Factors affecting anesthetic gas uptake
Solubility
The solubility of an anesthetic agent is defined by its blood-gas partition coefficient. It describes the relative affinity of an inhaled anesthetic agent for the blood. For example, isoflurane has a blood-gas partition coefficient of 1.4. This means that, at equilibrium, the isoflurane concentration in the blood would be 1.4 times the concentration in the gas (alveolar) phase. By definition, the partial pressures of the agent in blood and gas are identical at equilibrium, but the blood would contain more isoflurane. The blood-gas partition coefficients of commonly used inhalation anesthetic agents are listed in Table 61-1.
Table 61-1
Partition Coefficients at 37°C
Anesthetic Agent | Blood-Gas Partition Coefficient |
Desflurane | 0.45 |
Nitrous oxide | 0.47 |
Sevoflurane | 0.65 |
Isoflurane | 1.4 |
Enflurane | 1.8 |
Halothane | 2.5 |
Diethyl ether | 12.0 |
Methoxyflurane | 15.0 |
Modified from Eger EI II. Effect of inspired anesthetic concentration on the rate of rise of alveolar concentration. Anesthesiology. 1963;24:153-157.
The higher the blood-gas partition coefficient, the greater the amount of anesthetic agent dissolved in blood at equilibrium, and onset of anesthesia is delayed because it is not the total amount of drug in the blood, but the partial pressure of inhalation agent in the blood and, therefore, in the brain that induces anesthesia. For agents with a high coefficient, it takes a relatively long time to “fill the tank” before the partial pressure begins to rise high enough to induce anesthesia. Gases with a high coefficient, because the gas diffuses so quickly into blood, have a relatively low alveolar/inspired gas ratio (FA/FI) (Figure 61-1), which also delays onset. Uptake of the more soluble inhalation agents can be increased by anesthetic overpressuring, that is, delivering a concentration of inspired gas two to four times the MAC (minimum alveolar concentration).
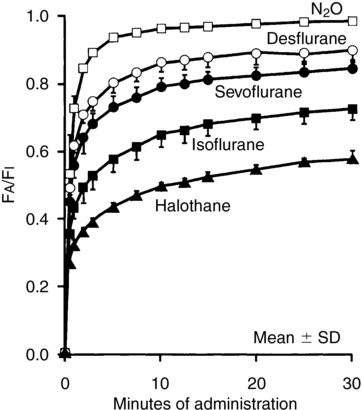