Chapter 1 Functional Anatomy of the Airway
II Upper Airway
A Nose
The nose serves a number of functions: respiration, olfaction, humidification, filtration, and phonation. In the adult human, the two nasal fossae extend 10 to 14 cm from the nostrils to the nasopharynx. The two fossae are divided mainly by a midline quadrilateral cartilaginous septum together with the two extreme medial portions of the lateral cartilages. The nasal septum is composed mainly of the perpendicular plate of the ethmoid bone descending from the cribriform plate, the septal cartilage, and the vomer (Fig. 1-1). It is normally a midline structure but can be deviated to one side.1 Disruption of the cribriform plate secondary to facial trauma or head injury may allow direct communication with the anterior cranial fossa. The use of positive-pressure mask ventilation in this scenario may lead to the entry of bacteria or foreign material, resulting in meningitis or sepsis. In addition, nasal airways, nasotracheal tubes, and nasogastric tubes may be inadvertently introduced into the subarachnoid space. The posterior portion of the septum is usually midline, but trauma-associated septal deviations and congenital choanal atresia can cause posterior obstruction (Fig. 1-2).
Each nasal fossa is convoluted and provides approximately 60 cm2 surface area per side for warming and humidifying the inspired air.2 The nose is also able to prewarm inspired air to a temperature of 32° C to 34° C, over a wide range of ambient temperatures from 8° C to 40° C.3 The nasal fossa is bounded laterally by inferior, middle, and superior turbinate bones (conchae),4 which divide the fossa into scroll-like spaces called the inferior, middle, and superior meatuses (see Fig. 1-1).2,5,6 The inferior turbinate usually limits the size of the nasotracheal tube that can be passed through the nose, and damage to the lateral wall may occur as a result of vigorous attempts during nasotracheal intubation. The arterial supply to the nasal cavity is mainly from the ethmoid branches of the ophthalmic artery, the sphenopalatine and greater palatine branches of the maxillary artery, and the superior labial and lateral nasal branches of the facial artery. Kiesselbach’s plexus, where these vessels anastomose, is situated in Little’s area on the anterior-inferior portion of the nasal septum. This is a common source of clinically significant epistaxis. The turbinates have a rich vascular supply that affords the nasal airway the ability to expand or contract according to the degree of vascular engorgement. The vascular mucous membrane overlying the turbinates can be damaged easily, leading to profuse hemorrhage. The paired paranasal sinuses—sphenoid, ethmoid, maxillary, and frontal—drain through apertures into the lateral wall of the nose. Prolonged nasotracheal intubation may lead to infection of the maxillary sinus due to obstruction of the ostia.7
The olfactory area is located in the upper third of the nasal fossa and consists of the middle and upper septum and the superior turbinate bone. The respiratory portion is located in the lower third of the nasal fossa.6 The respiratory mucous membrane consists of ciliated columnar cells containing goblet cells and nonciliated columnar cells with microvilli and basal cells. The olfactory cells have specialized hairlike processes, called the olfactory hair, which are innervated by the olfactory nerve.6
The nonolfactory sensory nerve supply to the nasal mucosa is derived from the first two divisions of the trigeminal nerve, the anterior ethmoidal and maxillary nerves. Airborne chemical irritants cause firing of the trigeminal nerves, which presumably are responsible for reflexes such as sneezing and apnea.8 The afferent pathway for the sneezing reflex originates at the histamine-activated type C neurons of the trigeminal nerve, and the efferent pathway consists of several somatic motor nerves. The act of sneezing is associated with an increased intrathoracic air pressure of up to 100 mm Hg and may produce airflow up to 100 mph.8
The parasympathetic autonomic nerves reach the mucosa from the facial nerve after relay through the sphenopalatine ganglion, and sympathetic fibers are derived from the plexus surrounding the internal carotid artery through the vidian nerve.9 Approximately 10,000 L of ambient air passes through the nasal airway per day, and 1 L of moisture is added to this air in the process.10 The moisture is derived partly from transudation of fluid through the mucosal epithelium and partly from secretions produced by glands and goblet cells. These secretions have significant bactericidal properties. Foreign body invasion is further minimized by the stiff hairs (vibrissae), the ciliated epithelium, and the extensive lymphatic drainage of the area.
A series of complex autonomic reflexes controls the blood supply to the nasal mucosa and allows it to shrink and swell quickly. Reflex arcs also connect this area with other parts of the body. For example, the Kratschmer reflex leads to bronchiolar constriction on stimulation of the anterior nasal septum in animals. A demonstration of this reflex may be seen in the postoperative period as a patient becomes agitated when the nasal passage is packed.9
B Pharynx
The pharynx, 12 to 15 cm long, extends from the base of the skull to the level of the cricoid cartilage anteriorly and the inferior border of the sixth cervical vertebra posteriorly.11 It is widest at the level of the hyoid bone (5 cm) and narrowest at the level of the esophagus (1.5 cm), which is the most common site for obstruction after foreign body aspiration. It is further subdivided into the nasopharynx, oropharynx, and laryngopharynx. The nasopharynx, which primarily has a respiratory function, lies posterior to the termination of the turbinates and nasal septum and extends to the soft palate. The oropharynx has primarily a digestive function, starts below the soft palate, and extends to the superior edge of the epiglottis. The laryngopharynx (hypopharynx) lies between the fourth and sixth cervical vertebrae, starts at the superior border of the epiglottis, and extends to the inferior border of the cricoid cartilage, where it narrows and becomes continuous with the esophagus (Fig. 1-3). The eustachian tubes open into the lateral walls of the nasopharynx.
In the lateral walls of the oropharynx are situated the tonsillar pillars of the fauces. The anterior pillar contains the glossopharyngeus muscle, and the posterior pillar contains the palatoglossus muscle.12 The wall of the pharynx consists of two layers of muscles, an external circular layer and an internal longitudinal layer. Each layer is composed of three paired muscles. The stylopharyngeus, salpingopharyngeus, and palatopharyngeus muscles form the internal layer. They elevate the pharynx and shorten the larynx during deglutition. The superior, middle, and inferior constrictors form the external layer; they advance the food in a coordinated fashion from the oropharynx into the esophagus.
1 Defense Against Pathogens
Inhaled particles of size greater than 10 µm are removed by inertial impaction on the posterior nasopharynx. In addition, the inhaled airstream changes direction sharply (90 degrees) at the nasopharynx, resulting in some loss of momentum of the suspended particles. Being unable to remain suspended, the particles are trapped by the pharyngeal walls. The impacted particles are trapped by the circularly arrayed lymphoid tissue located at the entrance to the respiratory and alimentary tracts, known as the ring of Waldeyer (Fig. 1-4). The ring includes masses of lymphoid tissue or tonsils, including the two large palatine, lingual, eustachian tubal, and nasopharyngeal tonsils.
The nasopharyngeal tonsils are also called adenoids.13,14 These structures occasionally impede the passage of ETTs, especially if they are infected and enlarged. Specifically, enlarged adenoid tissue may impede passage of a nasotracheal tube or nasal airway or may simply obstruct the nasal airway passages. The lingual tonsils are located between the base of the tongue and the epiglottis. During routine anesthetic evaluation of the oropharynx, the lingual tonsils are typically not visible. Lingual tonsillar hypertrophy, which is usually asymptomatic, has been reported as a cause of unanticipated difficult intubation and fatal upper airway obstruction.15 In addition, sepsis originating from one of the numerous lymphoid aggregates may lead to a retropharyngeal or peritonsillar abscess, which poses anesthetic challenges.7
Ciliary activity also works to clear trapped nonsoluble particles that are held in an outer mucus layer within the nares. This function is influenced by temperature, viscosity of the mucus, and the osmotic properties of the discharge. The ciliary movement can be negatively affected by many factors, such as viral infections or environmental agents, including air pollution and cigarette smoke. The loss of ciliary function leads to chronic and recurrent infections and can gradually severely injure the respiratory tract, leading to conditions such as chronic bronchitis, sinusitis, and otitis.3
2 Upper Airway Obstruction
a Sedation and Anesthesia
The pharynx is the common pathway for food and the respiratory gases. Patency of the pharynx is vital to the patency of the airway and proper gas exchange in unintubated patients. Proper placement of an ETT requires an understanding of the distance relationships from the oropharynx to the vocal cords and carina. Complications such as a cuff leak at the level of the vocal cords and endobronchial intubation may thus be avoided (Fig. 1-5). Traditionally, it has been taught that upper airway obstruction in patients who are sedated or anesthetized (without an ETT), or who have altered levels of consciousness for other reasons, occurs as a result of the tongue’s falling back onto the posterior pharyngeal wall. Specifically, it is thought that a reduction in genioglossus muscle activity leads to posterior displacement of the tongue with subsequent obstruction.16 However, a number of publications offer a different explanation. The velopharyngeal segment of the upper airway adjacent to the soft palate has recently become the primary focus. This area is particularly prone to collapse and has been found to be the predominant flow-limiting site during sedation and anesthesia,17 speech disorders, and obstructive sleep apnea (OSA) (Fig. 1-6).
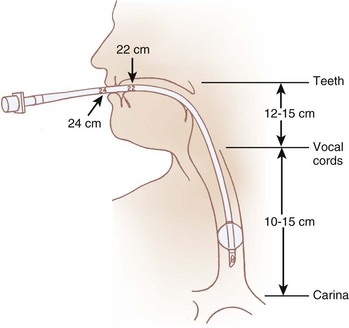
Figure 1-5 Important distances for proper endotracheal tube placement.
(From Stone DJ, Bogdonoff DL: Airway considerations in the management of patients requiring long-term endotracheal intubation. Anesth Analg 74:276, 1992.)
Nandi and colleagues, using lateral radiographs in patients under general inhalational anesthesia, showed that obstructive changes in the airway occurred at the level of the soft palate and epiglottis.18 Shorten and coworkers, using magnetic resonance imaging (MRI), found that patients receiving intravenous sedation for anxiolysis with midazolam had anterior-posterior dimensional changes in the upper airway also at the level of the soft palate and epiglottis while sparing the tongue (see Fig. 1-6).19 In addition, Mathru and coauthors, using MRI to evaluate volunteers receiving propofol anesthesia, found that obstruction occurs at the level of the soft palate and not the tongue.20 Therefore, it appears that the soft palate and epiglottis may play a more significant role than the tongue in pharyngeal upper airway obstruction.
b Obstructive Sleep Apnea
Reduction in the size of the pharynx is also a factor in the development of respiratory obstruction in patients with OSA.21 This problem has been studied with the use of imaging techniques including computed tomography (CT) and MRI, nasopharyngoscopy, fluoroscopy, and acoustic reflection.22 Structural changes that include tonsillar hypertrophy, retrognathia, and variations in craniofacial structures have been linked to sleep apnea risk, presumably by increasing upper airway collapsibility. CT and MRI studies in awake subjects have shown increased fatty tissue deposition and submucosal edema in the lateral walls of the pharynx, both of which can narrow the pharyngeal lumen and predispose to obstruction during sleep, when protective neuromuscular mechanisms wane.23 Obesity, the major risk factor for OSA, has been shown to increase pharyngeal collapsibility through reductions in lung volumes, especially decreases in functional residual capacity (FRC), which are accentuated with the onset of sleep. This decrease in FRC may increase pharyngeal collapsibility through reductions in tracheal traction on the pharyngeal segment.23,24 In awake male patients with OSA, CT has revealed a reduced airway caliber at all levels of the pharynx when compared with normal patients, with the narrowest portion posterior to the soft palate.25
The subatmospheric intra-airway pressure created by contraction of the diaphragm against the resistance of the nose can lead to a reduction in size of the pharyngeal airway. The collapsible segments of the pharynx are divided into three areas: retropalatal, retroglossal, and retroepiglottic. Patency depends on the contractile function of pharyngeal dilator muscles in these segments. The muscles involved are the tensor palatini, which retracts the soft palate away from the posterior pharyngeal wall; the genioglossus, which moves the tongue anteriorly; and the muscles that move the hyoid bone forward, including the geniohyoid, sternohyoid, and thyrohyoid muscles.23,26 In patients with OSA, elevated genioglossal and tensor palatini muscle activity has been observed in the awake state. In contrast, normal subjects have lower activity in these muscles. Observations like these suggest that increased upper airway dilator muscle activity compensates for a more anatomically narrow upper airway in OSA. The reduction in upper airway muscle activity during sleep has been implicated in leading to increased likelihood of upper airway obstruction in patients with OSA compared with healthy subjects.23,24 Studies also show that the configuration of the airway may differ in patients with OSA. Normally, the longer axis of the pharyngeal airway is transverse; however, in OSA patients the anterior-posterior axis is predominant. It is believed that this orientation is less efficient and may affect upper airway muscle function. Continuous positive airway pressure (CPAP) has been found to be effective in treating airway obstruction in these patients. The application of CPAP appears to increase the volume and cross-sectional area of the oropharynx, especially in the lateral axis.27
Clinical problems can arise from both the exaggeration and the depression of upper airway reflexes. A heightened reflex response can lead to laryngospasm and prolonged paroxysm of cough, whereas depressed reflexes can increase the risk of aspiration and compromised airway.28
The velopharynx, an area of the pharynx adjacent to the soft palate, is assuming increased importance in the understanding of OSA, speech disorders, and airway obstruction under anesthesia (see Fig. 1-6 B).29 Fiberoptic nasendoscopy and MRI are recommended for studying velopharyngeal dysfunction.29–31 Six skeletal muscles—the tensor veli palatini, levator veli palatini, musculus uvulae, palatoglossus, palatopharyngeus, and superior constrictor—help form the so-called velopharyngeal sphincter. The proper function of the sphincter is vital to opening and closing of the nasal passages to airflow during deglutition and normal breathing.
C Larynx
The larynx, which lies in the adult neck opposite the third through sixth cervical vertebrae,12 is situated at the crossroads between the food and air passages (or conduits). It is made up of cartilages forming the skeletal framework, ligaments, membranes, and muscles. Its primary function is to serve as the “watchdog” of the respiratory tract, allowing passage only to air and preventing secretions, food, and foreign bodies from entering the trachea. In addition, it functions as the organ of phonation. The larynx may be located somewhat higher in females and children. Until puberty, no differences in laryngeal size exist between males and females. At puberty, the larynx develops more rapidly in males than in females, almost doubling in the anteroposterior diameter. The female larynx is smaller and more cephalad.12 The measurements of the length, transverse diameter, and sagittal diameter of the adult larynx are 44, 36, and 43 mm, respectively, in the male and 41, 36, and 26 mm, respectively, in the female.32 Most larynxes develop somewhat asymmetrically.33 The inlet to the larynx is bounded anteriorly by the upper edge of the epiglottis, posteriorly by a fold of mucous membrane stretched between the two arytenoid cartilages, and laterally by the aryepiglottic folds.9
1 Bones of the Larynx
The hyoid bone (Fig. 1-7) suspends and anchors the larynx during respiratory and phonatory movement. It is U shaped, and its name is derived from the Greek word hyoeides, meaning shaped like the letter upsilon. It has a body, which is 2.5 cm wide by 1 cm thick, and greater and lesser horns (cornu). The hyoid does not articulate with any other bone. It is attached to the styloid processes of the temporal bones by the stylohyoid ligament and to the thyroid cartilage by the thyrohyoid membrane and muscle. Intrinsic tongue muscles originate on the hyoid, and the pharyngeal constrictors are also attached there.4,12,34
2 Cartilages of the Larynx
Nine cartilages provide the framework of the larynx (Fig. 1-8; see also Fig. 1-7). These are the unpaired thyroid, cricoid, and epiglottis and the paired arytenoids, corniculates, and cuneiforms. They are connected and supported by membranes, synovial joints, and ligaments. The ligaments, when covered by mucous membranes, are called folds. The thyroid, cricoid, and arytenoid cartilages consist of hyaline cartilage, whereas the other cartilages are elastic cartilage. Hyaline cartilage tends to ossify in the adult, and this occurs earlier in men than in women.3
a Thyroid Cartilage
The thyroid cartilage, the longest laryngeal cartilage and the largest structure in the larynx, acquires its shieldlike shape from the embryologic midline fusion of the two distinct quadrilateral laminae.35 In females, the sides join at an angle of approximately 120 degrees; in males, the angle is closer to 90 degrees. This smaller thyroid angle explains the greater laryngeal prominence (“Adam’s apple”), longer vocal cords, and lower-pitched voice in males.36 The thyroid notch lies in the midline at the top of the fusion site of the two laminae.37 On the inner side of this fusion line are attached the vestibular ligaments and, below them, the vocal ligaments (Fig. 1-9). The superior (greater) and inferior (lesser) cornua of the thyroid are slender, posteriorly directed extensions of the edges of the lamina. The lateral thyrohyoid ligament attaches the superior cornu to the hyoid bone, and the cricoid cartilage articulates with the inferior cornu at the cricothyroid joint. The movements of this joint are rotatory and gliding and result in changes in the length of the vocal folds.
b Cricoid Cartilage
The cricoid cartilage (see Fig. 1-9) represents the anatomic lower limit of the larynx and helps support it.35 The name cricoid is derived from the Greek words krikos and eidos, meaning shaped like a ring, and it is frequently said to have a signet-ring shape. It is thicker and stronger than the thyroid cartilage and represents the only complete cartilaginous ring in the airway. For this reason, cautious downward pressure on the cricoid cartilage to prevent passive regurgitation is possible without subsequent airway obstruction. Traditionally, it was thought that the pediatric airway was narrowest at the level of the cricoid, and recommendations for ETT size were made based on the size of the cricoid ring. However, studies done with video bronchoscopes on anesthetized and paralyzed children have shown that the glottic opening may be narrower than the cricoid region.38 Therefore, an ETT tube may cause more damage to the vocal cords than to the subglottic area.
The bulky portion or lamina is located posteriorly. The tracheal rings are connected to the cricoid by ligaments and muscles. The cricoid lamina forms ball-and-socket synovial articulations with the arytenoids posterosuperiorly and with the thyroid cartilage inferolaterally and anteriorly.35 It also attaches to the thyroid cartilage by means of the cricothyroid membrane (CTM), a relatively avascular and easily palpated landmark in most adults (see Figs. 1-8 and 1-9).
The inner diameters of the cricoid cartilage have been measured in cadavers, with great variability noted. Randestad and colleagues reported that the smallest diameter is in the frontal plane, which in females ranged from 8.9 to 17.0 mm (mean, 11.6 mm) and in males from 11.0 to 21.5 mm (mean, 15.0 mm).39 They pointed out that placement of a standard size ETT (7 mm inner diameter for females, 8 mm for males) through the cricoid cartilage while preventing mucosal necrosis may be difficult in certain individuals.39 The CTM represents an important identifiable landmark, providing access to the airway by percutaneous or surgical cricothyroidotomy.
The dimensions of the CTM have been identified in cadaveric specimens.40–42 However, the actual methods of obtaining the anatomic measurements varied, making comparisons difficult to interpret. Caparosa and Zavatsky described the CTM as a trapezoid with a width ranging from 27 to 32 mm, representing the actual anatomic limit of the membrane, and a height of 5 to 12 mm.41 Bennett and coauthors40 reported the width as 9 to 19 mm and the height as 8 to 19 mm, whereas Dover and coworkers42 reported a width of 6.0 to 11.0 mm and a height of 7.5 to 13.0 mm, using the distance between the cricothyroid muscles as their horizontal limit. The width and height of the membrane are reported to be smaller in females than in males.10,42
Anteriorly, vascular structures overlie the membrane and pose a risk of hemorrhage.40,42–44 Cadaveric studies have reported the presence of a transverse cricothyroid artery, a branch of the superior thyroid artery, traversing the upper half of the membrane. Therefore, a transverse incision in the lower third of the membrane is recommended. The superior thyroid artery courses along the lateral edge of the membrane, and various branches of the superior and inferior thyroid veins and the jugular veins are also reported to traverse the membrane.
c Arytenoids
The two light arytenoid cartilages (see Fig. 1-8) are shaped like three-sided pyramids, and they lie in the posterior aspect of the larynx.45 The arytenoid’s medial surface is flat and is covered with only a firm, tight layer of mucoperichondrium.45,46 The base of the arytenoid is concave and articulates by a true diarthrodial joint with the superior lateral aspect of the posterior lamina of the cricoid cartilage. It is described as a ball and socket with three movements—rocking or rotating, gliding, and pivoting—that control adduction and abduction of the vocal cords. All such synovial joints can be affected by rheumatoid arthritis. Cricoarytenoid arthritis is present in a majority of patients with rheumatoid arthritis and has been identified as a cause of life-threatening upper airway obstruction.47 Cricoarytenoid arthropathy has also been reported as a rare but potentially fatal cause of acute upper airway obstruction in patients with systemic lupus erythematosus.48
The lateral extension of the arytenoid base is called the muscular process. Important intrinsic laryngeal muscles, the lateral and posterior cricoarytenoids, originate here. The medial extension of the arytenoid base is called the vocal process. Vocal ligaments, the bases of the true vocal folds, extend from the vocal process to the midline of the inner surface of the thyroid lamina (see Fig. 1-9). The fibrous membrane that connects the vocal ligament to the thyroid cartilage actually penetrates the body of the thyroid. This membrane is called Broyles’ ligament. This ligament contains lymphatics and blood vessels and therefore can act as an avenue for extension of laryngeal cancer outside the larynx.35,49 The relationship between the anterior commissure of the larynx and the inner aspect of the thyroid cartilage is important to otolaryngologists, who perform thyroplasties and supraglottic laryngectomies on the basis of its location. A study of cadavers reported that the anterior commissure of the larynx can usually be found above the midpoint of the vertical midline fusion of the thyroid cartilage ala.46,50
d Epiglottis
The epiglottis is considered to be vestigial by many authorities.51 Composed primarily of fibroelastic cartilage, the epiglottis does not ossify and maintains some flexibility throughout life.35,45,52 It is shaped like a leaf and is found between the larynx and the base of the tongue (see Figs. 1-8 and 1-9).34,46 The anterior surface of the epiglottis is concave, and this, in combination with laryngeal elevation, aids in airway protection during deglutition.1 In approximately 1% of the population, the tip and posterior aspect of the epiglottis are visible during a pharyngoscopic view with the mouth opened and tongue protruded. Contrary to reports, this does not always predict ease of intubation.53 The upper border of the epiglottis is attached by its narrow tip or petiole to the midline of the thyroid cartilage by the thyroepiglottic ligament (see Fig. 1-9). The hyoepiglottic ligament connects the epiglottis to the back of the body of the hyoid bone.34,54 The mucous membrane that covers the anterior aspect of the epiglottis sweeps forward to the tongue as the median glossoepiglottic fold and to the pharynx as the paired lateral pharyngoepiglottic folds.35 The pouchlike areas found between the median and lateral folds are the valleculae (Fig. 1-10). The tip of a properly placed Macintosh laryngoscope blade rests in this area. The vallecula is a common site of impaction of foreign bodies, such as fish bones, in the upper airway.
The introduction of advanced scanning techniques using contrast-enhanced multidetector computed tomography (MDCT) has enabled three-dimensional (3-D) and four-dimensional visualization of larger airways. Images of normal and diseased structures can be generated by using special postprocedure CT virtual endoscopy computer software. The presence of air-filled lumens of the upper airways makes it possible for the technical staff to build high-quality endoscopy-like images. Similar techniques have been applied to lower airways also. A virtual endoscopic picture of acute epiglottitis can be seen in Fig. 1-11.55
e Cuneiform and Corniculate Cartilages
The epiglottis is connected to the arytenoid cartilages by the laterally placed aryepiglottic ligaments and folds (see Figs. 1-8 and 1-10)). Two sets of paired fibroelastic cartilages are embedded in each aryepiglottic fold.45 The sesamoid cuneiform cartilage is roughly cylindrical and lies anterosuperior to the corniculate in the fold. The cuneiform may be seen laryngoscopically as a whitish elevation through the mucosa (see Fig. 1-10). The corniculate is a small, triangular object visible directly over the arytenoid cartilage. The cuneiform and corniculate cartilages reinforce and support the aryepiglottic folds35,46 and may help the arytenoids move.12,52
f False and True Vocal Cords
The thyrohyoid membrane (see Figs. 1-7 through 1-9), attaching the superior edge of the thyroid cartilage to the hyoid bone, provides cranial support and suspension.12 It is separated from the hyoid body by a bursa that facilitates movement of the larynx during deglutition.46 The thicker median section of the thyrohyoid membrane is the thyrohyoid ligament, and its thinner lateral edges are pierced by the internal branches of the superior laryngeal nerves.
Beneath the laryngeal mucosa is a fibrous sheet containing many elastic fibers, known as the fibroelastic membrane of the larynx. Its upper area, the quadrangular membrane, extends in the aryepiglottic fold between the arytenoids and the epiglottis. The lower free border of the membrane is called the vestibular ligament; it forms the vestibular folds, or false cords (see Figs. 1-9 and 1-10).34,36,47
The CTM joins the cricoid and thyroid cartilages. The thickened median area of this fibrous tissue, the “conus elasticus,” extends up inside the thyroid lamina to the anterior commissure and continues and blends with the vocal ligament. The cricothyroid ligament thus connects the cricoid, thyroid, and arytenoid cartilages.36,47 The thickened inner edges of the cricothyroid ligament, called the vocal ligament, form the base of the vocal folds (see Fig. 1-10).12,46
g Laryngeal Cavity
The laryngeal cavity (Fig. 1-12) extends from the laryngeal inlet to the lower border of the cricoid cartilage. When it is viewed laryngoscopically from above, two paired inward projections of tissue are visible in the laryngeal cavity: the superiorly placed vestibular folds, or false cords, and the more inferiorly placed vocal folds, or true vocal cords (see Fig. 1-10). The space between the true cords is called the rima glottidis, or the glottis (see Fig. 1-12). The glottis is divided into two parts. The anterior intermembranous section is situated between the two vocal folds. The two vocal folds meet at the anterior commissure of the larynx (see Fig. 1-10). The posterior intercartilaginous part passes between the two arytenoid cartilages and the mucosa, stretching between them in the midline posteriorly, forming the posterior commissure of the larynx (see Fig. 1-10).36 At rest, the vocal processes are approximately 8 mm apart. The area extending from the laryngeal inlet to the vestibular folds is known as the vestibule or supraglottic larynx (see Fig. 1-12). The laryngeal space from the free border of the cords to the cricoid cartilage is called the subglottic or infraglottic larynx.
On the basis of cadaver studies, the measurements of the subglottis have been identified.41,56,57 Understanding the anatomic relationships between the cricothyroid space and the vocal folds is important to minimize complications after cricothyrotomy (Fig. 1-13).58 Bennett and colleagues40 reported this distance to be 9.78 mm. The region between the vestibular folds and the glottis is termed the ventricle or the sinus (see Fig. 1-12). The ventricle may expand anterolaterally to a pouchlike area with many lubricating mucous glands, called the laryngeal saccule (see Fig. 1-12).35 The saccule is believed to help in voice resonance in apes.46,51 The pyriform sinus lies laterally to the aryepiglottic fold within the inner surface of the thyroid cartilage (see Fig. 1-10).46
The epithelium of the vestibular folds is of the ciliated pseudostratified variety (respiratory), whereas the epithelium of the vocal folds is of the nonkeratinized squamous type.37 Therefore, the entire interior of the larynx is covered with respiratory epithelium except for the vocal folds.9
Airway protection is enhanced by the orientation of the cords. The false cords are directed inferiorly at their free border. This position can help to stop egress of air during a Valsalva maneuver. The true cords are oriented slightly superiorly. This prevents air or matter from entering the lungs. Great pressure is required to separate adducted true cords.52 Air trapped in the ventricle during closure pushes the false cords and the true cords more tightly together.15,37,52
3 Muscles, Innervation, and Blood Supply of the Larynx
The complex and delicate functions of the larynx are made possible by an intricate group of small muscles. These muscles can be divided into extrinsic and intrinsic groups.51,54 The extrinsic group connects the larynx with its anatomic neighbors, such as the hyoid bone, and modifies the position and movement of the larynx. The intrinsic group facilitates the movements of the laryngeal cartilages against one another and directly affects glottic movement.
a Extrinsic Muscles of the Larynx
The suprahyoid muscles attach the larynx to the hyoid bone and elevate the larynx. These muscles are the stylohyoid, geniohyoid, mylohyoid, thyrohyoid, digastric, and stylopharyngeus muscles. In the infrahyoid muscle group are the omohyoid, sternothyroid, thyrohyoid, and sternohyoid muscles. These “strap” muscles, in addition to lowering the larynx, can modify the internal relationship of laryngeal cartilages and folds to one another. The inferior constrictor of the pharynx primarily assists in deglutition (Table 1-1).12,35–37
Muscle | Function | Innervation |
---|---|---|
Sternohyoid |
b Intrinsic Muscles of the Larynx
The function of the intrinsic musculature is threefold: (1) to open the vocal cords during inspiration, (2) to close the cords and the laryngeal inlet during deglutition, and (3) to alter the tension of the cords during phonation.12,35,59 The larynx can close at three levels: the aryepiglottic folds close by contraction of the aryepiglottic and oblique arytenoid muscles, the false vocal cords close by action of the lateral thyroarytenoids, and the true vocal cords by contraction of the interarytenoids, the lateral cricoarytenoids, and the cricothyroid.12 The intrinsic muscles include the aryepiglottic and thyroepiglottic, thyroarytenoid and vocalis, oblique and transverse arytenoids, lateral and posterior cricoarytenoids, and cricothyroids (Fig. 1-14). All but the transverse arytenoid are paired.
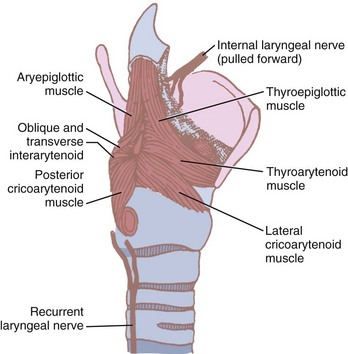
Figure 1-14 Intrinsic muscles of the larynx and their nerve supply.
(Modified from Ellis H, Feldman S: Anatomy for anaesthetists, ed 6, Oxford, 1993, Blackwell Scientific.)
Some authors consider the cricothyroid muscle to be both an extrinsic and an intrinsic muscle of the larynx because its actions affect both laryngeal movement and the glottic structures. It is the only intrinsic muscle found external to the larynx itself. The paired cricothyroid muscles join the cricoid cartilage and the thyroid cartilage (Fig. 1-15). The muscle has two parts. A larger, ventral section runs vertically between the cricoid and the inferior thyroid border. The smaller, oblique segment attaches to the posterior inner thyroid border and the lesser cornu of the thyroid. During swallowing, the muscle contracts and the ventral head draws the anterior part of the cricoid cartilage toward the relatively fixed lower border of the thyroid cartilage. The oblique head of the muscle rocks the cricoid lamina posteriorly. Because the arytenoids do not move, the vocal ligaments are tensed and the glottic length is increased 30%.46,60
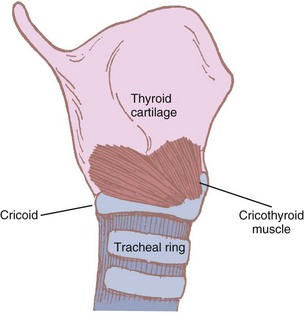
Figure 1-15 The cricothyroid muscle and its attachments.
(Modified from Ellis H, Feldman S: Anatomy for anaesthetists, ed 6, Oxford, 1993, Blackwell Scientific.)
The thick, posterior cricoarytenoid muscle originates near the entire posterior midline of the cricoid cartilage. Muscle fibers run superiorly and laterally to the posterior area of the muscular process of the arytenoid cartilage.20 On contraction, the posterior cricoarytenoid rotates the arytenoids and moves the vocal folds laterally. The posterior cricoarytenoid is the only true abductor of the vocal folds.36,45,46,52
The lateral cricoarytenoid muscle joins the superior border of the lateral cricoid cartilage and the muscular process of the arytenoid. This muscle rotates the arytenoids medially, adducting the true vocal folds.35 The unpaired transverse arytenoid muscle joins the posterolateral aspects of the arytenoids. This muscle, which is covered anteriorly by a mucous membrane, forms the posterior commissure of the larynx. Its contraction brings the arytenoids together and ensures posterior adduction of the glottis.35,36,45
The oblique arytenoids (see Fig. 1-14) ascend diagonally from the muscular processes posteriorly across the cartilage to the opposite superior arytenoid and help close the glottis. Fibers of the oblique arytenoid may continue from the apex through the aryepiglottic fold as the aryepiglottic muscle, which attaches itself to the lateral aspect of the epiglottis. The aryepiglottic muscle and the oblique arytenoid act as a purse-string sphincter during deglutition.46
The thyroarytenoid muscle (see Fig. 1-14) is broad and sometimes is divided into three parts. It is among the fastest-contracting striated muscles.52 The muscle arises along the entire lower border of the thyroid cartilage. It passes posteriorly, superiorly, and laterally to attach to the anterolateral surface and the vocal process of the arytenoid.
The segment of thyroarytenoid muscle that lies adjacent to the vocal ligament (and frequently surrounds it) is called the vocalis muscle. The vocalis is the major tensor of vocal fold and can “thin” the fold to achieve a high pitch. Beneath the mucosa of the fold, extending from the anterior commissure back to the vocal process, is a potential space called Reinke’s space. This area can become edematous if traumatized. The more laterally attached fibers of the thyroarytenoid function as the prime adductor of the vocal folds.46
The most lateral section of the muscle, sometimes called the thyroepiglottic muscle, attaches to the lateral aspects of arytenoids, the aryepiglottic fold, and even the epiglottis. When it contracts, the arytenoids are pulled medially, down, and forward.35,46 This shortens and relaxes the vocal ligament. The function and innervation of the extrinsic muscles are summarized in Table 1-1. Table 1-2 describes the intrinsic musculature of the larynx.
Muscle | Function | Innervation |
---|---|---|
Posterior cricoarytenoid | Abductor of vocal cords | Recurrent laryngeal |
Lateral cricoarytenoid | Adducts arytenoids, closing glottis | Recurrent laryngeal |
Transverse arytenoid | Adducts arytenoids | Recurrent laryngeal |
Oblique arytenoid | Closes glottis | Recurrent laryngeal |
Aryepiglottic | Closes glottis | Recurrent laryngeal |
Vocalis | Relaxes the cords | Recurrent laryngeal |
Thyroarytenoid | Relaxes tension cords | Recurrent laryngeal |
Cricothyroid | Tensor of the cords | Superior laryngeal (external branch) |
c Innervation of the Larynx
The main nerves of the larynx are the recurrent laryngeal nerves and the internal and external branches of the superior laryngeal nerves. The external branch of the superior laryngeal nerve supplies motor innervation to the cricothyroid muscle. All other motor supply to the laryngeal musculature is provided by the recurrent laryngeal nerve (see Fig. 1-14). The superior laryngeal and recurrent laryngeal nerves are derivatives of the vagus nerve.
The superior laryngeal nerve usually separates from the main trunk, off the inferior vagal ganglion, just outside the jugular foramen. At approximately the level of the hyoid bone, it divides into the smaller external and larger internal branches. The external branch travels below the superior thyroid artery to the cricothyroid muscle, giving off a branch to the inferior constrictor of the pharynx along the way. The internal branch travels along with the superior laryngeal artery and passes through the thyrohyoid membrane laterally between the greater cornu of the thyroid and the hyoid. The nerve and artery together pass through the pyriform recess, where the nerve may be anesthetized intraorally. The nerve divides almost immediately into a series of sensory branches and provides sensory innervation from the posterior aspect of the tongue base to as far down as the vocal cords. Sensory innervation of the epiglottis is dense, and the true vocal folds are more heavily innervated posteriorly than anteriorly.52
The left recurrent laryngeal nerve branches from the vagus in the thorax and courses cephalad after hooking around the arch of the aorta in close relation to the ligamentum arteriosum, at approximately the level of the fourth and fifth thoracic vertebrae. On the right, the nerve loops posteriorly beneath the subclavian artery, at approximately the first and second thoracic vertebrae, before following a cephalad course to the larynx. Both nerves ascend the neck in the tracheoesophageal groove before they reach the larynx. The nerves enter the larynx just posterior to, or sometimes anterior to, the cricothyroid articulation. The recurrent laryngeal nerve supplies all the intrinsic muscles of the larynx except the cricothyroid. The recurrent laryngeal nerve also provides sensory innervation to the larynx below the vocal cords. Parasympathetic fibers to the larynx travel along the laryngeal nerves, and the sympathetics from the superior cervical ganglion travel to the larynx with blood vessels. Tables 1-1 and 1-2 summarize the innervation of the laryngeal musculature.
Glottic Closure and Laryngeal Spasm
Stimulation of the superior laryngeal nerve endings in the supraglottic region can induce protective closure of the glottis. This short-lived phenomenon is a polysynaptic involuntary reflex.52 Triggering of other nerves, notably cranial nerves such as the trigeminal and glossopharyngeal, can produce a lesser degree of reflex glottic closure.61,62 The nerve endings in the mammalian supraglottic area are highly sensitive to touch, heat, and chemical stimuli.63 This sensitivity is especially intense in the posterior commissure of the larynx, close to where the pyriform recesses blend with the hypopharynx.63,64 Complex sensory receptors, similar in structure to lingual taste buds, have been demonstrated here.65 Instillation of water, saline, bases, or acids has been demonstrated to cause glottic closure in vitro and in vivo.66 Infants also respond to stimulation with prolonged apnea, although this response disappears later in life.4
The term episodic paroxysmal laryngospasm has been coined to describe laryngeal dysfunction that may or may not arise as a true episode of respiratory distress.66,67 Postoperative superior laryngeal nerve injury has been reported to cause paroxysmal laryngospasm arising with stridor and acute airway obstruction. Superior laryngeal nerve blockade may be temporarily effective in some patients.68
Laryngospasm occurs when glottic closure persists long after removal of the stimulus.62,64 This has led to speculation that laryngospasm represents a focal seizure of the adductors innervated by the recurrent laryngeal nerve.69 This state is initiated by repeated superior laryngeal nerve stimulation.62 It has been reported that the recurrent laryngeal nerve may also be responsible for laryngospasm.70 Symptoms abate, perhaps through a central mechanism, as hypoxia and hypercarbia worsen.71
Vocal Cord Palsies
The recurrent laryngeal nerve may be traumatized during surgery on the thyroid and parathyroid glands.14,72 Malignancy or benign processes of the neck, trauma, pressure from an ETT or a laryngeal mask airway, and stretching of the neck may also affect the nerve.9,54,58,73 The left recurrent laryngeal nerve may be compressed by neoplasms in the thorax, aneurysm of the aortic arch, or an enlarged left atrium (mitral stenosis).34 It may occasionally be injured during ligation of a patent ductus arteriosus. The left nerve is likely to be paralyzed twice as frequently as the right one because of its close relationship to many intrathoracic structures. Damage to the superior laryngeal nerve (external branch) during thyroidectomy is the most common cause of voice change.74
Under normal circumstances, the vocal cords meet in the midline during phonation (Fig. 1-16). On inspiration, they move away from each other. They return toward the midline on expiration, leaving a small opening between them. When laryngeal spasm occurs, both true and false vocal cords lie tightly in the midline opposite each other. To arrive at a clinical diagnosis, the position of the cords must be examined laryngoscopically during phonation and inspiration (Fig. 1-17; see Fig. 1-16).
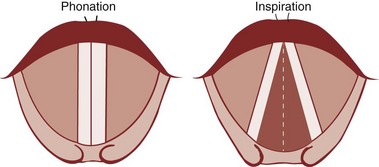
Figure 1-16 Position of vocal cords during phonation and inspiration.
(From Hodder Headline PLC, London.)
The recurrent laryngeal nerve carries both abductor and adductor fibers to the vocal cords. The abductor fibers are more vulnerable, and moderate trauma causes a pure abductor paralysis (Selmon’s law).75 Severe trauma causes both abductor and adductor fibers to be affected.9 Pure adductor paralysis does not occur as a clinical entity. In the case of pure unilateral abductor palsy, both cords meet in the midline on phonation (because adduction is still possible on the affected side). However, only the normal cord abducts during inspiration (see Fig. 1-17). In the case of complete unilateral palsy of the recurrent laryngeal nerve, both abductors and adductors are affected. On phonation, the unaffected cord crosses the midline to meet its paralyzed counterpart, appearing to lie in front of the affected cord (see Fig. 1-17).9 On inspiration, the unaffected cord moves to full abduction. When abductor fibers are damaged bilaterally (incomplete bilateral damage to the recurrent laryngeal nerve), the adductor fibers draw the cords toward each other, and the glottic opening is reduced to a slit, resulting in severe respiratory distress (see Fig. 1-17).51,54 However, with a complete palsy, each vocal cord lies midway between abduction and adduction, and a reasonable glottic opening exists. Thus, bilateral incomplete palsy is more dangerous than the complete variety.
Damage to the external branch of the superior laryngeal nerve or to the superior laryngeal nerve trunk causes paralysis of the cricothyroid muscle (the tuning fork of the larynx), resulting in hoarseness that improves with time because of increased compensatory action of the opposite muscle. The glottic chink appears oblique during phonation. The aryepiglottic fold on the affected side appears shortened, and the one on the normal side is lengthened. The cords may appear wavy. The symptoms include frequent throat clearing and difficulty in raising the vocal pitch.60 A total bilateral paralysis of vagus nerves affects the recurrent laryngeal nerves and the superior laryngeal nerves. In this condition, the cords assume the abducted, cadaveric position.5,9 The vocal cords are relaxed and appear wavy (Fig. 1-18).9,60 A similar picture may be seen after the use of muscle relaxants.
d Blood Supply of the Larynx
Blood supply to the larynx is derived from the external carotid and subclavian arteries. The external carotid gives rise to the superior thyroid artery, which bifurcates, forming the superior laryngeal artery. This artery courses with the superior laryngeal nerve through the thyrohyoid membrane to supply the supraglottic region. The inferior thyroid artery, derived from the thyrocervical trunk, terminates as the inferior laryngeal artery. This vessel travels in the tracheoesophageal groove with the recurrent laryngeal nerve and supplies the infraglottic larynx. There are extensive connections with the ipsilateral superior laryngeal artery and across the midline. A small cricothyroid artery may branch from the superior thyroid and cross the CTM. It most commonly travels near the inferior border of the thyroid cartilage.46
III Lower Airway
A Gross Structure of the Trachea and Bronchi
The adult trachea begins at the cricoid cartilage, opposite the sixth cervical vertebra (see Figs. 1-7 and 1-8). It is 10 to 20 cm long and 12 mm in diameter. It is flattened posteriorly and contains 16 to 20 horseshoe-shaped cartilaginous rings. At the sixth ring, the trachea becomes intrathoracic. The first and last rings are broader than the rest. The lower borders of the last ring split and curve interiorly between the two bronchi to form the carina at the level of the fifth thoracic vertebra (angle of Louis, second intercostal space). The posterior part of the trachea, void of cartilage, consists of a membrane of smooth muscle and fibroelastic tissue joining the ends of the cartilages. The muscle of the trachea is stratified with an inner circular and an outer longitudinal layer. The longitudinal bundles predominate in children but are virtually absent in adults.45,51 Both the trachea and the proximal airways have extensive submucosal glands beneath the epithelium.76
It has been determined that the lengthening of the trachea during neck extension occurs mainly between the vocal cords and the sternal notch. This explains why ETTs fixed at the mouth ascend on average 2 cm in the trachea with neck extension.77
The tip of the tracheal tube moves toward the vocal cords, increasing the chance of accidental extubation. During flexion, the tube moves toward the carina or even the bronchus, depending on the original tube position and the extent of flexion. This is true in both adults and children.77–79 It is therefore necessary to exercise constant vigil when the neck is moved in any direction to rule out displacement of the tube tip.
In the adult, the right main stem bronchus is wider and shorter and takes off at a steeper angle than the left main stem bronchus. Therefore, ETTs, suction catheters, and foreign bodies more readily enter the right bronchial lumen. However, the angulations of the two bronchi are almost equal in children younger than 3 years of age. The right main stem bronchus gives rise to three lobar bronchi, and the left to two. Both the main bronchi and the lower lobe bronchi are situated outside the lung substance. The large main bronchi are 7 to 12 mm in diameter; they divide into 20 bronchopulmonary divisions supplying each respective lobule’s medium bronchi (4 to 7 mm) and small bronchi (0.8 to 4 mm). Bronchioles are bronchi that are smaller than 0.8 mm in diameter. Bronchioles do not have any cartilage in their walls.46 The tracheobronchial airways occupy 1% of the lung volume, with the remaining 99% composed of large vessels and lung parenchyma.76
Bronchioles are of two types, terminal and respiratory. The terminal bronchioles do not bear any alveoli; they lead into the alveoli-bearing respiratory bronchioles. Each terminal bronchiole leads to three respiratory bronchioles, and each respiratory bronchiole leads to four generations of alveolar ducts (Fig. 1-19).46 Although the diameter of each new generation of airway decreases progressively, the aggregate cross-sectional area increases. This is especially true for airways 2 mm or less in diameter, because further branching is not accompanied by concomitant decreases in caliber. The failure of the airway diameter to decrease with subsequent divisions produces the “inverted thumbtack” appearance on a graph depicting increasing surface area as a function of distance from the mouth (Fig. 1-20).7
The bronchi are surrounded by irregular cartilaginous rings that are similar in structure to the trachea except that the attachment of the posterior membrane is more anterior (Fig. 1-21).47 The rings give way to discrete, cartilaginous plates as the bronchi become intrapulmonary at the lung roots (Fig. 1-22). Eventually, even these plates disappear, usually at airway diameters of approximately 0.6 mm.47
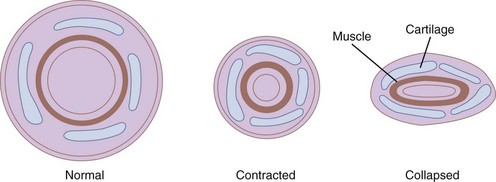
Figure 1-22 Cross-sectional views of medium bronchi (4 to 8 mm diameter) with peribronchial space.
(From Horsfield K: The relation between structure and function of the airways of the lung. Br J Dis Chest 68:145, 1974.)
The rings or plates of the bronchi are interconnected by a strong fibroelastic sheath within which a myoelastic layer consisting of smooth muscle and elastic tissue is arrayed.7 The myoelastic band is arranged in a special pattern called a geodesic network, representing the shortest distance between two points on a curved surface (see Fig. 1-19). This architectural design serves as the strongest and most effective mechanism for withstanding or generating pressures within a tube without fiber slippage along the length of the outer surface of the tube. The network of smooth muscle runs around the airway in two opposing spirals. This arrangement helps in not only constricting the airway but shortening it.80 The primary function of the muscular component is to change the size of the airway according to the respiratory phase. The smooth muscle tone (bronchomotor tone) is predominantly under the influence of the vagus nerve. The elastic layer runs longitudinally but encircles the bronchus at the points of division.7
The muscular layer becomes progressively thinner distally, but its thickness relative to the bronchial wall increases. Therefore, the terminal bronchiole with the narrowest lumen has perhaps the thickest muscle, almost 20% of the total thickness of the wall that lacks cartilaginous support.46,47 For this reason, smaller bronchioles may be readily closed off by action of the musculature during prolonged bronchial spasm. Such an arrangement may facilitate closure of unperfused portions of the lung when a ventilation-perfusion mismatch occurs (e.g., pulmonary embolism). The smooth muscles and the glands of the cartilaginous airways are innervated by the autonomic nervous system. They are stimulated by the vagus and inhibited by sympathetic impulses derived from the upper thoracic ganglia. This smooth muscle mass can increase twofold to threefold in patients with severe asthma.80
B Airway Epithelium and Airway Defense Mechanisms
The cartilaginous airways are lined by a tall, columnar, pseudostratified epithelium containing at least 13 cell types.32 An important function of this lining is the production of mucus, a part of the respiratory defense mechanism. The mucus is steadily propelled to the outside by a conveyer belt mechanism. The large airways have a mucous secretory apparatus that consists of serous and goblet cells and submucous glands. The submucous glands empty into secretory tubules, which in turn connect with the larger connecting ducts. Several connecting ducts unite and form the ciliated duct that opens into the airway lumen. No mucous glands are present in the bronchioles.
The most numerous cells of the large airways are the ciliated epithelial cells, which bear 250 cilia per cell.9,32 The length of the cilia decreases progressively in the smaller airways. On the surface of the cell are found small claws and microvilli. The microvilli probably regulate the volume of secretions through reabsorption, a function that may be shared with the brush cells scattered along the airways. The basal cell, more numerous in the large airways, imparts to the epithelium the pseudostratified appearance. The other cell types, except for the K cell, develop from the basal cell through the intermediate cell. This cell lies in the layer above the basal cell and differentiates into cells with secretory or ciliary function.9,32,48 The K cell, or Kulchitsky-like cell, resembles the Kulchitsky cells of the gastrointestinal tract. These cells take up, decarboxylate, and store amine precursors, such as levodopa (L-dopa) and therefore are known as amine precursor uptake and decarboxylation (APUD) cells. The functions of the K cells are not definitely known, but proposed roles include mechanoreception (stretch) or chemoreception (carbon dioxide). Globule leukocytes are derived from subepithelial mast cells and interact with them to transfer immunoglobulin E to the secretions and to alter membrane permeability to locally produced or circulating antibodies. The ubiquitous lymphocytes and plasma cells defend against pathogens. Table 1-3 lists important cell types that constitute the airway epithelium.
Cell | Probable Function |
---|---|
Epithelial | |
Goblet | Mucous secretion |
Serous | Mucous secretion |
Ciliated | Mucous propulsion-resorption, supportive |
Brush | Mucous resorption |
Basal | Supportive, parent |
Intermediate | Parent |
Clara | Supportive, parent |
Kulchitsky | Neuroendocrine; possible mechanoreceptor, chemoreceptor |
Mesenchymal | |
“Globule” leukocyte | Immunologic defense |
Lymphocyte | Defense |
Modified from Jeffrey PK, Reid L: New features of the rat airway epithelium: A quantitative and electron microscopic study. J Anat 120:295, 1975.
C Blood Supply
Bronchial arteries supply the bronchi and the bronchioles. Arterial supply extends into the respiratory bronchiole. Arterial anastomoses occur in the adventitia of the bronchiole. The branches enter the submucosa after piercing the muscle layer to form the submucosal capillary plexus. The venous radices arising from the capillary plexus reach the venous plexus in the adventitia by penetrating the muscle layer. When the muscle layer contracts, the arteries can maintain forward flow to the capillary plexus. However, the capillaries cannot force the blood back into the venous plexus. Therefore, prolonged bronchial spasm can lead to mucous membrane swelling in the small airways.7 The venous drainage of the bronchi occurs through the bronchial, azygous, hemiazygos, and intercostal veins. There is some communication between the pulmonary artery and the bronchiolar capillary plexus leading to normally occurring “anatomic shunting.”
D Function of the Lower Airway
1 Forces Acting on the Airway
Different forces act on the airway to alter its morphology continuously. These forces are modified by (1) the location of a given airway segment (intrathoracic or extrathoracic), (2) the phases of respiration, (3) lung volume, (4) gravity, (5) age, and (6) disease.47,48
Intrathoracic, intrapulmonary airways such as the distal bronchi and bronchioles are surrounded by a potential space, the peribronchial space (Fig. 1-23). The bronchi are untethered and therefore move longitudinally within this sheath. However, the bronchiolar adventitia is attached by an elastic tissue matrix to the adjoining elastic framework of the surrounding alveoli and parenchyma. Consequently, the bronchioles are subject to transmitted tissue forces.9,43
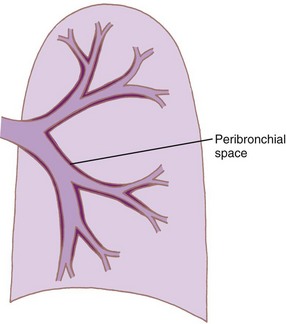
Figure 1-23 Diagram showing formation of peribronchial space by invagination of the visceral pleura.
(From Horsfield K: The relation between structure and function of the airways of the lung. Br J Dis Chest 68:145, 1974.)
Many forces act in concert to modify the airway lumen (Fig. 1-24). The forces that tend to expand the lumen include the pressure of the gas in the bronchi bronchioles and the elastic tissue forces of the alveoli. Forces that tend to close the airway include the elasticity of the bronchial wall, which increases as the lumen expands; the forces related to bronchial muscle contraction; and the pressure of the gas in the surrounding alveoli. The algebraic sum of these forces at any given time determines the diameter of the airway.46,47
The lower part of the trachea and proximal bronchi are intrathoracic but extrapulmonary. Consequently, they are subject to the regular intrathoracic pressures (intrapleural pressure) but not to the tissue elastic recoil forces. The upper trachea is both extrathoracic and extrapulmonary. Although it is unaffected by the elastic recoil of the lung, it is subject to the effects of ambient pressure and cervical tissue forces.43,47
The dynamic forces are altered by gravity such that the forces tending to expand the lung are greater at the top than at the bottom of the lung regardless of whether the patient is prone, supine, or erect.49 The diameter and length of the airways of all sizes vary directly as the cube root of the lung volume varies when the lung expands.50 On expiration below FRC, the retractive forces gradually decrease the airway size toward the point of closing volume. Because of the effect of gravity, the basal airways close first. The retractive forces of the elastic tissues decrease with aging, which explains why closing volume increases with age. This effect is exaggerated in diseases involving elastic tissue damage (e.g., pulmonary emphysema).
2 Relationship Between Structure and Function
The extent to which the retractive forces affect the airway morphology is related to the specific structure of the airway segment in question. When the fibromuscular membrane of the trachea contracts, the ends of the cartilages are approximated, and the lumen narrows in both the intrathoracic and the extrathoracic trachea. When the radial forces decrease airway diameter, the posterior membrane invaginates into the lumen (see Fig. 1-21). However, the rigid cartilaginous hoops prevent luminal occlusion. Extrapulmonary bronchi behave in a similar fashion.
The medium intrapulmonary bronchi within the peribronchial sheath are surrounded by cartilaginous plates. Although these plates add some rigidity to the wall, they do not prevent collapse, so these airways are dependent on the elastic retractive forces of the surrounding tissue (see Fig. 1-24).47 Therefore, forced expiration can collapse many bronchioles in emphysema.
The miniature carinas at small airway bifurcations maintain airway lumens. Intrinsic bronchial muscles reduce the lumen and increase the mean velocity of the airflow during forced expiratory maneuvers, particularly in the peripheral airways with small flow rates. Here, two additional anatomic adaptations contribute to increasing flow rates. First, as the muscular ring contracts, the mucous lining is thrown into accordion-type folds that project into the lumen, further narrowing it (Fig. 1-25).47 Second, the venous plexus situated between the muscle and the cartilage fills and invaginates into the lumen during muscle contraction. These mechanisms permit bronchoconstriction without distorting the surrounding tissues and minimize the muscular effort required to reduce the airway lumen. The drawback of such an arrangement is that even a small amount of fluid or sputum can result in complete occlusion of the small airways.47 Therefore, it is not surprising that airway resistance is increased tremendously during an asthmatic attack that is characterized by both bronchospasm and increased secretions.51,52,81 The small airways can also be affected by interstitial pulmonary edema, a condition in which the peribronchial space can accumulate fluid and isolate the bronchus from the surrounding retractive forces (see Fig. 1-25).
In severe asthma, thickening of the airway walls occurs due to increases in smooth muscle mass, infiltration with inflammatory cells, deposition of connective tissue, vascular changes, and mucous gland hyperplasia. Such a thickening is called airway remodeling. Airway remodeling occurs in milder and even asymptomatic cases of asthma. In the past, airway thickening was confirmed with the use of invasive techniques such as biopsy. More recently, scanning techniques have been used to study airway remodeling.82 MDCT has been used to objectively assess airway remodeling in patients with severe asthma. MDCT used in conjunction with special software can yield reproducible results concerning airway remodeling, and the 3-D airway images allow for correlation of airway function with structural changes.82 The indices of airway wall thickness measured by MDCT are inversely correlated with changes in the 1-second forced expiratory volume (FEV1). Noninvasive measurements of airway thickness over a period of time has the ability to show responses to treatment with corticosteroids and bronchodilators.82,83
V Clinical Pearls
• Cricoarytenoid arthritis can lead to airway difficulties in patients with rheumatoid arthritis or systemic lupus erythematosus.
• To diagnose vocal cord dysfunction, it is necessary to examine the position of the vocal cords during inspiration and phonation.
• The recurrent laryngeal and superior laryngeal nerves may be injured during thyroid surgery, leading to severe vocal cord dysfunction.
• A bilateral partial recurrent nerve palsy is more dangerous than complete palsy.
• Neck movement during anesthesia can result in movement of the tip of the endotracheal tube (ETT).
• New imaging techniques such as virtual endoscopy and multidetector computed tomography (MDCT) are providing added insight into the structure and function of the airways in health and disease.
• Upper airway obstruction in sedated patients occurs at the level of soft palate and not at the level of the tongue.
All references can be found online at expertconsult.com.
3 Pohunek P. Development, structure and function of the upper airways. Paediatr Respir Rev. 2004;5:2–8.
23 Patil SP, Schneider H, Schwartz AR, Smith PL. Adult obstructive sleep apnea: Pathophysiology and diagnosis. Chest. 2007;132:325–337.
24 Isono S. Obstructive sleep apnea of obese adults: Pathophysiology and perioperative airway management. Anesthesiology. 2009;110:908–921.
28 Nishino T. Physiological and pathophysiological implications of upper airway reflexes in humans. Jpn J Physiol. 2000;50:3–14.
29 Rowe MR, D’Antonio LL. Velopharyngeal dysfunction: Evolving developments in evaluation. Curr Opin Otolaryngol Head Neck Surg. 2005;13:366–370.
47 Kolman J, Morris I. Cricoarytenoid arthritis: A cause of acute upper airway obstruction in rheumatoid arthritis. Can J Anaesth. 2002;49:729–732.
55 Thomas BP, Strother MK, Donnelly EF, Worrell JA. CT virtual endoscopy in the evaluation of large airway disease: Review. AJR Am J Roentgenol. 2009;192:S20–S30.
72 Fewins J, Simpson CB, Miller FR. Complications of thyroid and parathyroid surgery. Otolaryngol Clin North Am. 2003;36:189–206.
76 Hyde DM, Hamid Q, Irvin CG. Anatomy, pathology, and physiology of the tracheobronchial tree: Emphasis on the distal airways. J Allergy Clin Immunol. 2009;124:S72–S77.
77 Wong DT, Weng H, Lam E, et al. Lengthening of the trachea during neck extension: Which part of the trachea is stretched? Anesth Analg. 2008;107:989–993.
1 Isaacs RS, Sykes JM. Anatomy and physiology of the upper airway. Anesthesiol Clin North Am. 2002;20:733–745.
2 Reznik GK. Comparative anatomy, physiology, and function of the upper respiratory tract. Environ Health Perspect. 1990;85:171–176.
3 Pohunek P. Development, structure and function of the upper airways. Paediatr Respir Rev. 2004;5:2–8.
4 Roberts JT, Pino R. Functional anatomy of the upper airway. In: Roberts JT, ed. Clinical management of the airway. Philadelphia: WB Saunders; 1994:2–39.
5 Williams PL, Warwick R, Dyson M, Bannister LH. Gray’s anatomy. ed 37. New York: Churchill Livingstone; 1989:1171–1180.
6 Williams PL, Warwick R, Dyson M, Bannister LH. Gray’s anatomy. ed 37. New York: Churchill Livingstone; 1989:365–371.
7 Deutschman CS, Wilton P, Sinow J, et al. Paranasal sinusitis associated with nasotracheal intubation: A frequently unrecognized and treatable source of sepsis. Crit Care Med. 1986;14:111–114.
8 Baraniuk JN, Kim D. Nasonasal reflexes, the nasal cycle, and sneeze. Curr Allergy Asthma Rep. 2007;7:105–111.
9 Linton RF. Structure and function of the respiratory tract in relation to anaesthesia. In: Churchill-Davidson HC, ed. A practice of anaesthesia. ed 5. Chicago: Year Book Medical Publishers; 1984:12–44.
10 Grande CM, Ramanathan S, Grande CM. The structural correlates of airway function. Probl Anesth. 1988;2:175–190.
11 Beasley P. Anatomy of the pharynx and oesophagus. In: Kerr AG, ed. Scott Brown’s Otolaryngology. Oxford: Butterworth-Heinemann; 1997:8.
12 Roberts J. Fundamentals of tracheal intubation. New York: Grune & Stratton; 1983. pp 1–201
13 Green GM. Lung defense mechanisms. Med Clin North Am. 1973;57:547–562.
14 Newhouse M, Sanchis J, Bienenstock J. Lung defense mechanisms (first of two parts). N Engl J Med. 1976;295:990–998.
15 Ovassapian A, Glassenberg R, Randel GI, et al. The unexpected difficult airway and lingual tonsil hyperplasia: A case series and a review of the literature. Anesthesiology. 2002;97:124–132.
16 Morikawa S, Safar P, Decarlo J. Influence of the headjaw position upon upper airway patency. Anesthesiology. 1961;22:265–270.
17 Ayuse T, Hoshino Y, Kurata S, et al. The effect of gender on compensatory neuromuscular response to upper airway obstruction in normal subjects under midazolam general anesthesia. Anesth Analg. 2009;109:1209–1218.
18 Nandi PR, Charlesworth CH, Taylor SJ, et al. Effect of general anaesthesia on the pharynx. Br J Anaesth. 1991;66:157–162.
19 Shorten GD, Opie NJ, Graziotti P, et al. Assessment of upper airway anatomy in awake, sedated and anaesthetised patients using magnetic resonance imaging. Anaesth Intensive Care. 1994;22:165–169.
20 Mathru M, Esch O, Lang J, et al. Magnetic resonance imaging of the upper airway: Effects of propofol anesthesia and nasal continuous positive airway pressure in humans. Anesthesiology. 1996;84:273–279.
21 Hudgel DW. The role of upper airway anatomy and physiology in obstructive sleep apnea. Clin Chest Med. 1992;13:383–398.
22 Ayappa I, Rapoport DM. The upper airway in sleep: Physiology of the pharynx. Sleep Med Rev. 2003;7:9–33.
23 Patil SP, Schneider H, Schwartz AR, Smith PL. Adult obstructive sleep apnea: Pathophysiology and diagnosis. Chest. 2007;132:325–337.
24 Isono S. Obstructive sleep apnea of obese adults: Pathophysiology and perioperative airway management. Anesthesiology. 2009;110:908–921.
25 Haponik EF, Smith PL, Bohlman ME, et al. Computerized tomography in obstructive sleep apnea: Correlation of airway size with physiology during sleep and wakefulness. Am Rev Respir Dis. 1983;127:221–226.
26 Benumof JL. Obstructive sleep apnea in the adult obese patient: Implications for airway management. J Clin Anesth. 2001;13:144–156.
27 Schwab RJ, Gefter WB, Pack AI, Hoffman EA. Dynamic imaging of the upper airway during respiration in normal subjects. J Appl Physiol. 1993;74:1504–1514.
28 Nishino T. Physiological and pathophysiological implications of upper airway reflexes in humans. Jpn J Physiol. 2000;50:3–14.
29 Rowe MR, D’Antonio LL. Velopharyngeal dysfunction: Evolving developments in evaluation. Curr Opin Otolaryngol Head Neck Surg. 2005;13:366–370.
30 The Vancouver Sleep and Breathing Center. Website. Available at http://www.sleep-breathing.bc.ca/index.htm (accessed December 2011)
31 Bivati MJ, Sie K, Wiet GJ, et al. Velopharyngeal insufficiency. Available at http://emedicine.medscape.com/article/873018-overview (accessed December 2011)
32 Gray H, Bannister LH, Berry MM, Williams PL. Gray’s anatomy. ed 38. New York: Churchill Livingstone; 1995:1637.
33 Hirano M, Kurita S, Yukizane K, Hibi S. Asymmetry of the laryngeal framework: A morphologic study of cadaver larynges. Ann Otol Rhinol Laryngol. 1989;98:135–140.
34 Roberts JT. Functional anatomy of the larynx. Int Anesthesiol Clin. 1990;28:101–105.
35 Hanafee WN, Ward PH. Anatomy and physiology. In: Hanafee WN, Ward PH. The larynx: Radiology, surgery, pathology. New York: Thieme Medical; 1990:3–12.
36 Fink RF. Anatomy of the larynx. In: Fink RF, ed. The human larynx: A functional study. New York: Raven Press; 1975:1–19.
37 Tucker HM. Physiology of the larynx. In: Tucker HM, ed. The larynx. ed 2. New York: Thieme Medical; 1993:23–34.
38 Dalal PG, Murray D, Messner AH, et al. Pediatric laryngeal dimensions: An age-based analysis. Anesth Analg. 2009;108:1475–1479.
39 Randestad A, Lindholm CE, Fabian P. Dimensions of the cricoid cartilage and the trachea. Laryngoscope. 2000;110:1957–1961.
40 Bennett JD, Guha SC, Sankar AB. Cricothyrotomy: The anatomical basis. J R Coll Surg Edinb. 1996;41:57–60.
41 Caparosa RJ, Zavatsky AR. Practical aspects of the cricothyroid space. Laryngoscope. 1957;67:577–591.
42 Dover K, Howdieshell T, Colborn GL. The dimensions and vascular anatomy of the cricothyroid membrane: Relevance to emergent surgical airway access. Clin Anat. 1996;9:291–295.
43 Goumas P, Kokkinis K, Petrocheilos J, et al. Cricothyroidotomy and the anatomy of the cricothyroid space: An autopsy study. J Laryngol Otol. 1997;111:354–356.
44 Little CM, Parker MG, Tarnopolsky R. The incidence of vasculature at risk during cricothyroidostomy. Ann Emerg Med. 1986;15:805–807.
45 Fried MP, Meller SM. Adult laryngeal anatomy. In: Fried MP, ed. The larynx: A multidisciplinary approach. Boston: Little, Brown; 1988:41–50.
46 Anatomy of the larynx. In: Tucker HM, ed. The larynx. ed 2. New York: Thieme Medical; 1993:21.
47 Kolman J, Morris I. Cricoarytenoid arthritis: A cause of acute upper airway obstruction in rheumatoid arthritis. Can J Anaesth. 2002;49:729–732.
48 Karim A, Ahmed S, Siddiqui R, et al. Severe upper airway obstruction from cricoarytenoiditis as the sole presenting manifestation of a systemic lupus erythematosus flare. Chest. 2002;121:990–993.
49 Broyles EN. The anterior commissure tendon. Ann Otol Rhinol Laryngol. 1943;52:341–343.
50 Meiteles LZ, Lin PT, Wenk EJ. An anatomic study of the external laryngeal framework with surgical implications. Otolaryngol Head Neck Surg. 1992;106:235–240.
51 Williams PL, Warwick R, Dyson M, Bannister LH. Gray’s anatomy. ed 37. New York: Churchill Livingstone; 1989:1248–1286.
52 Petcu LG, Sasaki CT. Laryngeal anatomy and physiology. Clin Chest Med. 1991;12:415–423.
53 Grover VK, Mahajan R, Tomar M. Class zero airway and laryngoscopy. Anesth Analg. 2003;96:911.
54 Ellis H, Feldman S. Anatomy for anaesthetists, ed 6. Oxford: Blackwell Scientific; 1993. pp 3–81
55 Thomas BP, Strother MK, Donnelly EF, Worrell JA. CT virtual endoscopy in the evaluation of large airway disease: Review. AJR Am J Roentgenol. 2009;192:S20–S30.
56 Isshiki N. Phonosurgery. In: Lawrence VL, Gould WJ. Disorders of human communication. New York: Springer-Verlag; 1984:5–219.
57 Kirchner JA. Cricothyroidotomy and subglottic stenoses. Plast Reconstr Surg. 1991;68:828–829.
58 Lowinger D, Benjamin B, Gadd L. Recurrent laryngeal nerve injury caused by a laryngeal mask airway. Anaesth Intensive Care. 1999;27:202–205.
59 Tucker HM, Harvey M. Anatomy of the larynx. In: Tucker HM, ed. The larynx. ed 2. New York: Thieme Medical; 1993:1.
60 Abelson TI, Tucker HM. Laryngeal findings in superior laryngeal nerve paralysis: A controversy. Otolaryngol Head Neck Surg. 1981;89:463–470.
61 Kirchner J. Laryngeal reflex system. In: Baer T, Sasaki C. Laryngeal function in phonation and respiration. Boston: Little, Brown; 1987:65–70.
62 Sasaki CT, Suzuki M. Laryngeal spasm: A neurophysiologic redefinition. Ann Otol Rhinol Laryngol. 1977;86:150–157.
63 Rex MAE. The production of laryngospasm in the cat by volatile anaesthetic agents. Br J Anaesth. 1970;42:941–947.
64 Thach BT. Neuromuscular control of upper airway patency. Clin Perinatol. 1992;19:773–788.
65 Ide C, Munger BL. The cytologic composition of primate laryngeal chemosensory corpuscles. Am J Anat. 1980;158:193–209.
66 Storey AT, Johnson P. Laryngeal water receptors initiating apnea in the lamb. Exp Neurol. 1975;47:42–55.
67 Gallivan GJ, Hoffman L, Gallivan KH. Episodic paroxysmal laryngospasm: Voice and pulmonary function assessment and management. J Voice. 1996;10:93–105.
68 Wani MK, Woodson GE. Paroxysmal laryngospasm after laryngeal nerve injury. Laryngoscope. 1999;109:694–697.
69 Sasaki CT. Physiology of the larynx. In: English GM, ed. Otolaryngology, vol 3. Philadelphia: Harper and Row; 1984:10–12.
70 Sercarz JA, Nasri S, Gerratt BR, et al. Recurrent laryngeal nerve afferents and their role in laryngospasm. Am J Otolaryngol. 1995;16:49–52.
71 Nishino T, Yonezawa T, Honda Y. Modification of laryngospasm in response to changes in PaCO2 and PaO2 in the cat. Anesthesiology. 1981;55:286–291.
72 Fewins J, Simpson CB, Miller FR. Complications of thyroid and parathyroid surgery. Otolaryngol Clin North Am. 2003;36:189–206.
73 Salem MR, Wong AY, Barangan VC, et al. Postoperative vocal cord paralysis in paediatric patients: Reports of cases and a review of possible aetiological factors. Br J Anaesth. 1971;43:696–700.
74 Kark AE, Kissin MW, Auerbach R, Meikle M. Voice changes after thyroidectomy: Role of the external laryngeal nerve. Br Med J (Clin Res Ed). 1984;289:1412–1415.
75 Ellis H. Clinical anatomy, ed 10. Oxford: Blackwell Scientific; 2002.
76 Hyde DM, Hamid Q, Irvin CG. Anatomy, pathology, and physiology of the tracheobronchial tree: emphasis on the distal airways. J Allergy Clin Immunol. 2009;124:S72–S77.
77 Wong DT, Weng H, Lam E, et al. Lengthening of the trachea during neck extension: Which part of the trachea is stretched? Anesth Analg. 2008;107:989–993.
78 Jin-Hee K, Ro YJ, Seong-Won M, et al. Elongation of the trachea during neck extension in children: Implications of the safety of endotracheal tubes. Anesth Analg. 2005;101:974–977.
79 Toung TJ, Grayson R, Saklad J, Wang H. Movement of the distal end of the endotracheal tube during flexion and extension of the neck. Anesth Analg. 1985;64:1030–1032.
80 Jeffery PK. Remodeling in asthma and chronic obstructive lung disease. Am J Respir Crit Care Med. 2001;164:S28–S38.
81 Maleck WH, Koetter KK, Less SD. Pharyngoscopic views. Anesth Analg. 1999;89:256–257.
82 Aysola RS, Hoffman EA, Gierada D, et al. Airway remodeling measured by multidetector CT is increased in severe asthma and correlates with pathology. Chest. 2008;134:1183–1191.
83 Kasahara K, Shiba K, Ozawa T, et al. Correlation between the bronchial subepithelial layer and whole airway wall thickness in patients with asthma. Thorax. 2002;57:242–246.