserved inner ear function are also not amenable to traditional hearing aids. This group of patients may benefit from a device that offers bone-anchored hearing.11 Baha implants are also approved for patients with single-sided deafness, for example, due to the effects of a tumor in the cerebellopontine angle or its treatment. In this instance, sound is delivered to the skull on the side lacking sensorineural function and transmitted by bone conduction to the normal contralateral ear, where it is perceived (Fig. 49-3).
Surgical Technique
The postauricular area is cleaned and shaved, and the site of the abutment placement is marked. The abutment is typically placed 50 to 55 mm posterior to the ear canal along the temporal line. A dermatome is used to raise an anteriorly based skin flap. The underlying and surrounding soft tissues are removed, leaving the periosteum intact. A small circle of the periosteum (about 6 mm2) is removed, exposing the underlying bony cortex. A 4-mm hole is drilled at the center of the exposed bony cortex. A countersink is then used to enlarge the hole. The abutment is slowly threaded into the previously prepared hole using the abutment inserter (Fig. 49-4). The skin flap is reflected back to its original position, with a central opening to accommodate the abutment. The edges of the skin flap are then sutured to the surrounding periosteum and skin. The abutment is left undisturbed for 3 to 4 months, which allows osseointegration to occur. Once the abutment is osseointegrated, it is ready for coupling to the sound processor.
Complications
In a study looking at postoperative complications of Baha implant in 149 patients, House and Kutz12 found that the most common complication is skin growth over the abutment (7.4%). This is managed by either steroid application or skin flap revision with removal of the underlying scar tissues. In some cases, a split-thickness skin graft may be needed. Other complications include implant extrusion (3.4%), wound infection (1.3%), and flap necrosis (0.7%). Implant extrusion is more likely to occur in radiated bone and in the pediatric population. Pretreatment with hyperbaric oxygen is suggested in radiated patients. The delay to use is extended in this population and in children by 2 to 3 months to allow for optimal osseointegration.
Outcomes
In one of the largest series to date studying the Baha implant, Håkansson et al.13 reported results from 147 patients over 10 years. Patients were divided into three groups based on their pure tone average (PTA) bone-conduction thresholds: 0 to 45 dB, 46 to 60 dB, and more than 60 dB hearing level. The authors noted a strong relationship between PTA and successful rehabilitation. In the group with the best cochlear reserve (PTA of up to 45 dB), 89% of patients said their hearing was subjectively improved by the implant, while 8% felt their hearing was worse. Conversely, in the groups with progressively less cochlear function (the 46 to 60 dB and the more than 60 dB groups), 61% and 22% of patients reported subjective hearing improvement, respectively. Furthermore, SDSs improved on average from 14% unaided and 67% with a traditional hearing aid to 81% with the Baha. This number increased to 85% if people with a sensorineural loss greater than the 60 dB hearing level were excluded and to 89% if subjects with a PTA worse than 45 dB were excluded. Based on these results, the authors recommended that to be in consideration for a “high success rate” with the Baha, patients should have a PTA by bone conduction that is less than the 45 dB hearing level, though improvements in hearing should still be expected for a PTA of up to 60 dB.
Lustig et al.14 performed a review of experience with the Baha in the United States. The most common indications for implantation included chronic otitis media and external auditory canal stenosis and/or aural atresia. Patients who had undergone skull base surgery and had complete closure of the external auditory canal were also included. Overall, each patient had an average improvement of 32 ± 19 dB with the use of the Baha. Complications were limited to local infection and inflammation at the implant site in 3 of 40 patients and failure to osseointegrate in 1 patient. Patient response to the implant was uniformly satisfactory.
In addition to implantation for purely conductive or mixed hearing losses, emerging data indicate the value of Baha amplification for patients with unilateral profound sensorineural hearing loss. The Baha on the deafened ear effectively expanded the sound field for the patient and improved the patient’s speech understanding in noise, much like a CROS hearing aid or transcranial CROS system.15,16 However, in contrast to CROS, the Baha does not require the placement of an ear mold in the better-hearing ear. As the better-hearing ear functions normally, the acoustic “head shadow” can be used to isolate sounds incident to the deafened side but heard in the better ear through transcranial bone conduction from the Baha. This avoids the potential discomfort and perceptual costs of wearing an ear mold on the better-hearing ear. Preliminary results show subjective improvement in both sound quality and speech understanding in noise.17
Cochlear Implants
Cochlear implants are neural prostheses that convey sound information to the auditory cortex via electrical stimulation of the auditory nerve, bypassing the dysfunctional cochlea in individuals with bilateral severe to profound sensorineural deafness. A typical cochlear implant consists of an external component, which includes the microphone and the speech processor, and an internal component, which includes the receiver-stimulator and the stimulating electrodes. The speech processor is battery powered and is housed in a behind-the-ear unit similar to a hearing aid or in a “body” style encasement worn at the waist, carried in a pocket, or otherwise harnessed to the body. A microphone captures acoustic input and delivers it to the speech processor that, in turn, converts it into electrical signals. An external antenna magnetically retained behind the ear transmits the encoded signals across the scalp via radiofrequency to the antenna of the internal device. The signals are then sorted tonotopically and are delivered to different auditory nerve fibers along the cochlear spiral. An example of a cochlear implant is shown in Fig. 49-5.
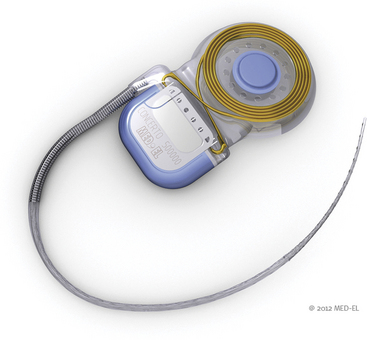
FIGURE 49-5 Cochlear implant. The electrode array and the ground lead are connected to the internal processor.
(Courtesy of Med El.)
Indications
Severe to profound impairment of cochlear function in both ears and anatomic preservation of the auditory nerve in the implanted ear are requirements for cochlear implantation. Currently, criteria vary somewhat but generally include an upper threshold of 40% to 50% speech discrimination with a hearing aid for the poorer hearing ear, with up to 60% in the better-hearing ear, and PTA hearing loss (the average threshold for 500, 1000, and 2000 Hz) of 70 dB or greater in both ears. As experience with cochlear implants grows, outcomes have continued to improve and candidacy criteria based on speech discrimination have continued to evolve toward higher levels of function over the past 25 years.18,19 Mean recognition scores for words in isolation after implantation now far exceed the 40% level, and individuals with some preserved speech-recognition ability preoperatively often score substantially higher postoperatively than prior to implantation.20,21 Residual hearing as reflected in aided speech-recognition levels is an important predictor of implant success.22,23 When combined with duration of deafness, preoperative scores on tests of sentence recognition provide a predictive composite that accounts for approximately 80% of the variance in postoperative word recognition.19
Preservation of auditory nerve integrity should be strongly considered in all neurofibromatosis type 2 (NF2) cases—whether or not the contralateral ear has already demonstrated hearing loss—because it provides an opportunity for future benefit from prosthetic hearing at the cochlea. If the contralateral tumor ear continues to benefit from a hearing aid but is undergoing measurable decline in function, early implantation of the resected side provides an opportunity for the patient to transfer to electrical hearing as acoustic hearing diminishes. Lustig et al. reported on audiologic results in seven patients with NF2 who were implanted following surgical resection with nerve preservation or stereotactic radiation of vestibular schwannomas.24 Whereas hearing acuity and awareness of environmental sound was achieved in all cases, the variability in speech understanding exceeded what is typical for cochlear implantation for cochlear disease. Three patients acquired open-set speech perception in auditory-only testing conditions. In most cases, even when speech understanding was poor, lipreading was enhanced by improved sound awareness, as was sound localization in cases with some residual hearing in the contralateral ear. Trotter and Briggs25 observed favorable communication results in three NF2 patients with cochlear implants in ears whose tumors were treated with stereotactic radiation. Subtotal removal of vestibular schwannoma in two patients and stereotactic radiation therapy in a third were associated with high open-set function in all patients.26 Neff et al. reported sustained open- and closed-set speech perception benefit during an average of 7.9 years.27 They emphasized the utility of promontory electrical stimulation as a predictor of favorable outcome.
Comprehensive assessment of candidacy is essential to minimize risks and realize benefits of cochlear implantation. To ensure complete assessment of candidacy, clinicians should consider the many factors likely to affect performance with a cochlear implant, including audiologic, medical, surgical, developmental, cognitive, and psychosocial factors. Candidates should understand that the cochlear implant is a communication tool and is not a cure for deafness, because expectations largely shape postoperative satisfaction with any form of auditory rehabilitation.28