11 Extraaxial Brain Tumors
Introduction
Extraaxial brain tumors (EBTs) include an array of tumors that arise from structures and tissues directly adjacent to the brain, including the meninges, nerve sheaths, and the pituitary gland, which give rise to meningiomas, schwannomas, and pituitary adenomas, respectively. As a group, they account for over 50% of all brain tumors diagnosed in the United States and, therefore, a large proportion of brain tumors seen by neurologists and neurosurgeons.1 In fact, meningiomas (which account for 32.1% of all brain tumors) are the most common brain tumor diagnosed in patients greater than 34 years of age and pituitary adenomas (8.4% of all brain tumors) are the most common brain tumor diagnosed in patients between 20 and 34 years of age.1 While these tumors are usually benign, they can be associated with significant morbidity and, rarely, mortality, because of their prevalence and occasionally malignant behavior. In this chapter, we discuss the current management of extraaxial brain tumors, with particular emphasis on the three major types of EBTs, including meningiomas, schwanommas, and pituitary adenomas. General principles of diagnosis, management, and treatment can be extrapolated to other EBTs, such as craniopharygiomas and chordomas. We consider recent advances in the diagnosis and treatment of these tumors that have either impacted or may prospectively impact the management of patients.
Incidence and epidemiology
EBTs account for three out of the four most common primary brain tumors diagnosed in the United States. According to the Central Brain Tumor Registry of the United States (CBTRUS), the incidence of EBTs is 8.3 cases per 100,000 person-years.1 Meningiomas, with an incidence of 5.3 per 100,000 person-years, are the single most common brain tumor histology diagnosed in the United States, occurring in nearly twice as many patients as high-grade gliomas. The incidence of meningiomas is 2.2 times greater in females than males. Despite their classical presentation in middle-aged women, the incidence steadily increases with age (i.e., greatest incidence in the 85+ age group). While there is no reported racial predilection in the United States, there is an increased incidence in the Polynesian population, who more frequently have multiple and larger tumors than other populations.2
Nerve sheath tumors and pituitary tumors are the third and fourth most common brain tumors after meningiomas and glioblastoma (accounting for 9.0% and 8.4% of brain tumors, respectively). Although these tumors are the most common brain tumors diagnosed in patients 20 to 34 years of age, their incidence actually peaks in the 65 to 74-year-old age group. Neither tumor has different gender-specific incidence rates, but pituitary tumors are significantly more likely to be diagnosed in blacks, whereas vestibular schwannomas are significantly more likely to be identified in whites.1
Like gliomas, the incidence of EBTs has increased steadily over the last decade, presumably due to an increased rate of diagnosis rather than a true increase in incidence.3 The increased availability and use of neuroimaging modalities have significantly increased the detection of incidental intracranial pathologies, including meningiomas and vestibular schwannomas.4 The increased incidence is also partially attributed to an increased willingness of the medical community to pursue a diagnosis and treatment in older patients.5
Many risk factors have been suggested for EBTs. Of these, radiation exposure is the only universally accepted factor placing people at risk for meningioma induction.6 This concept gained wide acceptance after Modan and colleagues retrospectively discovered a four-fold increase in the incidence of meningiomas among children treated with the Kienbock-Adamson protocol for tinea capitis, a low dose radiation treatment targeting the scalp.7 Low-dose radiation-induced meningiomas are those associated with exposure to less than 10 Gy, but meningiomas have been induced by as little as 1 to 2 Gy. Higher radiation doses are associated with a decrease in latency of meningioma induction.8
Other risk factors for meningiomas have also been explored. One of the early postulates was that head injury caused meningiomas. Associations between head trauma (especially in young males 10 to 19 years of age) and meningiomas have been reported, with a latency of 15 to 24 years.9,10 The evidence supporting this hypothesis is inconsistent and the seemingly conflicting data leaves no definitive proof of a causal relationship between head injury and the subsequent development of meningiomas. The female predilection of meningiomas suggests that female sex hormones may also be a risk factor for tumorigenesis. This theory was bolstered by the identification of estrogen and progesterone receptors on subsets of meningiomas. Tumors expressing progesterone receptors (PR+) behave in a more benign clinical fashion and are less likely to recur. Those expressing estrogen receptors or lacking progesterone receptors display more frequent genotypic alterations and karyotype abnormalities consistent with more aggressive meningiomas.11 Despite these findings, the evidence that exogenous hormones affect tumor frequency is mixed. Blitshteyn and colleagues retrospectively reviewed records from 355,318 women at the Mayo Clinic between 1993 and 2003 and found that women with either current or past hormone replacement therapy (HRT) had a 2.2-fold risk of developing meningiomas.12 A much smaller population-based case-control study failed to find such an association.13 The fact that receptor status affects gene expression profiles, particularly of those near the NF2 gene locus, which has been implicated in meningioma tumorigenesis, suggests that further examination into the effect of female sex hormones may be warranted.14 Increased body mass index (BMI) has also been associated with a greater incidence of meningiomas.15,16 The association between increased BMI and meningioma incidence may be mediated by aromatase in adipose tissue which increases circulating estrogen levels.
Recently, many studies have studied the impact of mobile phone use on tumor incidence. Hardell and colleagues performed a meta-analysis of such studies and concluded that there is a slight statistically significant increased risk for development of glioma (odds ratio [OR] = 2.0, 95% confidence interval [CI] = 1.2 to 3.4) and vestibular schwanomma (OR = 2.4, 95% CI = 1.1 to 5.3), but not meningiomas, using a greater than or equal to 10 years latency period.17
Presentation
The precise symptomatology with which a tumor presents depends on its location and size. The most common locations of meningiomas in descending order of frequency are: convexity, parasagittal, sphenoid and middle cranial fossa, frontal base and posterior fossa, cerebellar convexity, cerebellopontine angle, intraventricular, and clivus. Schwannomas arise most commonly from the vestibular component of the vestibulocochlear nerve (>90%), sensory division of the trigeminal nerve (1% to 10%), facial nerve (1%), nerves of the jugular foramen (glossopharyngeal, vagus, and spinal accessory nerve), hypoglossal nerve, extraocular nerves, and the olfactory nerve. The various syndromes with which EBTs present are outlined in Tables 11-1 through 11-3.
TABLE 11-1 Meningioma Location and Associated Typical Clinical Presentations
Parasagittal and falcine meningiomas | Anterior 1/3 | Headache and mental status changes |
Middle 1/3 | Jacksonian seizures and progressive hemiparesis | |
Posterior 1/3 | ||
Headache, visual symptoms, seizures, or mental status changes | ||
Sphenoid wing meningiomas | Lateral / pterional | Similar to convexity tumors |
Middle 1/3 (alar) | Hemiparesis / dysphasia | |
Medial (clinoidal) | Visual acuity/field disturbance due to optic nerve compression, proptosis, cranial nerve dysfunction (III,IV,V,VI) | |
Olfactory groove | Foster-Kennedy syndrome (anosmia, ipsilateral optic atrophy with contralateral papilledema), frontal lobe syndromes / mental status changes, urinary incontinence, seizure | |
Tuberculum sella / suprasellar | Visual acuity/field disturbance, anosmia, hydrocephalus, endocrinologic syndromes | |
Cavernous sinus | Cranial nerve deficits (III,IV,V,VI) | |
Cerebellopontine angle | Hearing loss, facial pain / numbness / weakness / spasm, headaches, cerebellar signs | |
Foramen magnum | Unilateral cervical pain, extremity motor and sensory loss (clockwise involvement), cold and clumsy hands with intrinsic hand atrophy | |
Petroclival | Hearing loss, vertigo, tinnitus, facial pain, diplopia, cranial nerve deficits (V,VI,VII,VII) |
TABLE 11-2 Intracranial Schwannomas: Typical Clinical Presentation
Vestibular | Unilateral sensory hearing loss, tinnitus, disequilibrium |
Trigeminal | Trigeminal nerve dysfunction (numbness, pain), headache, diplopia, hearing loss/tinnitus |
Facial | Hearing loss, facial paralysis (may be acute), facial pain, hemifacial spasm, tinnitus, vertigo |
Jugular foramen | Cranial nerve palsies (IX,X,XI) |
Accessory nerve | Chronic neck and shoulder pain, muscle spasms |
Hypoglossal | Headache, cranial nerve dysfunction (IX,X,XI), limb weakness |
TABLE 11-3 Pituitary Adenomas: Typical Clinical Presentation
Nonfunctioning adenoma | Headache, bitemporal hemianopia, diplopia, dysmenorrhea, fatigue |
Prolactinoma | Amenorrhea, lactation, impotence |
Cushing disease | Weight gain, hypertension, diabetes mellitus, moon facies, supraclavicular fat pads, central obesity, facial plethora, abdominal striae, muscle weakness, osteoporosis |
Acromegaly | Increasing hand/foot size, prominent brow/jaw, diabetes mellitus, carpal tunnel syndrome |
TSH-secreting adenoma | Signs and symptoms consistent with hyperthyroidism |
Diagnosis
MENINGIOMAS
The classic radiographic features of meningiomas include presence of a broad dural base, dural tails, diffuse contrast enhancement, and the presence of an arachnoid plane.18 These criteria can help distinguish between a CP angle meningioma and a vestibular schwannoma (Figure 11-1). Using these classic criteria, MRI has a sensitivity and specificity of 98% and 97%, respectively. However, the sensitivity and positive-predictive value of conventional MRI drops significantly in high-grade (i.e., atypical and malignant) meningiomas.19 MR spectroscopy, which displays distinct peaks for various intratumoral metabolites including choline, creatine, N-acetyl-aspartate (NAA), and lactate, can more definitively identify meningiomas by identifying an alanine peak (unique to meningiomas) and an increased glutamate/creatine ratio.20 Moreover, atypical meningiomas (WHO grade II) are much more likely to have a lactate peak (a marker of proliferation) than WHO grade I tumors.21
SCHWANNOMAS
The imaging evaluation of schwannomas includes conventional MR sequences with contrast. Like meningiomas, schwanommas are extraaxial lesions that enhance diffusely. In contrast, schwannomas generally do not have dural tails but rather follow the course of cranial nerves along the skull base, such as into the internal auditory meatus and Meckel’s cave (Figure 11-2). Besides imaging appearance, brainstem auditory evoked responses (BAERs) are a critical diagnostic test for management planning. While for large tumors with brainstem compression it is nearly impossible to preserve hearing, with smaller tumors there is a great interest to preserve functional hearing.
PITUITARY TUMORS
Pituitary tumors are the most common tumor of the sellar region, accounting for over 90% of such lesions. Unlike other EBTs, because of the hypervascularity of the pituitary gland, microadenomas (i.e., pituitary adenomas < 10mm) are often identified by their lack of contrast enhancement while the remainder of the gland enhances briskly (Figure 11-3). Still, in some cases, especially in Cushing disease, an adenoma cannot be identified on MRI despite biochemical evidence of an ACTH-secreting pituitary adenoma. Dynamic pituitary MRI, which uses multiple sequential image acquisition following gadolinium intravenous contrast, significantly increases adenoma detection to nearly 100%, a rate much higher than the 50% to 60% rate reported for nondynamic MRI.22
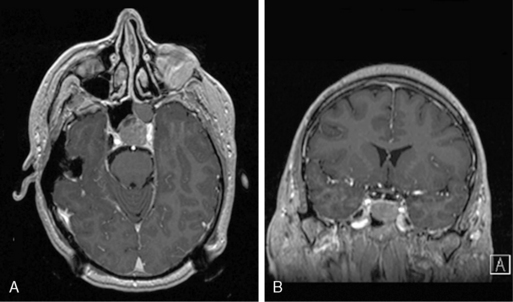
Figure 11-3 (A) and coronal (B) T1-weighted postcontrast MRIs of a patient with a nonfunctioning pituitary adenoma.
In addition to imaging, all patients with sellar or suprasellar lesions need thorough biochemical evaluation of their hypothalamic-pituitary axis, which can provide insight into the nature of an otherwise seemingly nonfunctional pituitary tumor. In addition to biochemical evaluation of circulating hormone levels in the peripheral blood, inferior petrosal sinus sampling (IPSS) has become an integral part of the evaluation of patients with suspected Cushing disease. Comparing central-to-peripheral ratios of ACTH levels at baseline and in response to corticotrophin releasing hormone (CRH) (≥ 2.0 and ≥ 3.0, respectively) is very sensitive for confirming a “central” etiology for Cushing syndrome, or Cushing disease.23
Management
OBSERVATION
Expectant management potentially provides patients with an overall improved quality of life (QOL) for the duration of the disease by not exposing them to the morbidity associated with other treatment paradigms.24 The morbidity of surgery can be quite significant; in one study, among patients older than age 70 years who underwent operation for asymptomatic meningioma, the neurological morbidity rate was 23.3%.25 Expectant management is therefore particularly pertinent to older patients with incidentally discovered and asymptomatic tumors.
The majority of incidental meningiomas show minimal growth. This is particularly true for heavily calcified meninigiomas. Thus, they may be observed without surgical intervention unless specific symptoms appear. Tumor growth is associated with patient age, with tumors in younger patients having a shorter doubling time than in older patients. While radiological features, such as calcification or T2 signal hypointensity or isointensity, may predict decreased growth potential, initial tumor size does not correlate with growth rate.26 Despite minimal growth in most tumors, asymptomatic tumors must be followed: in one study of 40 patients with incidental meningiomas, 33% of tumors grew during a mean follow-up of 32 months, and 36% of patients had symptomatic progression.27
Like incidental meningiomas, there is a place for expectant management of schwannomas. Small and medium-sized vestibular schwannomas that are found incidentally (accounting for approximately 10% of all vestibular schwannomas and found in up to 0.02% of the general population) may have a more benign nature and be less likely to require intervention.28,29 In one series, conservatively managed schwannomas either did not grow or regressed in 42% of patients and had an overall average growth rate of 0.91 mm per year.30 Conservative management can be successful in up to 85% of patients selected for expectant management.31 First-year growth rate is an good predictor of future growth, and therefore must be monitored, if tumors are to be managed conservatively, to determine whether intervention will be necessary.32 The indications for intervention should be based on a combination of rapid tumor growth with the development of symptoms.30 In patients older than 65 years with vestibular schwannomas, these tumors may not require surgical intervention.
Incidental pituitary tumors, or pituitary “incidentalomas,” can also be managed without surgical intervention. Patients with pituitary incidentalomas usually follow a benign course for at least 6 years, not requiring neurosurgical intervention as long as clinical observation is continued.33 Generally, only those that are greater than 10 mm (macroadenomas) enlarge or cause complications and may require closer clinical observation or upfront surgery. Regardless of size, patients who are treated conservatively should undergo biochemical assessment and ophthalmological examination, since occult endocrine dysfunction or visual field defects may be present at the time a pituitary incidentaloma is detected.33–35
MICROSURGICAL RESECTION
Meningiomas
The importance of complete surgical excision for meningiomas has been well documented for over 50 years. In 1957, Simpson retrospectively reviewed the postoperative course of 265 patients with meningiomas, 55 of whom experienced recurrences (21%). Patients with a gross total resection of tumor, dural attachments, and abnormal bone (grade I excision) had a recurrence rate of 9%, those with gross total excision with coagulation of dural attachments (grade II) had a recurrence rate of 19%, those with gross total excision without coagulation of dural attachments (grade III) had a recurrent rate of 29%, and those with partial resection (grade IV) had a recurrence rate of 44%.36 These recurrence rates most likely are underestimates since this study was conducted in the pre-CT and pre-MRI era. Nonetheless, these findings highlight the fact that surgical excision is the most important factor in the prevention of recurrence. Tumors that cannot be totally excised because of their adjacency to critical structures such as cranial nerves and sinuses (e.g., medial sphenoid wing, petroclival, clinoidal, and tentorial-based tumors, and posterior parasagittal lesions, respectively) therefore are at highest risk for tumor recurrence. The highest recurrence rates are found for patients with sphenoid wing meningiomas(>20%), parasagittal meningiomas (8% to 24%), and suprasellar meningiomas (5% to 10%). In contrast, convexity meningiomas, which are relatively easily excisable, have reported recurrence-free rates at 5,10, and 15 years of 93%, 80%, and 68%, respectively.37 Other risk factors for recurrence include histopathologic findings of increased mitosis/Ki-67 labeling index, focal necrosis, nuclear pleomorphism, prominent nucleoli, syncytial tumors, the presence of brain invasion, and loss of 1p36.1-p34.38,39 Interestingly, “high-risk tumors” occur more frequently at the brain surface than at the cranial base, suggesting that the tendency of cranial base meningiomas to recur depends on surgical rather than biological factors.40
The morbidity of microsurgical excision, like extent of resection, is intimately related to tumor location. Easily accessible tumors, such as convexity, lateral and middle third sphenoid wing meningiomas, and anterior third parasagittal and falcine meningiomas, are amenable to complete resection and associated with low morbidity (10% of patients with neurologic sequelae) and mortality (0 to 3%). Neurologic sequelae associated with these resections typically manifest secondary to compromise of adjacent cerebrovascular structures, immediate postoperative edema, and epilepsy. Tumors of the skull base, tentorium, foramen magnum, and other difficult locations are associated with significantly higher morbidity and mortality due to associations with cranial nerves and proximal cerebral vessels. Permanent neurologic deficit ascribed to cranial nerve dysfunction has been reported in a wide range (18% to 86%).41 The highest of these complication rates are typically associated with petroclival and cavernous sinus meningiomas, especially in cases where a complete resection is performed. Preoperative embolization has led to decreased morbidity in patients in whom the tumor blood supply may be difficult to access at the time of surgery.
Despite improvements in microsurgical techniques, image guidance and perioperative critical/medical care, mortality rates in large series remain at 1% to 14%.42,43 Factors increasing mortality include poor preoperative clinical condition, compressive symptoms from tumor, older age, incomplete tumor removal, pulmonary embolism, and intracranial hemorrhage.44
Vestibular Schwannomas
The introduction of the operating microscope, more sensitive diagnostic imaging, and intraoperative facial and cochlear monitoring have steadily decreased the morbidity and mortality associated with resection of vestibular schwannomas. In a meta-analysis of 16 studies including 5005 patients undergoing microsurgery for sporadic unilateral vestibular schwannomas, tumor resection was complete in 96% of cases, with a mortality rate of 0.63%. The most common nonneurologic complication was cerebrospinal fluid leak, which occurred in 6.0% of patients.45 The challenge of surgical resection lies in preserving facial nerve and auditory function. Detailed evaluation of individual large series shows preservation of facial nerve function is inversely proportional to tumor size. Indeed, when evaluating facial nerve preservation after resection of intracanalicular lesions alone, multiple studies have reported 100% postoperative grade I House-Brackmann function.46,47 Resection of small tumors (<2.0 cm), medium sized tumors (2.0 to 3.9 cm), and large tumors (>4.0 cm) is associated with a 95%−97%, 61%−73%, and 28%−57% preservation of grade I or II House-Brackmann function, respectively.48–50 The suboccipital and translabyrinthine approaches afford comparable and excellent results as compared to the middle fossa approach in which increased manipulation of the superiorly located facial nerve in the internal auditory canal may account for a higher risk to facial nerve function.