Chapter 2 Febrile Seizures
What are Febrile Seizures?
Several definitions of febrile seizures exist. The National Institutes of Health (NIH) Consensus Conference1,2 defined febrile seizures as “events in infancy or childhood, usually occurring between 3 months and 5 years of age, associated with fever but without evidence of intracranial infection or defined cause. Seizures with fever in children who have suffered a previous nonfebrile seizure are excluded.” More recently the International League Against Epilepsy (ILAE)3 defined febrile seizures as “seizures occurring in childhood after age one month, associated with a febrile illness not caused by an infection of the central nervous system, without previous neonatal seizures or a previous unprovoked seizure, and not meeting criteria for other acute symptomatic seizures.”
Note that the NIH and ILAE definitions differ in both the minimal and maximal age “permitted” for febrile seizures, and this may cause confusion among parents and physicians. In addition, although both the NIH and ILAE definitions exclude children with prior afebrile seizures and those with seizures due to an intracranial infection or other specific cause, they do not exclude children with preexisting neurological deficits. This may confound studies looking at the cognitive outcome of these seizures. Note also that “fever” is not defined. In general, a temperature higher than 38°C4,5 or 38.4°C (101°F) has been defined as constituting fever.
The majority of febrile seizures occur early in the febrile episode and often are the presenting sign of the fever.6–8 A typical scenario is a child who presents with a seizure, and the presence of fever is discovered later, once the child arrives in an emergency room. Whether the rate of temperature rise is an important determinant of the occurrence of a febrile seizure, rather than the actual temperature achieved, is not well substantiated.9,10
Frequency and Pathophysiology of Febrile Seizures
Several large population-based studies examined the incidence (the number of new cases occurring in a defined population over a specified period of time) and the prevalence (proportion of individuals in a population that has ever had the disorder) of febrile seizures. They found similar rates (2 to 5%) in the Western world and higher rates in Japan and Guam (Figure 2-1). For examples, in Rochester, Minnesota, the annual incidence of febrile seizures was ∼4/1000 children younger than age 5, and ∼2% of children experienced a febrile seizure by the age of 5.11,12 The large Oakland, California,13 and British studies14 also found that by the age of 5 years 2 to 2.3% of children had had a febrile seizure. The National Collaborative Perinatal Project (NCPP) reported a somewhat higher rate (3.5%15,16), that was similar to results from Sweden (cumulative prevalence of 4.1% in children under age 517) and Holland (a rate of 3.9%18).
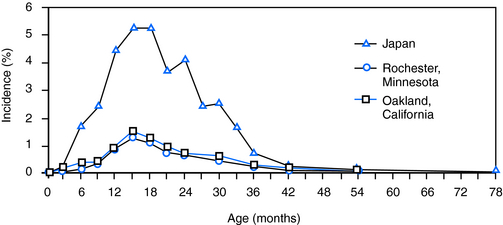
Figure 2–1 Age-specific incidence of febrile seizures in the United States and in Japan.
Reprinted with permission from Hauser [1994], Blackwell Publishing.
In contrast to their frequency in the Western world, febrile seizures occur in ∼8 to 10% of Japanese children4 and of those in Guam.19,20 This increased incidence is likely a result of both environmental factors (e.g., parents and children sleep in close proximity, so that febrile seizures are more likely to be observed), as well as genetic elements.
Why will a child have a febrile seizure? Current evidence supports the notion that in a given child, environmental and genetic factors may interact to determine whether that child will have a febrile seizure. Furthermore, the relative contribution of each varies significantly: febrile seizures occur sporadically as well as run in families, where the contribution of genetic background to their onset is likely higher.21 Looking at risk factors for febrile seizures, Bethune et al.22 found that both attending day care (increasing the chance of fever and infection) and a history of a family member with febrile seizure tripled the risk of having such seizures. Contribution of presumed genetic background (presence of febrile seizures in a relative), as well as environmental factors (e.g., maternal smoking during pregnancy), was reported also by Berg et al.23
How does fever lead to a febrile seizure? Temperature influences neuronal electrophysiological activity.24 For example, the function of ion channels such as the TRPV4 (transient receptor potential vanilloid 4) are markedly dependent on temperature in the physiological and fever ranges, ∼36°C to 42°C.25 Children can also develop seizures on simple hyperthermia and not fever, such as hyperthermia related to anticholinergic overdose26 or hot showers,27 suggesting that increased temperature in itself can be a convulsant in children.
Recently, hyperthermia in an animal model has been shown to induce hyperventilation resulting in alkalosis that in turn provoked seizures.28 This happened 25 to 30 minutes after the onset of the hyperthermia and was not observed in a second febrile seizure model in an immature rat.29 In the latter case, seizures commenced within A: 2.9 ∀ 0.1 minutes of the onset of the hyperthermia procedure (n = 52), and respiratory rates when seizures started B: 167 ∀ 4.9, n = 12 did not differ significantly from those rates prior to C: 161.8 ∀ 2.6 or during the first minute of D: 173 ∀ 3.7 the hyperthermia. Whether alkalosis plays a role in febrile seizures in children remains unknown.
Genetic susceptibility may increase the ability of fever to provoke seizures. Recently, mutations of several ion channels that predispose to febrile seizures have been described,30–33 including mutations of sodium30,31 and chloride (GABAA receptor32,33) channels. In many families, these febrile seizures are associated with epilepsy of diverse phenotypes, a syndrome termed GEFS+ (generalized epilepsy with febrile seizures plus).34 Other single-gene mutations, such as in the interleukin gene promoter (see later discussion) might also render individuals more susceptible to febrile seizures,35,36 and multigene interactions might contribute to the occurrence of these seizures in a more complex and subtle way.
Fever may promote seizures via the function of pyrogenic cytokines including interleukin-1.37,38 This notion is supported by reports of mutations in the interleukin-1 gene promoter that result in increased production of the cytokine in individuals with febrile seizures,36 although the significance of this finding has been debated.39 In summary, the contribution of gene-environment interaction to the generation of febrile seizures, and to their potential causal relationship to epilepsy,40 is a topic of active investigation; increasingly sophisticated experimental tools should rapidly advance our understanding of this question.
Specific infectious etiology of the fever might contribute to the generation of human febrile seizures. A disproportionate number of febrile seizures has been associated with infection with the human herpes virus 6 (HHV6) in some,41 but not all, studies.42 This suggests that mechanisms specific to this virus, including perhaps a unique profile of cytokine induction, might selectively augment neuronal excitability and thus preferentially provoke seizures.
Types of Febrile Seizures
Febrile seizures are divided into: (a) simple (∼70 to 80% of the total5,15,18,43–45), (b) complex, and (c) febrile status epilepticus (FSE).
Simple febrile seizures are short generalized seizures, typically but not necessarily motor, that last less than 108,46 or 1516,45 minutes. Specifically, these seizures do not have focal features and do not recur within the same febrile episode (or within 24 hours from the first seizure). Complex febrile seizures are either longer, lasting >10 minutes8,46 or >15 minutes,16,45 have focal features, or recur within 24 hours of the first episode15,46 or within the same febrile illness.47 Febrile status epilepticus (FSE) is defined as febrile seizures longer than 30 minutes. Note that Scott et al.48,49 define seizures lasting more than 30 minutes in normal children without intracranial infection as prolonged febrile seizures rather than status epilepticus.
Complex febrile seizures, and particularly febrile status epilepticus, are associated with a higher risk of later epilepsy (see later discussion), so recognizing them is of clinical importance. Recent research has found that the duration of a seizure is regulated in each child independent of the probability of having a seizure. Thus, Shinnar et al.50 have found that once a child has had a prolonged seizure, a subsequent seizure is likely to be prolonged as well. This is important for targeting children for abortive therapy to prevent long febrile seizures (see later discussion).
Outcome of Febrile Seizures
The outcome of febrile seizures is measured in terms of cognitive function (e.g., school performance, cognitive tests) and the risk for developing epilepsy. Several large studies have suggested that simple febrile seizures, nonfocal and with a duration of less than 10 to 15 minutes, do not lead to long-term sequelae16,43,46,51,52 in either of these realms. However, a smaller study from Taiwan cautioned that infants less than a year of age at the time of their seizure might function less well in certain learning tasks.53,54 By and large, however, the overwhelming evidence regarding simple febrile seizures is that they are benign.
The outcome of complex febrile seizures and of febrile status epilepticus is more controversial.16,45,46,55 In terms of cognitive function, both the American National Collaborative Perinatal Project16,56 and the British cohort51 used general broad measures such as school performance as outcome and evaluated children at age 6 or 10, not later. They concluded that complex febrile seizures do not alter cognition, but their design, as mentioned earlier, limits the confidence of these conclusions. Evaluations of cognitive outcome of prolonged febrile seizures or febrile status are complicated by the fact that many epidemiological studies do not exclude children who are prior neurologically compromised (see definition of febrile seizures in the section “What Are Febrile Seizures?”). Inclusion of children with neurological deficits before the seizures occurred complicates attempts to look at the impact of these seizures on intellect. Notably, British investigators have recently begun to better define the populations they study to exclude children with neurological deficits. The results of these studies should be helpful to sort out the consequences of these seizures on the normal developing brain.57
Whether prolonged febrile seizures and febrile status epilepticus lead to epilepsy has been a subject of debate. Whereas prospective epidemiological evaluations have provided little evidence for epileptogenesis,16,45,46 retrospective analyses have strongly linked a history of complex, and particularly of prolonged, febrile seizures to temporal lobe epilepsy,58–61