Chapter 168 Explant Analysis of Wear, Degradation, and Fatigue in Motion Preserving Spinal Implants
Because of their rarity, certain failure modes may escape detection in a clinical trial. For this reason, the U.S. Food and Drug Administration (FDA) is keenly interested in the detailed analysis of explanted implants, as evidenced by the inquiry and recommendations of three FDA panels for cervical and lumbar artificial discs from 2004 to 2007. In the 2007 FDA panel meeting for the Bryan cervical disc (Medtronic, Memphis, TN), one of the conditions for approval was that the manufacturer conduct a 10-year postmarket retrieval analysis of the device (www.fda.gov/cdrh/meetings/071707-summary.html). Retrieval analysis is considered an absolutely essential companion activity not only for prospective, randomized clinical trials but also for the postmarket surveillance of new spinal implant designs.
Retrieval analysis has strong regulatory and societal implications and is performed by clinicians, engineers, and biologists. There are great advantages from their collaboration as a team, which allows for the relevant patient-related, surgeon-related, and implant-related factors to be considered in as broad a context as possible. When properly performed by experienced investigators, retrieval analysis can provide a definitive source of information on implant-related and biomaterial-related failure modes, biocompatibility, and their impact on the overall longevity of the surgical procedure. When compared with the findings of experimental or numerical studies, the results of retrieval analysis can help validate preclinical testing and computer-based methods that are essential when evaluating new designs. Taken one step further, when the aggregate results of retrieval analysis are integrated into the implant design process, the technique can provide feedback or motivation for adjusting implant designs or finding alternative biomaterials. As explained in a consensus statement from the National Institutes of Health,1 “Technology progresses by facing its failures and learning from its successes. The goal of device research and development is to improve patient care through improvement of implants. A fundamental objective is to understand successful implants and assess failures through retrieval analysis.”
There is also strong educational and ethical motivation for participating in retrieval analysis. When involving researchers, engineers, and clinicians in the early stages of their careers, retrieval analysis has a major educational component. Clinicians who are actively implanting motion preserving devices have an ethical responsibility to contribute to retrieval analysis to provide their patients with the best care possible. The removal of an implant, especially a lumbar artificial disc, exposes the patient to increased risk of serious, potentially life-threatening complications. The clinician has a responsibility to ensure that implanted and explanted devices and tissues are properly analyzed and the results disseminated to stakeholders. There are many scientific, educational, and ethical reasons why the research community, implant designers, and surgeons are motivated to participate in retrieval analyses. These reasons have been touched on in previous review articles and book chapters.2 It is a testament to the varied and compelling motivations for retrieval analysis that it continues to play an important role in the evolution of implant technology.
Practical Aspects of Retrieval Analysis
Retrieval Program
A retrieval program may be organized as the collection and analysis of implants from a single institution or from a multi-institutional study or independently as a more generalized retrieval repository. When a retrieval program is established as part of a clinical study, institutional review board approval or the equivalent should be obtained, which may require the informed consent of each patient to participate. A clinical study–type design is preferred when detailed clinical information, including protected health information, is being collected and analyzed as part of the study. Details on the design and establishment of a retrieval or repository program have been outlined previously.3
To ensure adequate receipt and processing of the retrieved implants, standard precautions should be used when handling the explanted components until they have completed a cleaning protocol. These precautions may include keeping the implant components in appropriate chemicals to preserve adhering tissue (e.g., formalin), provided that the preservative does not degrade the component itself. At our center, implants fabricated from titanium alloy, cobalt chromium (CoCr) alloy, and polyethylene are ultrasonically cleaned in soap and deionized water, rinsed, and sterilized using a 10% bleach solution. We omit the bleach solution for cleaning stainless steel implants because there is the potential for corrosion. Likewise, a mild cleanser is used for implant components composed of polymers that may be vulnerable to chemical changes from a bleach solution. Additional guidance for handling of retrieved implants and tissues may be found in American Society for Testing and Materials (ASTM) Standard F561.4
The analysis of retrieved implants and tissues may involve a broad range of test methods, which are comprehensively described in ASTM Standard F561.4 This manual provides guidance for analysis of all implant components, including metallic, polymeric, and ceramic materials. Although a detailed summary of this standard is beyond the scope of this chapter, we highlight in subsequent sections specific test methods that have been particularly helpful in the characterization of retrieved metal-on-polyethylene and metal-on-metal disc replacements.
Wear and Damage Assessment
Because wear and damage of retrieved TDRs can occur at length scales that are invisible to the naked eye, microscopy may be necessary to identify damage modes. An optical stereomicroscope, with 10× to 40× magnification, is typically sufficient to identify worn regions of retrieved implants, but frequently it is helpful to analyze the wear surfaces using scanning electron microscopy (SEM), achieving magnifications of 5000× or greater. In addition to optical and SEM, micro-CT and white light interferometry (WLIR) are two methods that have proven particularly useful in our previous analyses of wear in retrieved TDR and dynamic stabilization components.3,5–7 These novel wear assessment methods are highlighted in this section.
Micro-CT Analysis
We have used a micro-CT to detect nondestructively surface and internal voids and cracks within retrieved polyethylene TDR components5 and polyurethane motion preserving spinal implants.7 Depending on their thickness, CoCr alloy components produce substantial artifacts on micro-CT. Consequently, we have found it helpful to remove the wire marker before micro-CT analysis of rim wear in the Charité design. The removal of the wire marker for other analyses may be unnecessary because the artifact does not extend into the central core of the implant. For polymer components that do not incorporate wire markers (e.g., ProDisc [Synthes, Paoli, PA] or Dynesys [Zimmer Spine, Minneapolis, MN]), micro-CT artifacts are not an issue.
At the authors’ institution, polymer components of motion preserving spinal implants are scanned at 18 μm voxel resolution using a commercial micro-CT scanner (μCT80, Scanco Medical, Bassersdorf, Switzerland).5 The three-dimensional reconstructions of the component and two-dimensional sections taken through the component are evaluated for the presence of surface, through-thickness, and internal voids or cracks. Previously, we have observed the trajectory of cracks in polymer components of motion preserving spinal implants, including Dynesys.7 Using optical microscopy, we have also characterized permanent deformation and wear patterns of polymer components from motion preserving spinal devices. Using these methods, we have been able to distinguish these forms of surface and subsurface damage from iatrogenic damage that occurs during implant removal.
Because of attenuation artifacts encountered with metallic components, micro-CT is useful only for polymeric components from TDRs. To measure the macroscopic surface geometry of metallic components, tools employed by the research community include coordinate measurement machines, laser profilometers, and optical profilometers. To measure microscopic changes in the implant surface, WLIR may be used. As discussed next, interferometry is applicable to both metallic and polymeric components of disc replacements.
White Light Interferometry
We use WLIR to characterize the microscopic surface morphology of retrieved disc arthroplasty components. WLIR is capable of detecting surface height changes that are on the nanometer length scale by measuring the interference of white light reflected off the component within a specified field of view compared with the light from a reference beam. We have successfully analyzed the wear surfaces of polyethylene and CoCr alloy TDRs at our institution using a NewView 5000 equipped with advanced texture analysis software (Zygo, Middlefield, CT).6 We sample 5 to 10 square regions (typically 0.54 × 0.72 mm) of a component to obtain representative surface topography of the retrieved implant in both worn and unworn locations.
Figures 168-1A and B illustrate the surface topography obtained from unworn and worn surface regions of a retrieved polyethylene disc replacement component that was implanted for 12.7 years. The unworn polyethylene surface is dominated by machining marks that are on the order of several microns in amplitude (see Fig. 168-1A). Initially, microscopic evidence of adhesive and abrasive wear is detected by the erosion and removal of machining marks, along with the presence of fine scratches (see Fig. 168-1B).
The surface topography of a retrieved CoCr alloy disc replacement is shown in Figures 168-1C and D. The unworn CoCr surface is usually relatively flat and featureless, aside from microscopic scratches generated during the final polishing stage of the manufacturing process (see Fig. 168-1C). In a region of wear, the CoCr surface has evidence of localized, microscopic scratches with a characteristic length scale that is larger than the residual features from polishing (Fig. 168-1D).
As shown in Figure 168-1, surface characterization using WLIR provides useful information about the wear mechanisms in TDRs. In addition, by quantitatively analyzing the surface data, the roughness and waviness can be quantified and compared with as-manufactured components, providing insight into the magnitude of surface changes or wear that occur in vivo.8–10 Ultimately, quantitative measurements of implant surfaces are used to validate in vitro and computational models that seek to simulate in vivo wear processes.
Wear and Damage Mechanisms
As discussed in the previous section, metal and polymeric components from retrieved motion preserving spinal devices should be evaluated macroscopically and microscopically for the presence of damage modes typically observed in large joint arthroplasty components (e.g., burnishing, abrasion, scratching, pitting, plastic deformation, fracture, fatigue damage, and embedded debris). A detailed description of wear and wear mechanisms is beyond the scope of this chapter and can be found in books dedicated to this subject.11 A generic guide for the analysis of retrieved components is summarized in the Appendices for ASTM Standard F561.4 This section provides a concise summary of the most relevant wear and fatigue damage modes that may be encountered when inspecting retrieved motion preserving spinal implant components.
Abrasion and Scratching
Abrasive wear is evidenced by scratching and is common to both metallic and polymeric components for TDR. Abrasion may be apparent macroscopically by the naked eye, or it may be apparent only when viewed using microscopy. Abrasive wear occurs when microscopic surface irregularities (also referred to as “asperities”) in one implant scratch the surface of the opposing counterface. In the case of metal-on-polyethylene, the asperities on the metallic implant produce scratches in the softer polymeric implant. In the case of CoCr alloy metal-on-metal implants, abrasive wear is produced by locally stiffer asperities, such as carbides, plowing through the relatively softer cobalt alloy matrix. Abrasive wear can also occur when softer polymeric components of the implant contact surrounding bony structures (Fig. 168-2).
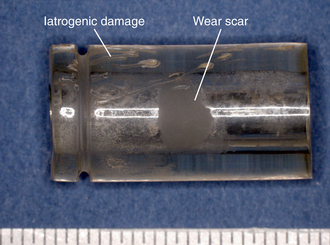
FIGURE 168-2 Abrasive wear observed on polymeric components from retrieved Dynesys systems, implanted 1.1 years.
During retrieval analysis, the pattern of scratches on an implant, whether macroscopic or microscopic, provides clues to the kinematics (motion) of the surfaces while they were contact in vivo. The microscopic multidirectional scratches and crisscrossing wear paths at the dome of a retrieved polyethylene TDR (Fig. 168-3) are consistent with the type of microscopic abrasive wear mechanisms previously observed in retrieved hip replacement components.12,13 By matching comparable regions of damage on two opposing bearing surfaces, it is further possible to infer the orientation of the components while they were in contact.
Burnishing
Typically encountered with polyethylene disc components, burnishing gives the polymer surface a polished, glossy appearance (Fig. 168-4). At a microscopic length scale, burnishing is associated with an adhesive wear mechanism, whereby the polyethylene surface wear occurs by adhesion to the metallic counterface. Magnified images of a burnished wear zone from a retrieved TDR are shown in Figure 168-4 and as noted also show evidence of scratching, which denotes the presence of abrasion. For this reason, the dominant wear mechanism in metal-on-polyethylene articulations is considered to be a combination of adhesion and abrasion, as seen in total joint replacements.12,13
Surface Deformation
In motion preserving spinal devices, macroscopic surface deformation has been observed in components that undergo compression in vivo. Polycarbonate urethane (PCU) spacers used in the Dynesys system undergo deformation owing to cold flow of the material, the compressive load applied during the surgery, and subsequent loading in vivo, which results in permanent bending of the implant and indentations from the supporting polyaxial screws (Fig. 168-5). Additional deformation of soft polymer components may occur from interaction with other components from the implant, for example, the cord component that passes through the center of the polyurethane spacer in the Dynesys system (see Fig. 168-5).
Fatigue Wear and Fracture
Fatigue wear and fracture, especially of the rim, are a concern with polyethylene TDRs. David et al.14 reported a case in which the entire rim of a disc replacement fractured from the central body of the core after 9.5 years in vivo. This case of rim failure was attributed to severe oxidation degradation after gamma sterilization in air.
The severity and clinical manifestation of fatigue-related rim damage in the Charité design varies widely, ranging from full-thickness transverse rim fracture (Fig. 168-6) to more benign radial crack formation (Fig. 168-7). In our retrieval studies of the Charité, radial cracks have been observed in 27 of 53 implants examined so far.15 Similarly, transverse cracks have been observed in 17 of 53 retrieved implants.15 In most cases, fatigue fracture is related to impingement by the metallic end plates. Fractures have also been observed in polymer components of dorsal devices such as the Dynesys (Fig. 168-8).
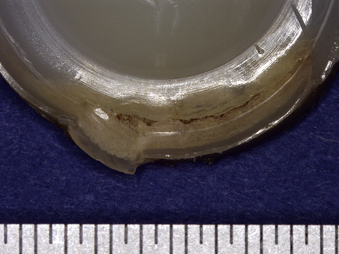
FIGURE 168-6 Fatigue-related rim fracture observed in a retrieved Charité implant that was implanted for 9.2 years.
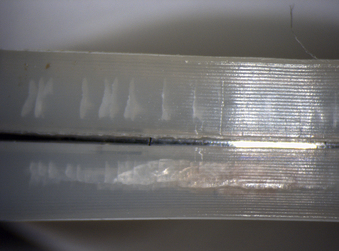
FIGURE 168-7 Fatigue-related radial rim cracking observed in a retrieved Charité prosthesis that was implanted for 3.1 years.
Embedded Debris
Embedded debris is an unusual but noteworthy damage mode for disc replacements. We have observed embedded debris in which a fractured radiographic wire marker became trapped between the rim and a metallic end plate of a TDR (Fig. 168-9). We have also observed embedded polymeric debris in dynamic systems with braided polymer cords (Fig. 168-10). The clinical significance of this wear mode is unknown at the present time. In large total joint replacements, embedded debris is a potential roughening mechanism for the metallic component, which can result in accelerated wear. Such a mechanism was not apparent in the retrieval shown in Figure 168-9, which appeared to be relatively stationary and resulted in only a faintly perceptible indentation of the metallic end plate. As a result, additional retrievals are necessary to understand better the incidence and clinical significance (if any) of embedded debris in TDRs. Although metallic surfaces are also theoretically susceptible to embedded debris, including third-body scratching by the radiopacifiers contained in bone cement for total joint applications, there are no reports yet in the literature of third-body wear being observed in metal-on-metal disc replacements.
Corrosion
Prior retrieval studies of dorsal instrumentation systems provide evidence that corrosion can occur in metallic spinal implants. Villarraga et al.16 reported findings from retrieved posterior rod instrumentation systems. Wear and corrosion were not observed in any of the 21 titanium implants that were evaluated, but corrosion and wear were observed in 58% of stainless steel implants included in the study. A common location for corrosion was where the transverse connector interfaces with the rod, which can involve multiple surface-to-surface contact points and small cavities where no contact occurs.16 These characteristics are inherently associated with micromotion, which can lead to wear, fretting corrosion, and wear particle formation. Corrosion of stainless steel components has also been reported in previous retrieval studies of spinal implants17–19 to worsen the fatigue performance of orthopaedic implants,20 and corrosion by-products are suspected to cause deleterious biologic tissue responses in the adjacent tissue surrounding the implant.19,21
Experience gained from dorsal spinal fusion instrumentation is important to keep in mind in evaluating TDR and other nonfusion spinal implant designs. Although retrieved motion preserving implant components from our laboratory and others22 have not shown evidence of corrosion, the effects of wear or corrosion debris on local and systemic tissues remain clinical concerns.23 Future long-term retrieval studies are needed to determine whether this remains a concern with contemporary motion preserving implant designs.
In Vivo Degradation
Although characterization of wear and damage mechanisms is perhaps one of the most fruitful goals of retrieval analysis, it is also equally important to investigate whether the biologic environment has resulted in any long-term chemical changes to the implant material, whether it be composed of polymer, metal, or ceramic. With polyethylene components, in vivo oxidation may be a potential long-term damage mechanism for artificial discs.5 However, in vivo chemical changes to implants may be incidental and unrelated to clinical performance. For polyethylene acetabular components, severe rim oxidation has been shown to occur after 10 years in vivo,24 but the clinical relevance is unclear because these implants do not normally articulate at the rim. With polyethylene TDR components, rim failure has been observed to occur in vivo, but it is unclear if oxidation is the driving mechanism in all of these cases, or whether impingement alone may be sufficient to generate the types of fractures that have been documented to occur clinically.3 In PCU components, surface chemical changes associated with polyurethane degradation have been observed; these changes were associated only with implants that have been implanted for relatively long periods and primarily in regions where the implant was exposed to biologic fluid.7 Trommsdorff et al.25 reported that chemical changes in retrieved Dynesys PCU spacers were negligible at 100 μm below the surface. The clinical relevance of these surface chemical changes is unknown.
In vivo changes in chemistry may also occur with metallic components. In metal-on-metal hip implants fabricated from CoCr alloys, tribochemical deposits have been observed on the surface of retrieved implants.26 These surface layers rich in carbon and oxygen, which have a smoky or hazy appearance, are attributed to joint fluids, which become fused to the bearing surface. The biofilms are thought to have a beneficial effect, by providing a solid lubricant for the articulating surface. We have observed comparable biofilms on retrieved CoCr alloy, metal-on-metal disc replacement components, suggesting that a similar mechanism may be occurring.27
A wide range of well-established techniques has been developed to assess chemical changes in polyethylene and metallic components for motion preserving spinal implants; a comprehensive list is provided by ASTM F561-05.4 With polyethylene components, preferred methods include characterization of crystalline content using differential scanning calorimetry, measurement of oxidation using Fourier transform infrared spectroscopy (FTIR), and measurement of mechanical properties using the small punch test (ASTM F2183-02). In previous case studies of polyethylene disc replacements, both FTIR and the small punch test have been successfully employed.5,14
For metallic components, electron dispersive x-ray spectroscopy combined with SEM is useful for characterizing the chemistry of the alloys and biologic surface layers. As previously mentioned, we have successfully employed electron dispersive x-ray spectroscopy to analyze biofilms on the surface of retrieved metal-on-metal implants fabricated from CoCr alloys.27 These electron dispersive x-ray spectroscopy analyses have enabled us to confirm that carbon-rich and oxygen-rich tribochemical reactions can occur on both the concave and the convex sides of metal-on-metal articulations in the spine. Further studies with an additional number of retrieved implants are necessary to determine the incidence of biofilms on CoCr alloy implants and for disc replacements produced from stainless steel or metalloceramic alloy composites.
Review of the Literature on Retrieval Analysis
At the present time, the literature regarding retrieval analysis of motion preserving spinal implants includes conference abstracts and individual case studies5,14,28,29 but relatively few published journal articles with larger series.6,15,30 In this section, we focus on studies that have included the analysis of a retrieval collection to seek commonalities in implant performance. We have included journal articles and more recent studies from conference proceedings in our review of retrieval analyses for motion preserving implants. Because the conference proceedings may not be readily available to all readers, we have also provided details about the findings from conference abstracts.
Cervical Spine Total Disc Replacements
The first published study in the field of artificial disc retrieval analysis was by Anderson et al.,30 who examined short-term, retrieved cervical disc replacements of two designs: the Bryan and the Prestige designs (Medtronic). The Bryan artificial disc is a one-piece design consisting of two titanium alloy shells articulating against a mobile polyurethane core. The end plates are connected by a polyurethane sheath. The Prestige design consists of two stainless steel end plates that articulate with a ball-in-trough mechanism. Both the Bryan and the Prestige designs are fully described by Kurtz.31 In the clinical study, the six Bryan retrievals were implanted on average 11.8 months (range, 4–16 months), and the two Prestige retrievals were implanted 18 months and 39 months.30 Few details about the retrieval methodology are included in this study, but it appears that microscopic characterization and, in the case of the Bryan disc, FTIR and gel-permeation chromatography (GPC) were performed. No significant changes in FTIR or GPC results were detected relative to unimplanted controls, but this is not surprising given the generally short-term nature of the explants and the small sample size. As the first retrieval study of its kind in disc arthroplasty, the work of Anderson et al.30 highlighted the importance of explant analysis for spine surgeons. However, because of the small numbers of retrievals and brief in vivo exposure, no conclusions can be drawn about generality of their findings.
Jensen et al.32 reported bone ingrowth into the titanium shells of Bryan retrievals from two patients who were revised after 8 months and 10 months of implantation. New bone growth was observed into the porous coating of all four retrieved end plates. The mean bone ingrowth, quantified by histologic sectioning, was 30.1% (12% standard deviation), which compared favorably with bone ingrowth reported in the literature for hip and knee replacements.
A retrieval study was presented at the 2007 Spine Arthroplasty Society in which the wear patterns in the core and sheath of the Bryan artificial disc were characterized.33 A secondary goal was to evaluate whether formalin storage could adversely affect the explants. Researchers tested the hypothesis that height loss of the core would increase with implantation time. Height loss was measured in the cores of 17 Bryan cervical TDRs that were retrieved from 14 patients (5 men, 7 women, 2 unknown) after 1.6 years in situ (range, 0.3–6.1 years). Implants were revised during 2003 to 2006 because of unresolved or recurring neck pain or radiculopathy (n = 15) and for infection or trauma (n = 1 each). Eight explants were stored in formalin for 1.4 to 3.3 years. Virgin, never-implanted sheaths and cores served as controls. SEM and WLIR were performed to identify wear mechanisms. The nominal height loss of the explanted cores (mean ± standard deviation) was 0.22 ± 0.09 mm (range, 0.04–0.35 mm). Although localized, microscopic evidence of adhesive and abrasive wear (confirmed by SEM and interferometry) was observed, researchers attributed most initial height loss to creep as opposed to material removal because the initial glossy surface finish of the cores was generally well preserved, even after 6.1 years in vivo. The sheaths typically showed evidence of folding or permanent deformation in regions where the core made repeated contact. No correlation was observed between core height loss and implantation time (ρ = 0.4, P = .18). No significant height difference was observed attributable to formalin storage. However, macroscopic changes in the explant surface, including cracking, occurred after 3 years of formalin storage; these findings were not present at the time of explanation. Researchers observed minimal wear and nominal changes in core height (approximately 0.2 mm) in this large series of polyurethane cervical disc explants. However, marked surface changes were noted after exposure of explants to formalin, and revising surgeons were cautioned to preserve polyurethane explants in a formalin-free environment.
The short-term in vivo wear performance of stainless steel Prestige cervical TDRs was characterized and compared with simulator results in two conference abstracts.34,35 In a preliminary study, the early wear tests by Anderson et al.30 were shown to produce similar wear mechanisms as retrievals; however, in the more recent study, the abrasion was more severe than what was observed in vivo.34 Because available Prestige retrievals were implanted for the short term, researchers conducted a second study to characterize the short-term wear response within the first 1.0 million cycles.35
At the 2008 meeting of the Orthopedic Research Society, researchers presented the results of three Prestige ST cervical TDRs that were wear tested in accordance with ISO/FDIS 18192.25 To evaluate the short-term in vitro wear behavior of the Prestige ST, the simulator was stopped after 0.05 million, 0.1 million, 0.2 million, 0.3 million, 0.4 million, 0.5 million, and 1.0 million cycles, and interval analyses were performed. These analyses consisted of photogrammetry and surface interferometry. The in vitro results from each interval analysis were compared with a Prestige ST retrieval collection analyzed from nine patients (two women and seven men). The artificial discs were Prestige I (n = 2), Prestige II (n = 2), or Prestige ST (n = 5), and all were of a stainless steel ball-and-trough design. The components had a range of implantation times from 0.7 to 3.3 years. Each component was previously characterized using the same methods as were used for in vitro interval analyses.
After 0.1 million cycles, the Prestige components exhibited a faint wear scar, produced by abrasive wear.35 This wear mechanism was consistent with short-term explants. The average surface roughness of the worn regions for both the retrievals and in vitro tested components was measured to be 0.12 ± 0.08 μm and 0.16 ± 0.07 μm. The results of this Prestige retrieval study suggest that the ISO/FDIS 18192 standard test method replicates the in vivo wear patterns in TDRs after less than 1 million cycles of testing. Previous wear test methods have shown other in vitro methods with up to 20 million cycles, which generated much more severe abrasive scratches than seen in vivo.30,34 The data also suggested that the same mechanism of abrasive wear is occurring at the bearing surface of both the retrievals and the components tested in vitro, although the greater worn surface area in the wear-tested components may indicate that the ranges of motion are more extensive than those experienced by TDRs in vivo.
Lumbar Spine Total Disc Replacements
With regard to fixation, bone ongrowth surfaces for TDRs have been tested in primate studies, but the fate of the bone-implant interface of lumbar TDRs in human patients has not yet been reported in the literature. Bone ongrowth could theoretically complicate revision. In an abstract presented at the 2006 Spine Arthroplasty Meeting,36 researchers investigated the failure modes, bone-implant interface, and extent of remaining calcium phosphate (CaP) coating in retrieved Charité TDRs with textured end plates. Eight textured end plates from four explanted TDRs were studied after 3 to 6 years in vivo. In each case, the coated end plates were revised in straightforward fashion by an osteotomy adjacent to the implant. None of the end plates had evidence of residual CaP, and one end plate had adherent bone visible (<10% of the surface area) on optical microscopy. The bone ongrowth to the textured surfaces was judged to provide improved resistance of the prosthesis to shear forces, which can result in migration when the teeth are not securely engaged in this design. Based on their findings, the authors advocated the use of textured, coated end plates over smooth end plates for total disc arthroplasty. Bone ongrowth has also been visually observed on the titanium–plasma spray coating of retrieved ProDisc end plates.29,37
Analysis of wear and surface damage in long-term implanted Charité TDRs has been reported in a series of journal publications.3,6,15 In the latest update of this multi-institutional series, 38 Charité components were retrieved with 16 years of implantation.15 The components were revised for intractable pain or facet degeneration. Components were analyzed using optical microscopy and micro-CT. One-sided wear patterns were shown in 43% (15 of 35) of components analyzed using micro-CT.15 Significant correlations were observed between implantation time and penetration and penetration rate. The dome of the components typically exhibited burnishing, which was consistent with the multidirectional wear observed in hip replacements, whereas the rim frequently showed evidence of radial and transverse cracking (19 of 38 and 14 of 38 retrievals), often produced by impingement. The rim damage modes of plastic deformation, delamination, and cracking were similar to those associated with knee components. The published Charité retrieval literature provides crucial long-term in vivo wear data for validation of spine wear simulators and for in vitro biomechanical testing.
Evidence of dome burnishing and rim impingement has also been noted in a more recent conference poster summarizing a collection of five short-term ProDisc-L prostheses implanted 2.2 years.37 The bearing surface of the ProDisc-L showed burnishing (three of five implants), mild scratching, and pitting (three of five implants). Impingement was noted in three of five components and associated with burnishing and plastic deformation. The authors of the ProDisc retrieval study remarked that, “a potentially worrisome finding is the evidence of impingement. Whether caused by patients achieving a larger range of motion that the implant is designed to accommodate or by component positioning that allows impingement at even smaller range of motion, impingement can be problematic.”37 A detailed TDR retrieval study for the ProDisc-L, displaying mild anterior impingement, has been reported more recently as a case study by Choma et al.29
Although rim impingement has been observed in retrieved TDRs of different designs, the clinical consequences of chronic rim impingement are poorly understood. In a study presented at the 2008 Spine Arthroplasty Society meeting,38 a retrieval collection of polyethylene mobile bearing TDRs was analyzed to determine whether rim impingement adversely affected dome penetration. Based on observations of plastic deformation, burnishing, or fracture of the rim, 28 of 40 (70%) of retrieved cores, implanted for 2 to 16 years (average, 7.9 years), were classified as exhibiting chronic rim impingement. Dome penetration was comparable in cores with chronic impingement (average, 0.3; range, 0.1–0.9 mm) compared with cores with no impingement (average, 0.3; range, 0.1–0.5 mm). Rim penetration was significantly greater in cores with chronic impingement (P < .05). Using linear regression, the dome penetration rate for cores with negligible impingement (0.036 mm/year, 95% confidence interval 0.012–0.061 mm/year) appeared slightly higher than for cores with chronic impingement (0.021 mm/year, 95% confidence interval 0.005–0.038 mm/year); however, the difference was not significant. The results of this study did not support the hypothesis that chronic rim impingement would be associated with greater dome penetration. However, the findings suggest that dome wear and impingement are effectively decoupled phenomena and may be studied independently of each other.
In addition to impingement, rim damage observed in polyethylene TDR retrievals has been associated with postirradiation oxidation.39 Analysis of explanted Charité cores using FTIR spectroscopy has shown that the exposed rim experiences severe oxidation after 10 or more years.39 These findings appear consistent for TDRs that were gamma irradiated in air and in first-generation polymeric barrier packaging.40 However, the central dome appears to be protected from in vivo oxidation owing to contact with the metallic end plates. No correlation was observed between wear of the central dome and oxidation.39 These observations are similar to the in vivo oxidation patterns noted in artificial hips, which exhibit rim embrittlement after 10 years in vivo but show reduced oxidation at the bearing surface where the femoral head contacts the polyethylene.40 In contrast to hip replacement, the rim of a TDR core may be intended to support chronic loading for the lifetime of the patient. The findings of in vivo oxidation in gamma-sterilized polyethylene TDR components provide additional motivation for developing in vitro mechanical tests that incorporate accelerated aging or some other oxidative challenge to simulate changes in the bearing materials that may occur with long-term in vivo exposure.
A study presented at the 2008 Spineweek meeting was conducted to correlate better long-term clinical wear rates of the Charité with simulator wear rates.41 It was hypothesized that the wear mechanisms of the retrievals would be more accurately simulated by International Standard of Organization (ISO) protocols with coupled motion compared with ASTM-type protocols that resulted in linear motion. Researchers analyzed dome wear rate and surface morphology of 41 Charité (SBIII) explants from 35 patients (71% female). The cores were implanted for 7.5 years (range, 1.8–16.3 years). Investigators also examined 12 Charité wear-tested cores and 6 controls. Six cores were tested according to an ASTM-type protocol for 10 million cycles42; three additional cores were unloaded and soaked. Six cores were tested according to the ISO protocol for 2 million cycles with three loaded and soaked controls. All of the cores in this study were produced by the same manufacturer (Link). The explanted cores typically exhibited burnishing or evidence of adhesive and abrasive dome wear, consistent with multidirectional motion. The wear rate of the explants, obtained by correlation of dome height with implantation time, was 0.023 mm/year. The ASTM-tested cores exhibited unidirectional abrasive wear at a rate of 0.007 mm/Mcycles (million cycles). The ISO-tested cores exhibited regional burnishing and wear at a rate of 0.124 mm/Mcycles. The ISO protocol generated wear surface morphology that was closer to the retrievals than the ASTM protocol, and 2 million cycles of the ISO protocol corresponded, on average, to about 5.6 years of clinical wear. The findings from this study further suggest that the ISO protocol provides a useful starting point for clinical validation of spine wear simulations incorporating lumbar polyethylene TDRs.
Because of its longer clinical history, more retrieval research has been published to date with metal-on-polyethylene lumbar discs than with metal-on-metal discs. At the present time, detailed results are available regarding the retrieval analysis of a single lumbar metal-on-metal TDR.43 This implant was removed after 12 months in situ at L5-S1 from a 43-year-old woman because of nerve root impingement. The components generally exhibited highly polished surfaces, similar to the surfaces observed on explanted metal-on-metal hip implants. The primary wear mechanism was microabrasion, which was evident by microscopic scratching of the articulating surfaces. Focal microplasticity was also observed at the apex of the dome and the anterior and posterior vertices of the cupped component, suggesting that the primary motion in these locations was flexion-extension. Surface deposits, manifested as a smoky or hazy discoloration, were observed on both components, consistent with organic films previously observed in well-functioning metal-on-metal hip joints. The surface features of the Maverick retrieval were compared with wear-tested components in a study by Pare et al.44 The surface topography of unidirectional tested components was found to be more severely abraded than the components that were tested under combined flexion-extension, lateral bending, and axial torsion. Although only a single retrieval was available for comparison at the time of the study, the retrieval results were more closely comparable to the wear test results with combined motion.
Dynamic Stabilization System Studies
Dynamic stabilization devices are nonfusion devices designed to stabilize the motion segment in lieu of fusion. Retrieval analysis for the Dynesys system (Zimmer Spine) has been reported. This system consists of fixed pedicle screws, PCU spacers that resist extension and compressive loads, and polyethylene terephthalate (PET) cords that resist flexion and tensile loading. Trommsdorff et al.25,45,46 examined the biostability of retrieved spacer and cord components.
In a retrieval study of 12 cords retrieved from 10 different patients (implanted 2–5.5 years), changes in surface chemistry were evaluated using attenuated total reflectance Fourier transform infrared spectroscopy (ATR-FTIR).45 Molecular weight distribution was evaluated using GPC. The retrieved cords were cleaned using an enzyme solution to remove biologic residue. The authors reported that the ATR-FTIR spectra obtained at different positions along the retrieved cords showed no signs of significant hydrolysis. Molecular weight analysis conducted on two retrieved cords (3.3 years and 5.5 years) did not show evidence of a significant change in molecular weight distribution. The authors concluded that the Dynesys PET cords showed good biostability at 5.5 years.
In a case study by Trommsdorff et al.,46 a system that had been implanted for 5.5 years was retrieved from L3-4 in a 56-year-old patient who had an abscess in proximity to the left spacer, which was presumably caused by infection. The contralateral component was not infected. Both spacers were retrieved, and their chemical structure was compared with a control component using ATR-FTIR. SEM was also used to evaluate regions on the spacer surfaces. Compared with the control spacer, the left spacer that was adjacent to the abscess showed changes in the infrared peaks that could be attributed to hydrolysis of the soft and hard segments of the PCU. On the right spacer, there was no remarkable chemical degradation. No change in the chemical structure was observed in either spacer at a depth of 100 μ or in the bulk material. SEM showed microcracks on the left spacer, whereas the right spacer was described as “perfectly smooth.” The authors concluded that the functionality of the implant was not impaired, because the degradation was limited to the surface layer (<2.5% of the wall thickness).
In a larger study of 50 retrieved Dynesys systems with implantation times ranging from a few months to 5.5 years, the investigators conducted optical microscopy, SEM, ATR-FTIR, and GPC analyses of the PCU spacers.25 The PET cords were also inspected using optical microscopy. The PET cords showed minor damage to the outermost layers in the regions of fixation but were intact elsewhere. The PCU spacers typically showed minor deformation owing to cold flow of the material. Regions of wear were observed, which the authors concluded to have been from articulation of the spacer with the facet joint. No changes in molecular weight distribution were observed in 3.3-year and 5.1-year retrievals. At the surfaces of the PCU spacers, small changes in chemistry were observed, which the authors attributed to the absorption of biologic fluids and minor hydrolytic changes of the material. At a depth of 100 μ below the surface, no change in the chemistry of the PCU was found. The authors concluded that the PET cords and PCU spacers were biologically stable over an implantation time of 5.5 years.
In a more recent abstract by Trommsdorff et al.47 summarizing the findings of 64 retrieved Dynesys systems, the results related to the PCU spacers were similar. The systems were implanted for up to 7 years. The authors also reported the incidence of screw loosening (20% of retrievals) and screw breakage (16% of retrievals). The screw breakages occurred at approximately one-third of the screw length from the tip of the screw and were apparently due to fatigue fracture. The authors reported that this rate was in the same range or lower than that of other comparable designs.
Ianuzzi et al.7,48 have reported similar results from their collection of retrieved Dynesys systems, which consisted of 75 spacers from 17 patients with implantation an average of 2.3 years (range, 0.7–7 years). The systems were primarily revised for persistent pain (16 of 17 patients) and screw loosening (11 of 17 patients); 1 patient experienced complications secondary to implant migration. Optical microscopy was conducted to evaluate spacer deformation and wear. ATR-FTIR was used to evaluate changes in surface chemistry compared with two control components. Similar to the results of Trommsdorff et al.,47 a focal region of abrasive wear was observed along the length of 51 of 75 spacers, which was likely due to impingement with surrounding bony structures. One spacer exhibited short surface cracks that extended from the center of the spacer to the outer surface. Changes in chemical structure were also observed on the surfaces of the spacers, although evidence of material degradation was observed in only 10 of 75 spacers. The findings from the spacers were determined to be incidental because the components from the short-term retrieval study were revised for reasons unrelated to wear, surface damage, or biostability.
Recommendations for Future Testing and Research
Because of the prevalence of impingement seen in the Charité retrievals, the authors recommend that impingement fatigue tests be developed to evaluate the performance of TDRs. In the Charité, impingement can occur during regular flexion or extension activities and has been shown with in vitro cadaver tests.49 Impingement can also occur secondary to subsidence, subluxation, or migration of the end plates. Because resistance to chronic impingement damage is desirable, fatigue test methods should be developed to reproduce the rim fracture modes observed in the Charité retrievals presented in this study. Once validated, the protocol could be used to screen implant materials for fatigue resistance under clinically relevant loading conditions. Additionally, given the potential for component oxidation, it would be useful to precondition test specimens using accelerated aging before rim fatigue tests.
Anderson P.A., Kurtz S.M., Toth J.M. Explant analysis of total disc replacement. Semin Spine Surg. 2006;18:1-12.
ASTM F561–05ASTM F561–05. Standard practice for retrieval and analysis of medical devices, and associated tissues and fluids. West Conshohocken, PA: American Society for Testing and Materials; 2005.
Ianuzzi A., Kurtz S., Kane W., et al. In vivo deformation, surface damage, and biostability of retrieved Dynesys systems. Spine (Phila Pa 1976). 2010;35:E1310-E1316.
Kurtz S.M., MacDonald D., Ianuzzi A., et al. The natural history of polyethylene oxidation in total disc replacement. Spine (Phila Pa 1976). 2009;34:2369-2377.
Kurtz S., Siskey R., Ciccarelli L., et al. Retrieval analysis of total disc replacements: implications for standardized wear testing. J ASTM Int. 2006;3:1-12.
Kurtz S.M., van Ooij A., Ross E.R.S., et al. Polyethylene wear and rim fracture in total disc arthroplasty. Spine J. 2007;7:12-21.
1. NIH Consensus Statement. Improving medical implant performance through retrieval information: challenges and opportunities. National Institutes of Health Technology Assessment Conference; 2000.
2. Anderson P.A., Kurtz S.M., Toth J.M. Explant analysis of total disc replacement. Semin Spine Surg. 2006;18:1-12.
3. Kurtz S.M., van Ooij A., Ross E.R.S., et al. Polyethylene wear and rim fracture in total disc arthroplasty. Spine J. 2007;7:12-21.
4. ASTM F561–05. Standard practice for retrieval and analysis of medical devices, and associated tissues and fluids. West Conshohocken, PA: American Society for Testing and Materials; 2005.
5. Kurtz S.M., Peloza J., Siskey R., et al. Analysis of a retrieved polyethylene total disc replacement component. Spine J. 2005;5:344-350.
6. Kurtz S., Siskey R., Ciccarelli L., et al. Retrieval analysis of total disc replacements: implications for standardized wear testing. J ASTM Int. 2006;3:1-12.
7. Ianuzzi A., Kurtz S., Kane W., et al. In vivo deformation, surface damage, and biostability of retrieved Dynesys systems. Spine (Phila Pa 1976). 2010;35:E1310-E1316.
8. Kurtz S.M., Muhlstein C.L., Edidin A.A. Surface morphology and wear mechanisms of four clinically relevant biomaterials after hip simulator testing. J Biomed Mater Res. 2000;52:447-459.
9. Kurtz S., Turner J., Herr M., et al. Assessment of surface roughness and waviness using white light interferometry for short-term implanted, highly crosslinked acetabular components. In: Kurtz S., Gsell R., Martell J., editors. Highly crosslinked and thermally treated ultra-high molecular weight polyethylene for joint replacements, ASTM STP 1445. West Conshohocken, PA: ASTM International, 2004.
10. Kurtz S.M., Turner J.L., Herr M., et al. Deconvolution of surface topology for quantification of initial wear in highly cross-linked acetabular components for THA. J Biomed Mater Res. 2002;63:492-500.
11. Bhushan B. Principles and applications of tribology. New York: John Wiley & Sons; 1999.
12. McKellop H.A., Campbell P., Park S.H., et al. The origin of submicron polyethylene wear debris in total hip arthroplasty. Clin Orthop Relat Res. 1995;311:3-20.
13. Wang A., Essner A., Polineni V.K., et al. Lubrication and wear of ultra-high molecular weight polyethylene in total joint replacements. Tribol Int. 1998;31:17-33.
14. David T. Revision of a Charite artificial disc 9.5 years in vivo to a new Charite artificial disc: case report and explant analysis. Eur Spine J. 2005;14:507-511.
15. Kurtz S.M., Patwardhan A., MacDonald D., et al. What is the correlation of in vivo wear and damage patterns with in vitro TDR motion response? Spine (Phila Pa 1976). 2008;33:481-489.
16. Villarraga M.L., Cripton P.A., Teti S.D., et al. Wear and corrosion in retrieved thoracolumbar posterior internal fixation. Spine (Phila Pa 1976). 2006;31:2454-2462.
17. Prikryl M., Srivastava S.C., Viviani G.R., et al. Role of corrosion in Harrington and Luque rods failure. Biomaterials. 1989;10:109-117.
18. Sim E. [Spinal internal fixator—analysis of problems in 28 cases]. Wien Klin Wochenschr. 1992;104:349-355.
19. Vieweg U., van Roost D., Wolf H.K., et al. Corrosion on an internal spinal fixator system. Spine (Phila Pa 1976). 1999;24:946-951.
20. Jacobs J.J., Gilbert J.L., Urban R.M. Corrosion of metal orthopaedic implants. J Bone Joint Surg [Am]. 1998;80:268-282.
21. Denaro V., Papapietro N., Sgambato A., et al. Periprosthetic electrochemical corrosion of titanium and titanium-based alloys as a cause of spinal fusion failure. Spine (Phila Pa 1976). 2008;33:8-13.
22. Anderson P.A., Rouleau J.P., Bryan V.E., et al. Wear analysis of the Bryan Cervical Disc prosthesis. Spine (Phila Pa 1976). 2003;28:S186-S194.
23. Hallab N.J., Cunningham B.W., Jacobs J.J. Spinal implant debris-induced osteolysis. Spine (Phila Pa 1976). 2003;28:S125-S138.
24. Kurtz S.M., Rimnac C.M., Hozack W.J., et al. In vivo degradation of polyethylene liners after gamma sterilization in air. J Bone Joint Surg [Am]. 2005;87:815-823.
25. Trommsdorff U., Zurbrugg D., Abt N.A., et al. Analysis of retrieved components of a dynamic stabilization system for the spine. presented at 68th Annual Meeting of the German Society for Surgery, Berlin, Germany. 2004.
26. Buscher R., Tager G., Dudzinski W., et al. Subsurface microstructure of metal-on-metal hip joints and its relationship to wear particle generation. J Biomed Mater Res. 2005;72:206-214.
27. Kurtz SM, McCombe P, Ciccarelli L, et al: In vivo wear mechanisms of a retrieved metal-on-metal total disc replacement, presented at 12th International Meeting on Advanced Spine Techniques (IMAST), Banff, Alberta, Canada, July 7-9, 2005.
28. van Ooij A., Kurtz S.M., Stessels F., et al. Polyethylene wear debris and long-term clinical failure of the Charite disc prosthesis: a study of 4 patients. Spine (Phila Pa 1976). 2007;32:223-229.
29. Choma T.J., Miranda J., Siskey R., et al. Retrieval analysis of a ProDisc-L total disc replacement. J Spinal Disord Tech. 2009;22:290-296.
30. Anderson P.A., Rouleau J.P., Toth J.M., et al. A comparison of simulator-tested and -retrieved cervical disc prostheses. Invited submission from the Joint Section Meeting on Disorders of the Spine and Peripheral Nerves, March 2004. J Neurosurg. 2004;1:202-210.
31. Kurtz S.M. Total disc arthroplasty. In: Kurtz S.M., Edidin A.A., editors. Spine technology handbook. New York: Academic Press; 2006:303-370.
32. Jensen W.K., Anderson P.A., Nel L., et al. Bone ingrowth in retrieved Bryan Cervical Disc prostheses. Spine (Phila Pa 1976). 2005;30:2497-2502.
33. Kurtz S, Heller J, Goffin J, et al: In vivo deformation and wear of retrieved Bryan cervical disc arthroplasties, presented at 7th Annual Meeting of the Spine Arthroplasty Society, Berlin, Germany, May 1–4, 2007.
34. Kurtz S.M., Chan F.W., Pare P.E., et al. Correlation of in vivo- and simulator-retrieved metal-on-metal cervical disc replacements. presented at 7th Annual Meeting of the Spine Arthroplasty Society, Berlin, Germany. May 1–4, 2007.
35. Siskey R., Kurtz S.M., Shah P., et al. Validation of ISO-Standard wear testing with retrieved metal-on-metal cervical disc replacements. presented at 7th Annual Meeting of the Spine Arthroplasty Society, Berlin, Germany. Transactions of the 54th Orthopedic Research Society. 2008;33;1927.
36. Kurtz S.M., van Ooij A., Ciccarelli L., et al. Analysis of textured endplates and bone ongrowth in retrieved Charité total disc replacements. Presented at Transactions of the 2006 Spine Arthroplasty Society Meeting, Montreal. May 9-13, 2006.
37. Wright T., Cottrell J. Retrieval analysis of ProDisc total disc replacements,. Berlin, Germany: 7th Annual Meeting of the Spine Arthroplasty Society; 2007. May 14
38. Kurtz S.M., Ianuzzi A., MacDonald D. Does chronic rim impingement influence dome wear in mobile bearing TDR?. Miami: Transactions of the 2008 Spine Arthroplasty Society Meeting, 2008;8:57. May 6–9, 2008
39. Kurtz S.M., MacDonald D., Ianuzzi A., et al. The natural history of polyethylene oxidation in total disc replacement. Spine (Phila Pa 1976). 2009;34:2369-2377.
40. Kurtz S.M., Hozack W.J., Purtill J.J., et al. Significance of in vivo degradation for polyethylene in total hip arthroplasty. Clin Orthop Relat Res. 2006;453:47-57.
41. Kurtz S.M., Ianuzzi A., Siskey R., et al. Clinical validation of spine wear simulators with long-term TDR wear rates for a mobile bearing polyethylene TDR. Geneva, Switzerland: Transactions of the Spineweek 2008 Meeting; May 26–31, 2008.
42. Serhan H.A., Dooris A.P., Parsons M.L., et al. In vitro wear assessment of the Charite Artificial Disc according to ASTM recommendations. Spine (Phila Pa 1976). 2006;31:1900-1910.
43. Kurtz S., McCombe P., Ciccarelli L., et al. Retrieval analysis of short-term implanted Maverick total disc arthroplasty. Proceedings. Philadelphia, PA: North American Spine Society; 2005.
44. Pare P.E., Chan F.W., Buchholz P., et al. Surface texture analysis of artificial disks wear-tested under different conditions and comparison to a retrieved implant. J ASTM Int. 2006;3:65-72.
45. Trommsdorff U., Zurbrugg D., Schneider W., et al. Biostability of poly(ethylene-terephthalate) cords used in a spinal implant system. presented at 7th World Biomaterials Congress, Sydney, Australia. 2004.
46. Trommsdorff U, Zurbrugg D, Stoll TM: In-vivo degradation of polycarbonate-urethane with and without contact to an abscess, presented at 7th World Biomaterials Congress, Sydney, Australia, 2004.
47. Trommsdorff U., Koettig P., et al. Analysis of retrievals of a dynamic stabilization system for the spine. presented at Eurospine, Barcelona, Spain. 2005.
48. Ianuzzi A., Kurtz S.M., van Ooij A., et al. In vivo deformation, surface damage, and biostability of retrieved Dynesys components for posterior dynamic stabilization. Transactions of the Orthopaedic Research Society, 2008;vol 33.
49. O’Leary P., Nicolakis M., Lorenz M.A., et al. Response of Charite total disc replacement under physiologic loads: prosthesis component motion patterns. presented at North American Spine Society, Philadelphia. 2005.