Chapter 12 Equipment for paediatric anaesthesia
Children neither look nor behave like small adults. Their requirements in the perioperative setting differ, including those of anaesthetic technique and equipment. In the UK, surgery for children constitutes less than 10% of the total surgery performed and, for financial reasons, development of equipment centres on the market for adult patients. Despite this, there is a rich history of innovation in paediatric anaesthesia. Some of the items we take for granted appear too simple to have been the subject of invention, an example of this being the T-piece breathing system developed from Magill’s system by Dr Phillip Ayre.1 It may not be the easiest to use,2 but lightly modified, remains popular with paediatric anaesthetists the world over (Fig. 12.1).
Anatomical and physiological differences between adults and children
Anatomical differences in the airway between adults and children
Compared to the adult, in the child:
• the tongue is relatively large and the larynx is in a higher position
• the epiglottis is longer and U-shaped
• in the younger child, the narrowest part of the upper airway is the cricoid ring: a tube passing easily through the laryngeal inlet may be too tight at the cricoid ring. Should insertion of a tracheal tube be necessary, its fit is critical. Too small a tube leads to an increase in resistance, large leak, and possible fluid ingress around the tube. Too tight a fit creates a risk of mucosal ischaemia and oedema, leading to stridor at extubation
• the larynx is smaller, so the reduction in diameter imposed by a tracheal tube will have a significantly larger effect on airway resistance to flow. The significance of apparatus dead space in comparison to the child’s total dead space becomes greater the smaller the child (Fig. 12.2). The two together may accommodate a high proportion of the tidal volume with a significant effect on carbon dioxide elimination.
Physiological differences in breathing between adults and children
• The chest wall of the child is more compliant, and contributes little to ventilation. The diaphragm moves less efficiently and contains fewer fatigue-resistant muscle fibres. Alveolar ventilation is, therefore, dependent more on rate; hence the respiratory rate in infants and younger children is higher than in adults. The high rate is achieved at the expense of the end expiratory pause. The latter is important for the efficient action of anaesthetic partial rebreathing systems (see below and Chapter 5).
• The work of breathing is higher in children, consuming relatively more oxygen. Airway maintenance devices and breathing systems, by presenting resistance to flow, contribute further to this. Though infants can cope with increased work of breathing in the short term, diaphragmatic fatigue will set in earlier than in adults.
• Closing capacity in small children may exceed and overlap functional residual capacity. This can be worsened by anaesthesia and the supine position, with resultant hypoxia. The ability to deliver positive end expiratory pressure or continuous positive airway pressure may help to overcome this.
• Compared to adults and older children, infants produce approximately twice as much carbon dioxide and consume twice the amount of oxygen relative to body weight.
Equipment
The anaesthetic machine
From its humble origins as a spartan trolley bearing just cylinders, vaporizers and the odd coffee stain, the anaesthetic machine has evolved into the anaesthesia workstation. The principles of the continuous flow machine remain the same, and this machine is most suited to paediatric practice. Draw-over apparatus presents too great a resistance to breathing to be used by children weighing under 20 kg and, if used, there must be some means of providing continuous flow, such as a manual bellows.3 For certain congenital cardiac conditions, use of air without obligatory oxygen flow is needed to avoid the pulmonary vasodilatation seen with higher oxygen concentrations. This requires a specially adapted anaesthetic machine. Aside from this unusual application, any continuous flow machine will suffice.
Transmission of infection
In an effort to prevent transmission of infection between patients, single-use equipment has become almost ubiquitous for paediatric anaesthesia in the developed world. The quality of components varies, but their build standard is generally ratified for use on one patient on one occasion only. This does not imply inferior quality. Lightweight durable plastics are particularly valuable in paediatric breathing systems, providing flexible low-resistance systems with a reduced tendency to ‘drag’ on other components. For adult patients, single-use breathing systems may be reused, provided an effective airway filter is used to isolate the system and anaesthetic machine from transmissible disease. Evidence is accumulating that paediatric filters are as efficient as the adult versions,4 but as yet the reuse of breathing systems is not encouraged. For more information see the section on breathing system humidification and filtration later in this chapter.
Regulation of equipment manufacture
The development and testing of new apparatus, and its ease of use, have been reviewed.5,6 Medical devices sold in the European economic area carry a CE mark (European mark of conformity assessment, Conformité Européene) placed by the manufacturer. To achieve this, the manufacturer provides details of risk analyses, performance in standard tests and technical data relating to manufacture of an item of equipment. The Competent Authority, for the UK, the Medicines and Healthcare Products Regulatory Agency, oversees this procedure (see Chapter 28). Medical devices are classified and tested according to potential risk of injury, e.g. a facemask is class 1 (low risk), a cardiac catheter class 3 (high risk).7 The CE marking process does not imply specific clinical testing; most pre-use testing is so-called bench testing, demonstrating equivalence or better function than existing similar equipment. Under these rules, scaling down of adult equipment to paediatric size is acceptable, but may not produce the most effective devices in use. An urge to release a new device meeting minimum standards onto the market is balanced against the need for commercial success; this provides manufacturers with an incentive to produce equipment with demonstrable clinical value. As an example, the laryngeal mask whilst scaled down from adult versions was still subject to specific testing to confirm it retained anatomical suitability for paediatric use.8 Further versions of this device have been subject to post marketing tests of performance in the clinical environment.9,10 A small number of devices without a CE mark are used on patients; these may be custom built or those requiring more clinical testing before a CE mark can be authorized. A device made within a hospital, for use in that hospital, does not require a CE mark, and may be provided with an exemption certificate for specified use elsewhere. In summary, excepting a small number of unique devices, all medical equipment used on children in the UK has a CE mark. This mark provides reassurance of manufacturing standards but does not imply clinical effectiveness, for which independent evaluation should be sought.
Equipment for management of the airway
Facemasks
A variety of paediatric facemasks exist (Fig. 12.3). To reduce dead space, the Rendell-Baker-Soucek mask was designed anatomically, from casts of children’s faces in the same way as a dental plate is made.11 This mask achieves a seal by virtue of its close approximation to the contours of the face. Other masks require some form of flexible lip or air filled cushion. The lipped round silicone mask (Fig. 12.3E) is easy to apply, providing an excellent seal for infant use. Disposable masks generally employ a cushion seal, the rest of the mask being of rigid construction. Whichever is chosen, it must be easy to hold and seal on the face, and this may well be a matter of trial and error. Attempts to reduce facemask anatomical dead space may be less important than previously thought, the actual increase in physiological dead space with anaesthesia being less than predicted.12
Tracheal tubes
Tracheal tubes are available in sizes and shapes to suit different patients and surgical procedures (Fig. 12.4). The internal diameter of the tracheal tube is the major determinant in airway resistance and hence the size by which tubes are measured and selected. The fit of the tube to each patient is determined by external diameter which varies with tube wall thickness. This is itself determined by the type of tube (Table 12.1), but can also vary for the same tube from different manufacturers.13 Other factors affect tube resistance: connectors, tube length, shape of tube and tendency to collect secretions.
Table 12.1 Dimensions of some non-cuffed infant endotracheal tubes
MANUFACTURER | INT. DIAMETER (mm) | EXT. DIAMETER (mm) |
---|---|---|
Portex (silicone) | 2.5 | 3.4 |
Sheridan (Ped-soft) | 2.5 | 3.6 |
Portex (ivory) | 2.5 | 3.6 |
Mallinkrodt (PVC) | 2.5 | 3.6 |
Rusch (clearway) | 2.5 | 4.0 |
Mallinkrodt (reinforced) | 2.5 | 4.0 |
Portex (reinforced) | 2.5 | 4.0 |
Rusch (rubber) | 2.5 | 4.0 |
Portex (silicone) | 2.5 | 4.2 |
Sheridan (Ped-soft) | 3.0 | 4.2 |
Portex (ivory) | 3.0 | 4.4 |
Mallinkrodt (PVC) | 3.0 | 4.3 |
Rusch (clearway) | 3.0 | 4.7 |
Mallinkrodt (reinforced) | 3.0 | 4.7 |
Portex (reinforced) | 3.0 | 4.7 |
Rusch (rubber) | 3.0 | 4.7 |
Portex (silicone) | 3.5 | 4.8 |
Sheridan (Ped-soft) | 3.5 | 4.9 |
Portex (ivory) | 3.5 | 5.0 |
Mallinkrodt (PVC) | 3.5 | 4.9 |
Rusch (clearway) | 3.5 | 5.3 |
Mallinkrodt (reinforced) | 3.5 | 5.3 |
Portex (reinforced) | 3.5 | 5.3 |
Rusch (rubber) | 3.5 | 5.3 |
The decision to intubate, and which tracheal tube to use, is of great significance. Previous attempts to circumvent the problem of tube resistance included the use of shouldered and tapered tubes, but these designs have been shown to confer no real advantage (Fig. 12.5). Below the age of 10 years, uncuffed tracheal tubes were the norm and were believed to minimize the chance of mucosal damage and post extubation stridor. Despite this perceived advantage, the lack of an airway seal with uncuffed tubes can permit fluid to enter the tracheobronchial tree, contribute to atmospheric pollution, lead to inadequate ventilation and induce anaesthesia in surgeons working around the upper airway.
Widespread use of uncuffed tubes has been questioned.14 Cuffed tubes offer advantages (Table 12.2) and are safe when used appropriately.15 New and better designs of cuffed paediatric sized tubes are emerging but more work still remains to be done, particularly on cuff position in preformed (shaped, e.g. RAE type) tubes and on the relationships between tube length and diameter.15 A change in practice will take time, and selecting an uncuffed tube with a leak at an inflation pressure of 25 cm H2O will remain common practice, despite evidence that in short procedures at least, it confers no benefit.16
Table 12.2 A comparison of cuffed and uncuffed tubes
Uncuffed tracheal tube | Cuffed tracheal tube | |
---|---|---|
Seal | attempts a seal in the cricoid ring, but seal may not be effective | used appropriately forms a good seal below cricoid in larger diameter of trachea |
Effect on airway mucosa | may be less prone to causing damage | less evidence of long term safety currently available |
Available lumen | maximizes available lumen of artificial airway | may reduce the available lumen |
Other | – allows use of smaller diameter tubes which may impact post-intubation laryngeal morbidity – choice of tube diameter less critical – lower tube exchange rates – new designs still needed with tube dimensions revised for new paradigm of cuffed tubes. |
Tube size selection is critical particularly for uncuffed tubes; formulae provide only a guide to the correct tube size (Table 12.3). Coexisting medical conditions may influence tube size, for example: children with Down syndrome often require a tube 1–2 mm smaller than expected for their age.17 Likewise, the required length of tube can only be estimated. Some tubes incorporate marks intended to guide how far to advance the tube into the larynx under direct vision. Preformed tubes may have a mark indicating the position for fixation over the lip. The placing of such marks is inconsistent across tube sizes and manufacturers, and they should not be relied upon.
Fixation of the tube should aim to prevent displacement, maintain the tube position with head movement, and still be relatively easy to secure and adjust (Fig. 12.6). Simple tape fixation fulfills many of these criteria (Fig. 12.7). Nasal intubation in children is more secure and preferred in the intensive care setting, as the tube tends to move less, reducing trauma to the tracheal mucosa.
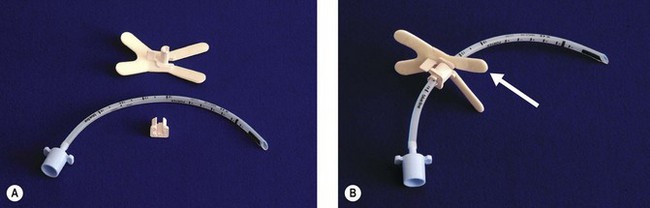
Figure 12.6 A. A device for fixing tracheal tubes, The Portex RSP B. The same device locked onto the tube, self-adhesive strips (arrowed) are used to fix the device to the face.
Flow at the interface of breathing system and tube is disturbed by changes in diameter and direction. Connectors aim to minimize this by smooth internal surfaces, gradual reductions in diameter and gentle direction changes. The commonest tube connector is the ISO 15 mm; another ISO standard system based on 8.5 mm connectors (Fig. 12.8) appears rarely used. Connectors do not reduce the available lumen as they dilate the tube at the point of insertion. Problems can arise when assembling small thin-walled parts, with buckling of the walls and possible occlusion of the lumen.18 Some older connectors remain in use as they are compact and may offer less resistance to gas flow (Fig. 12.9). Endotracheal tubes allow suction to be applied to the lower airway. To size a suction catheter for use, doubling the tube diameter in mm, gives the appropriate French gauge catheter size.19
Laryngeal Masks
Since its introduction, the Laryngeal Mask Airway (LMA) has been credited with a revolution in anaesthetic technique, and is widely used for spontaneous and controlled ventilation.20
Chapter 6, page 151, ‘Other laryngeal masks’, details the distinction in terminology used to describe these devices. The LMA has also proved valuable in managing the difficult airway, such as that encountered with Pierre Robin sequence.21 It can be used to guide fibreoptic intubation, or even blind passage of a tracheal tube,22 although caution is advised with the latter technique.23 A range of sizes, from neonatal to older child, is available (Fig. 12.10); size selection is based on patient weight (Table 12.4). Where more flexibility of the tube is needed, a reinforced version is available down to size 2 (Fig. 12.11).
Table 12.4 Recommended sizes and dimensions of laryngeal masks showing also the largest tracheal tube (TT) diameter that may be passed through each size
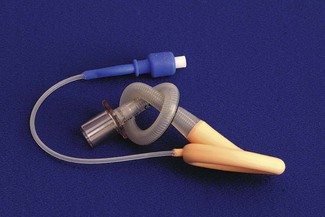
Figure 12.11 A size 2 (the smallest) reinforced laryngeal mask airway. The knot is for illustrative purposes only.
The laryngeal mask has lower resistance to gas flow compared with a tracheal tube, causes minimal stimulation of the airway and offers some protection against pulmonary aspiration of fluid from above. The classic design does not protect against aspiration of regurgitated fluid. The ProSeal LMA is designed to overcome this limitation, and early experience of this device with children is encouraging.24 It is recommended the laryngeal mask be inserted in exactly the same way as for the adult patient.8 Positioning and securing the laryngeal mask is generally easily accomplished in children, though some difficulties may be encountered with the smaller sizes, 1 and 1.5.25 In any event, infants usually need intubation and controlled ventilation for anaesthesia of longer duration.
When used for controlled ventilation, a small leak often occurs around the laryngeal mask. Attempts to silence this leak by sealing the patient’s mouth around the LMA stem or placing oropharyngeal packs may result in gastric insuflation and are to be discouraged. Loss of gas is minimal with normal inflation pressure; successful use with controlled ventilation and a circle absorber has been described.26 Pressure controlled ventilation is advantageous, as it allows effective ventilation with lower airway pressures than volume controlled ventilation.27 Oesophageal pH studies during controlled ventilation reveal no greater incidence of gastro-oesophageal reflux with the laryngeal mask compared to tracheal tube or facemask.28
Examination of the airway under anaesthesia and some surgery around the airway can be accomplished with a laryngeal mask in place.29 This may allow better maintenance of anaesthesia and oxygenation during the procedure, compared with jet entrainment or apnoeic techniques. Care is needed with laryngeal mask cuff pressures, particularly if nitrous oxide is employed; unchecked pressures are usually higher than expected and may injure structures in and around the upper airway.30 Overall, the complication rate is low and the laryngeal mask and its variants have a place in anaesthesia for an increasingly wide range of paediatric patients.
Other supraglottic airway devices
A number of other supraglottic airways have followed in the wake of the laryngeal mask. Their acceptance has been gradual and unbiased evaluation scarce. The outcome of appeals for new initiatives in further independent assessment of clinical performance is awaited.31
Airway adjuncts
Scaled versions of oral and nasal airway adjuncts exist for paediatric use (Figs 12.12 and 12.13) and are discussed in Chapter 6.
Gaining access to the airway
The laryngoscope
The larynx is usually seen with the direct laryngoscope. A variety of laryngoscope blade profiles exist. The choice is usually dependent upon the age of the patient and the personal preference of the anaesthetist (Fig. 12.15). Many practitioners use a straight blade for infant laryngoscopy; this blade picks up the relatively large epiglottis, affording a better view. For older children, a curved (small Macintosh pattern) blade will suffice. The little finger of the hand holding the laryngoscope may be used to apply external laryngeal pressure to improve the view. Flexible tracheal tube introducers can be used to railroad a tube into a larynx when a direct view cannot be obtained. These are available for use with tubes as small as 2.5 mm internal diameter.
A development of the bougie is the airway exchange catheter (AEC) (Fig. 12.16). A semi-rigid, hollow catheter with interchangeable 15 mm ISO male and Luer-Lok connectors, this is designed to allow tracheal tubes to be exchanged whilst retaining the ability to oxygenate the lungs. The AEC is inserted through the tracheal tube, which can then be removed and exchanged over this. Once in place, the end connector can be fastened, gas sampled from the tip to detect carbon dioxide and correct position confirmed. Oxygen can then be injected or insufflated through the catheter with either a high-pressure injector or a standard anaesthetic breathing system. With the connector removed, a tracheal tube can be guided over the catheter and into the trachea.32 The catheters are available to fit within tracheal tubes down to 3 mm internal diameter.
The fibreoptic bronchoscope
Both the standard size and a smaller 2.2 mm, flexible fibreoptic bronchoscope can be used to aid intubation in children when the larynx is difficult to visualize. The standard bronchoscope incorporates a suction channel, which can be used to thread a guide wire that is advanced into the trachea under direct vision. After removal of the bronchoscope, the wire remains in the trachea and is used to railroad a tracheal tube of the appropriate size.33
Use of the 2.2 mm bronchoscope follows the adult pattern of fibreoptic intubation, whereby the tracheal tube (down to size 2.5 mm) is ‘loaded’ onto the bronchoscope prior to visualizing the laryngeal inlet. This technique is suited to difficult intubation in infants. A tracheal tube without its connector is passed along the bronchoscope (Fig. 12.17). Following induction of anaesthesia, the airway is maintained with a laryngeal mask. The right-angle connector in the breathing system incorporates a sealing port, through which the bronchoscope is advanced, passing through the bars of the laryngeal mask and into the trachea. The cuff of the laryngeal mask is deflated and withdrawn back along the bronchoscope. The tube is advanced, along the bronchoscope, through the laryngeal mask and into the trachea (Fig. 12.18). The bronchoscope is withdrawn, the tube connector replaced, and ventilation resumed when correct tube position is confirmed.34 The tube may need changing over a suitable introducer (see above) for one of better fit. If the tube is long enough and the laryngeal mask does not impede the surgical view, the whole assembly can be left in position throughout surgery.
Instead of the laryngeal mask, a split oropharyngeal airway or modified tracheostomy tube can be used as a guide for the bronchoscope.35 With all these techniques, components must be pre-assembled and compatibility confirmed, with particular attention to ensuring the components will fit through one another. When using fibreoptic intubation in children, oral intubation is usually preferable, as the nasal airway may be abnormal, and trauma to the nasal mucosa with subsequent bleeding can abolish the view completely.
Rigid fibreoptic and other optical system laryngoscopes continue to be introduced for children following their evolution in adult anaesthetic practice (see Chapter 6). As with the newer generations of supraglottic airway, concerns regarding unproven clinical efficacy surround these devices. Experience varies and the important point is reiterated that even with a normal airway, the view achieved with a fibreoptic device is easily degraded by the different conditions of the paediatric upper airway.36,37
Alternative approach to the difficult airway
Cricothyroid cannulation offers an alternative approach when difficulty maintaining and securing the airway is anticipated or experienced. Use of proprietary cricothyroid cannulation kits (Fig. 12.19) has been described in children.38 Unlike adult practice, these techniques are difficult to conduct on awake individuals. Certainly in small children, with a small trachea and large neck veins, this option presents a challenge and the safety of elective transtracheal ventilation has been questioned. For similar reasons, rapid formation of a tracheostomy is not a straightforward option to rely on in an emergency.39
Anaesthetic breathing systems
The ability to continually and accurately measure inspired oxygen and end-tidal CO2 has allowed attention to be refocused on systems operating with low fresh gas flows and reduced pollution. Early breathing system designs required clinical testing with invasive monitoring to generate safe operational limits for use in conjunction with the limited monitoring prevailing in clinical practice. Latterly, patient monitoring has improved greatly. Pulse oximetry and end-tidal carbon dioxide monitoring, together with clinical observation, allow safer and more confident use of a variety of systems.12
Apparatus dead space is difficult to determine with accuracy, though clearly should be minimized. Some items have recommendations for use based on their internal volume, e.g. breathing system filters. The contribution to dead space by a particular piece of equipment may differ from its measured volume, due to the effects of mixing by gas flows within the item. The term functional dead space incorporates this phenomenon.
Partial rebreathing of exhaled gas is a feature of some breathing systems, and is described in more detail in Chapter 5. The T-piece, classified as Mapleson E system, is an example of this. It is worth noting that it is similar in function to the D system.
Repeatedly reversing the direction of flow of relatively large volumes of gas provides additional inertial resistance in the system, which is reduced with by use of smaller-bore hoses. The latter may have a reinforcing coil placed around the outside (in place of the standard corrugated type) permitting a smooth internal surface, with lower resistance to gas flow. Although all tubing is designed to show marked resistance to deformation, this form may kink more easily on extreme flexion (Fig. 12.20).
Compression volume (Table 12.5) during positive pressure ventilation refers to a combination of breathing system distension and internal compression of gas within that system caused by a pressure rise during inspiration. This will affect the inspiratory volume delivered to the patient (see below).
Definition | Volume lost during positive pressure ventilation due to compression of gas within the system |
Features in effects | All breathing systems |
More significant with large volume systems | |
More significant with small tidal volumes | |
Can be compensated for |
A large compression volume results in high system compliance, reducing the ‘feel’ when hand ventilating. This may be why the ‘educated hand’ of the anaesthetist is a poor detector of changes in patient compliance and system occlusion.12
Ideal airway and breathing equipment for paediatric anaesthesia
Breathing systems for use with very small children should ideally:
• have minimal functional/apparatus dead space
• be either valveless or fitted with very low resistance valves
• have small internal gas volumes
• be constructed in such a way as to minimize gas turbulence and subsequent flow resistance
• provide for heating and humidification together with filtration.
Breathing systems in use
Breathing systems, their performance and classification are considered in detail in Chapter 5. For paediatric use, armed with knowledge of the features discussed earlier, it is possible to consider the reasons for and against use of any one breathing system in a particular context, such as varying age and size of child, altered lung compliance and need for spontaneous or controlled ventilation. There is no absolute as to when children become suitable for conventional adult systems. Some authors suggest that at 20–25 kg body weight, i.e. with a tidal volume of around 140 ml, some adult systems function satisfactorily.40 If ventilation is controlled, adult systems can be used for smaller children than this.41
T-piece (Mapleson E and F)
The term ‘T-piece’ has evolved over time to now commonly describe the breathing system with the Jackson Rees modification (see below) rather than the original as described by Philip Ayre. The ‘T-piece’ is a valveless system with very low resistance, low apparatus dead space, and is usually used with an open-ended bag (Jackson Rees modification) on the expiratory limb (Fig. 12.1). A number of variants of the T shaped connector itself have been mostly superseded by the single-use disposable system in the form shown. ‘T-piece’ has a low compression volume and requires relatively high fresh gas flows (see Chapter 5) allowing rapid changes in composition of inspired gas. Classified as a Mapleson E without the bag and an F with it, this system functions in a similar way to the Mapleson D, though it lacks an expiratory valve. The T-piece is intrinsically very simple, lightweight and easy to use with few connections. It can be used for children of all ages for spontaneous ventilation, controlled ventilation and application of continuous positive airways pressure. If the bag is of the open-ended type, partial occlusion of the opening with the little finger against the palm of the hand allows the anaesthetist to carefully monitor and control ventilation with the body of the inflated bag manipulated between thumb and opposing finger(s).
Formulae exist for recommended fresh gas flows, but are for guidance only. For spontaneous ventilation, a fresh gas flow of up to three times predicted minute ventilation is required; for controlled ventilation this may be less, depending on the technique. If the fresh gas flow is used to drive inspiration by intermittent occlusion of the expiratory limb, higher flows are needed to provide adequate inspiratory flow. Manual ventilation with a reservoir bag or automatic ventilation with a ventilator, such as the Penlon Nuffield Series 200, enables use of a lower fresh gas flow. Ultimately, continuous monitoring of end tidal carbon dioxide is required for accurate use. The structure of the system makes it difficult to scavenge waste gas, though modifications exist to allow this.42 To scavenge from the unmodified T-piece requires some form of collector but vigilance is required to avoid occlusion of the outlet and subsequent high pressures in the system.
The T-piece is inefficient, requiring high fresh gas flows increasing with patient size. Scavenging is possible, but detracts from the system’s simplicity. These disadvantages, together with improvements in other systems and patient monitoring, have led to reduced use of the T-piece in some centres. Recognized as a simple system to use with the ability to provide continuous positive airways pressure and giving a ‘feel’ of airway dynamics, the T-piece remains popular for induction, short procedures and as a stand-by when problems arise (Table 12.6). Most disposable T-piece systems are provided with a connector allowing them to draw fresh gas from wall or cylinder oxygen, again useful for emergencies or patient recovery (Fig. 12.21).
Table 12.6 Advantages of the T-piece
Humphrey ADE
To maximize the advantages of different systems for different modes of ventilation, single systems have been designed which can perform essentially as a Mapleson A for spontaneous ventilation and a Mapleson D for controlled ventilation. This system was first described in 1983 (Fig. 12.22 and see Fig. 5.13). By employing parallel tubing and a low-resistance exhaust valve sited distant from the patient, control and monitoring of ventilation, with scavenging, are easily accomplished. A lever at the machine end is used to switch from the spontaneous A mode to controlled ventilation in the D mode, achieved with a ventilator such as the Penlon Nuffield Series 200. For children weighing under 20 kg, breathing spontaneously in the A mode, a fresh gas flow of 3 L min−1 prevents rebreathing. In the controlled ventilation mode the system performs like a T-piece; a fresh gas flow of 3 L min−1 maintains normocapnia. If the fresh gas flow is adjusted for weight, a lower end tidal carbon dioxide can be achieved.43 More recently, a carbon dioxide absorber has been incorporated, allowing even lower fresh gas flows.
Circle system
When the sweet-smelling, explosive anaesthetic agent cyclopropane was in common use, perhaps a circle system, with its ability to conserve gas by reducing gas flow and its high internal humidity, saved both money and lives. Such was the attraction of circle systems that for paediatric usage – in an era dominated by concerns about apparatus dead space and ‘work of breathing’ and prior to the availability of routine capnography – scaled-down versions44 or systems in which gas movement was assisted by some form of circulator45 were proposed. These were described in detail in the third edition of this textbook. Circle breathing systems are no longer considered to need such modifications for paediatric use. However, it is noteworthy that the concept of circulating gas is being reintroduced in a new guise (see Chapter 4, Dräger Zeus).
In current use, the perceived advantages of circle systems are a reduction in fresh gas flow, easy scavenging, reduced atmospheric pollution and addition and conservation of some heat and water vapour to inspired gas. When changes from adult to paediatric set-up are required, using the same system reduces the chances of assembly errors. However, if spontaneous ventilation is to be used in the smaller child it is perhaps worthwhile changing the breathing hoses from 22 mm to 15 mm diameter, and using smaller distal connectors with minimal dead space.
The circle system has been used successfully in children and infants, the latter usually with controlled ventilation.46 Reduction in fresh gas flow is less significant in small children, where all breathing systems employ a relatively low flow anyway.
Additionally, leaks from the system around the tracheal tube or laryngeal mask will limit flow reduction, though in practice this rarely precludes efficient use of the circle system.26 Gauging and rapidly changing the concentration of oxygen and volatile agent in the system is less easy than with the T-piece, and monitoring of these variables is mandatory. The large compression volume is reduced to some extent by the use of 15 mm tubing, but remains greater for this system.
The circle is a more complicated system than others used in paediatrics. However, used with appropriate monitoring and vigilance, it provides the added benefit of, amongst others (Table 12.7), significant reduction in wastage of anaesthetic agents.47
Breathing system humidification and filtration
Humidification of dry inspired gas by the patient is an important source of heat loss. Dry inspiratory gasses increase tracheal mucosal damage and the incidence of tracheal tube blockage. A range of simple heat and moisture exchangers is now available and in common use by anaesthetists (see Chapter 11). The level of humidification and heat retention necessary to prevent the above problems is unknown. Furthermore, the performance of humidifiers varies significantly between manufacturers and during use.48
Anaesthesia circuits may become contaminated with microbes from the patient’s respiratory tract; hepatitis C virus transmission has been reported between patients49 linked to breathing system contamination. Combined airway humidifiers and filters aim to prevent microbes entering the breathing system, whilst conserving airway heat and humidity (Fig. 12.23). Their widespread adoption into clinical practice may be beneficial, but should receive some appraisal. Filters contribute dead space and resistance to the breathing system, and may lead to more serious complications.50 These effects are greater for smaller tidal volumes, young children in particular require careful observation.51,52 In addition to such problems when using filters in children, there are doubts about the efficiency of these products. On standardized assessment, filters from various manufacturers exhibit differing particle penetration.53 This may result from a failure to account for the lower flow rates pertaining in paediatric systems,4 but in practice means paediatric airway filters have not been shown to offer system isolation to the same extent as their adult counterparts. Additionally it would seem that even very modest hydrostatic pressures of 0.3–0.5 kPa can cause liquid penetration of filters, which may carry with it infective agents.54 A recent study was able to demonstrate bacterial contamination of the machine (breathing system) side of filters in 9% of sampled filters.55 At the time of writing, knowledge of airway filtration is insufficient to recommend that airway filters allow reuse of breathing systems in children.
Ventilators for use during paediatric anaesthesia
Ventilators are described and classified in more detail in Chapter 9.
Ventilators designed specifically for paediatric use should ideally have a low internal volume and resistance, the ability to deliver small tidal volumes and high respiratory rates and a pressure-control feature. However, increasing sophistication of microprocessor control has allowed ventilators with larger internal volumes, e.g. the Penlon AV900 (Fig. 12.24), to be suitable for both paediatric and adult patients and most modern anaesthesia workstation ventilators are currently suitable for use with small children.
This following classification is descriptive of the mode of action:
Bag squeezers
There are commonly two types of ‘bag squeezer’. In one, the reservoir bag of the partial rebreathing or, more commonly, circle system is replaced by a ‘bag in bottle’ (bellows) arrangement that is also part of the ventilator system (see Fig. 9.7). The bellows may either be a standard ‘adult’ bellows (1500 ml) or it may be substituted for a smaller size (500 ml) so as to reduce the internal gas volume and, therefore, the compliance within the system. For a similar reason, the standard wide bore (22 mm) breathing hose may be replaced by a smaller bore (15 mm). Reducing the compliance was hitherto given greater priority as it reduced the ‘compression volume’ within the breathing system allowing more predictable tidal volumes to be delivered (see above). However, modern ventilators incorporate microprocessor control and feedback monitoring, allowing the compliance to be calculated and adjustments made to restore the tidal volume to that desired.
In paediatric anaesthesia there is additionally often a leak around the tracheal tube that prevents the accurate determination of tidal volume. Pressure-controlled ventilation overcomes many of these problems (Table 12.8). The ventilator delivers gas into the system to rapidly reach the preset pressure and this is maintained until expiration occurs. This compensates for leaks around the tracheal tube, the effects of compression volume and changes in fresh gas flow. It also reduces the risk of barotrauma. However, simple pressure ventilation alone provides no compensation for reduced ventilation following changes in lung compliance or breathing system and airway resistance. Adequate ventilation is ensured by monitoring the end tidal CO2 and adjusting the inspiratory pressure appropriately, hence monitoring of expired gasses at least for composition if not volume is important.
Table 12.8 Advantages of pressure control
In the second type of ‘bag squeezer’, the reservoir bag is replaced by a suitable length of breathing hose, one end of which is attached to the breathing system (see Fig. 9.7). The other end is connected to a valve that is fed by driving gas delivered from the ventilator. The gas is diverted into the breathing hose and acts as a gas piston mimicking the action of a manually squeezed reservoir bag. As the hosing also acts as the exit pathway for patient gas, its length must be sufficiently long to prevent driving gas entering the breathing system and diluting the anaesthetic gasses (see below). The Penlon Nuffield Series 200 ventilator (see Chapter 9) popularized this method and there are now a number of similar ventilators that perform the same function.
Hybrid systems: the Newton valve
This ingenious device can function as a sophisticated mechanical thumb or a bag squeezer and was designed for the Penlon Nuffield 200 ventilator (Fig. 12.25). The valve is attached to the expiratory limb of a T-piece system. The latter should have a minimum volume of 350 ml to prevent the driving gas from diluting the inspired gas.
Table 12.9 Advantages of volume control
• At the recommended fresh gas flow rate for the T-piece and at a low inspiratory flow rate from the ventilator, the pressure developed inside the Newton valve is low as a result of the continuous leakage from the fixed orifice outlet. Therefore, the valve only partially dams the outlet of the breathing system and so acts as a ‘partial thumb occluder’. This transmits a small tidal volume to the patient at a rate depending on, but less than the fresh gas flow into the T-piece.
• As the flow of driving gas from the ventilator increases, at some stage, inflow to the valve will be equal to the leak through the orifice and at this time the valve behaves almost as a complete thumb occluder on the T-piece expiratory limb. The delivered tidal volume then equals the fresh gas flow to the T-piece.
• Further increasing flow from the ventilator results in flow into the valve exceeding the leak, and some of the driving gas now passes back along the T-piece expiratory limb and can act as a gas piston. Tidal volumes will now exceed fresh gas flow and are altered by ventilator settings.
• During the expiratory phase, all gas passes out via the valve orifice, and for expiratory flows up to 15 l min−1 pressure within the valve should not rise above 5 cm H2O.
Modified with a Newton valve, the Nuffield 200 Series ventilator may be seen as the mechanical equivalent of the anaesthetist’s hand in combination with the open-ended bag on a T-piece. The system is easy to understand, can be switched rapidly from manual to automatic ventilation and permits scavenging of waste gas. It can deliver tidal volumes between 10 and 300 ml at frequencies from 10 to 85l min−1, making this a suitable ventilator for neonates and infants. The system requires high fresh gas flows and consumes large amounts of pressurized gas to drive the ventilator. The Newton valve is not suitable for patients over 20 kg.
Access to the circulatory system
Obtaining and maintaining secure venous access in small patients can be very challenging. As with adults, there are essentially two means to achieve this: the cannula over needle or wire through needle (Seldinger) techniques. Use of rigid indwelling needles alone is not recommended, as they tend to cut out of the vein resulting in extravasation of administered fluids. Much smaller cannulae are required for both peripheral and central access. Flow rates through narrow-bore cannulae are low (Table 12.10). In an emergency it may be impossible to cannulate a vein. Below the age of 6 years an intraosseous needle (Fig. 12.26) provides alternative access to the circulatory system for administration of drugs and fluid until venous cannulation is achieved. All contaminated sharps must be disposed of safely, and some cannulae incorporate a retraction device to ensure the needle end is covered after the vein is entered (Fig. 12.27). Cannulation of central veins, particularly the internal jugular and femoral veins, has traditionally been undertaken using surface landmarks as a guide. The use of two-dimensional ultrasound to cannulate central veins is well described.56 Ultrasound has been suggested to improve the success and safety compared to landmark techniques.57 Where long-term venous access is required, peripherally inserted lines may be easier to site and can be threaded into central veins if necessary.
Positioning, environmental control and temperature monitoring
Great care is required with positioning during anaesthesia, including eye protection and protection of vulnerable peripheral nerves, etc. Padding under the shoulders of the supine infant prevents the large occiput from putting the head into flexion. Depending on the site of surgery, a bar or bridge placed over the patient prevents the surgeon from inadvertently leaning on the chest or face and allows improved access for the anaesthetist to monitor the patient, check line sites, etc. When a limb tourniquet is used, they must be of adequate width and exceed limb circumference by 7–15 cm. Padding is needed, particularly at the edges, and the tourniquet can be inflated to a lower pressure than that for adults. Skin preparing fluids must not soak under the tourniquet, as the child’s thinner skin is easily damaged.58
All patients can lose heat during anaesthesia, the thermoneutral temperature zone (about 28°C in an unclothed adult) being higher in neonates. Small children have limited thermogenesis, so heat loss may be difficult to recoup.59 Depending on the operation and exposure, heat is lost through a combination of radiation, convection, conduction and evaporation of bodily fluids and can be prevented by a number of measures:
• Maintain the operating theatre at a higher temperature and humidity; this is rarely within the limits of staff comfort.
• Reduce evaporative loss by limiting exposure of wet areas at the operative site and humidifying inspired gas.
• Reduce radiation loss with foil blankets; correctly applied these reduce convective loss too (Fig. 12.28).
• Active warming, most commonly in the form of forced air warming, is a more effective way of maintaining temperature.60 A warm air microclimate can be created around the child by use of forced warmed air, in combination with impervious clear plastic covers adapted for surgical access (Fig. 12.29).
Transfer of the critically ill child
An increasing trend for transferring critically ill children to centralized paediatric intensive care units requires safe and rapid transfer conditions, usually provided by a retrieval team from the accepting unit. Resuscitation is initiated at the referring unit, often under the guidance of the retrieval service. A challenge for practitioners with little exposure to sick children is to judge appropriate tube sizes, etc. This is made easier by some form of universal measuring device together with prepared packs of equipment,61 such as the Broselow Tape and the Broselow Paediatric ALS Organizer.
Transfer brings risks from moving a potentially unstable patient into an environment compromised by motion, cold, poor light, noise and limited electric power. Ideal equipment for this is lightweight, robust and compact, and has a reliable power supply. All equipment can fail and manual back-up, such as self-inflating bags, is vital. Transfer equipment is kept together in a series of clearly identified portable packs (Fig. 12.30); all batteries are continuously charged and packs are replenished and checked for immediate reuse after a transfer. Equipment requirements vary according to the size of child and the nature of the illness, but it is likely that ventilation and sedation will be needed together with inotrope infusions and intravenous fluids.
Portable ventilators (Fig. 12.31) need to be capable of delivering air/oxygen mixes, creating positive end expiratory pressure, displaying airway pressures, detecting and alarming for disconnections and must have adjustable tidal volumes for a range of patient sizes. They are usually driven from a high-pressure oxygen source and have high gas consumption (20 L min−1 for the example shown). Tubes and lines need to be secured sufficiently well to survive multiple transfers and movement. Temperature control can be difficult; rigorous use of foil helps, and highly insulating material such as ‘bubble wrap’ provides excellent thermal insulation. Single-use heated mattresses are available for infants, heated by initiating a chemical reaction of the contents. Compact single module monitoring is available and even blood biochemistry and gasses can be monitored with a portable machine (Fig. 12.32).
Monitoring
Adult monitoring equipment is usually simple to adapt for children. Old-style monitoring, such as the oesophageal stethoscope, is still valuable and almost free of technical complications. More ambitious surgery with large volume blood loss requires invasive monitoring of cardiovascular parameters. Arterial and central venous cannulation are useful, and means now exist for less invasive monitoring of cardiac output, e.g. by arterial waveform analysis following calibration by a dilution technique (see Chapter 16). In neonates, blood gasses are monitored with transcutaneous sensors. This is less effective for older children, but fluorescent optical sensors sited on a catheter within a blood vessel can provide continuous readings of blood gasses without the need for repeated sampling. Mandatory modern monitoring has not been shown to improve the outcome from anaesthesia, but it does provide early warning of problems to the alert anaesthetist. Full monitoring of the awake child may be tricky, but once asleep it should be a formality.
Finally
Fear of the unknown and patchy knowledge derived from a multitude of sources fuels apprehension that is palpable to the child. A number of helpful publications exist that may allay some fears.62 An in-depth knowledge of paediatric anaesthesia and the requisite equipment is fulfilling and vital. It will go unnoticed by your patients, whose admiration is saved for those au fait with the more complex fields of the latest children’s toys, animals (real, stuffed and extinct) and apparently banal television programmes!
1 Ayre P. Endotracheal anaesthesia for babies; with special reference to hare-lip and cleft palate operations. Current Research in Anesthesiology. 1937;16:330.
2 Meakin GH. Role of the Jackson Rees T-piece in pediatric anesthesia [editorial]. Pediatric Anesthesia. 2007;17:613–615.
3 Hodges SC, Hodges AM. A protocol for safe anaesthesia for cleft lip and palate surgery in developing countries. Anaesthesia. 2000;55:436–441.
4 Malan CA, Wilkes AR, Hall JE, Gildersleve C. An evaluation of the filtration performance of paediatric breathing system filters at low flows. Anaesthesia. 2007;62:504–508.
5 Cook TM. Novel airway devices: spoilt for choice? [editorial]. Anaesthesia. 2003;58:107–110.
6 Bridgland IA. Monitoring medical devices: the need for new evaluation methodology [editorial]. Br J Anaesth. 2001;87:678–680.
7 Grant LJ. Regulations and safety in medical equipment design [editorial]. Anaesthesia. 1998;53:1–3.
8 Brain AI. An evaluation of the laryngeal mask airway during routine paediatric anaesthesia [letter]. Paediatr Anaesth. 1995;5:75.
9 Kelly F, Sale S, Bayley G, Cook T, Stoddart P, White M. A cohort evaluation of the pediatric ProSeal laryngeal mask airway in 100 children. Pediatric Anesthesia. 2008;18:947–951.
10 Sanders JC, Olomu PN, Furman JR. Detection, frequency and prediction of problems in the use of the proseal laryngeal mask airway in children. Pediatric Anesthesia. 2008;18:1183–1189.
11 Rendell-Baker L, Soucek DH. New pediatric facemasks and anesthetic equipment. Br Med J. 1962;I:1690.
12 Fisher DM. Anesthesia equipment for pediatrics. In: Gregory GA, ed. Pediatric anesthesia. New York: Churchill Livingstone; 1989:437–475.
13 Malhotra N. Tracheal intubation in paediatrics: select tubes from the same manufacturer [letter]. Anaesthesia. 2006;61:725–726.
14 James I. Cuffed tubes in children. Paediatrics in Anaesthesia. 2001;11:259–263.
15 Weiss M, Gerber AC. Cuffed tracheal tubes in children-things have changed [editorial]. Pediatric Anesthesia. 2006;16:1005–1007.
16 Khalil SN, Mankarious R, Campos C, Chuang AZ, Lemak NA. Absence or presence of a leak around tracheal tube may not affect postoperative croup in children. Paediatr Anaesth. 1998;8:393–396.
17 Allt JE, Howell CJ. Down’s syndrome. British Journal of Anaesthesia. CEPD Reviews. 2003;3:83–86.
18 Gupta K, Harry R. Cutting paediatric tubes – a potential cause of morbidity [letter]. Br J Anaesth. 1997;78:627.
19 Mackway-Jones K, Molyneux E, Phillips B, Wieteska S. Advanced paediatric life support, 3rd ed. London: BMJ Books; 2001.
20 Gursoy F, Algren JT, Skonsby BS. Positive pressure ventilation with the laryngeal mask airway in children. Anesth Analg. 1996;82:33–38.
21 Beveridge ME. Laryngeal mask anaesthesia for repair of cleft palate. Anaesthesia. 1989;44:656–657.
22 Stocks RM, Egerman R, Thompson J, Peery M. Airway management of the severely retrognathic child: use of the laryngeal mask airway. Ear Nose Throat J. 2002;81:223–226.
23 Auden SM, Lerner GM. Blind intubation via the laryngeal mask: a word of caution [letter]. Paediatr Anaesth. 2000;10:452.
24 Sanders JC, Olomu PN, Furman JR. Detection, frequency and prediction of problems in the use of the proseal laryngeal mask airway in children. Pediatric Anesthesia. 2008;18:1183–1189.
25 Bagshaw O. The size 1.5 laryngeal mask airway (LMA) in paediatric anaesthetic practice. Paediatr Anaesth. 2002;12:420–423.
26 Frohlich D, Schwall B, Funk W, Hobbhahn J. Laryngeal mask airway and uncuffed tracheal tubes are equally effective for low flow or closed system anaesthesia in children. Br J Anaesth. 1997;79:289–292.
27 Keidan I, Berkenstadt H, Segal E, Perel A. Pressure versus volume-controlled ventilation with the laryngeal mask airway in paediatric patients. Paediatr Anaesth. 2001;11:691–694.
28 Ozlu O, Turker AK, Ozgun G, Soykan I. Distal oesophageal pH measurement in children during general anaesthesia using the laryngeal mask airway, tracheal tube and face mask. Paediatric Aneasthesia. 2001;11:425–430.
29 Baraka A, Choueiry P, Medawar A. The laryngeal mask airway for fibreoptic bronchoscopy in children. Paediatr Anaesth. 2005;5:197–198.
30 Ong M, Chambers MA, Hullett B, Erb TO, von Ungern-Sternberg BS. Laryngeal mask airway and tracheal tube cuff pressures in children: are clinical endpoints valuable for guiding inflation? Anaesthesia. 2008;63:738–744.
31 Wilkes AR, Hodzovic I, Latto IP. Introducing new anaesthetic equipment into clinical practice [editorial]. Anaesthesia. 2008;63:571–575.
32 Thomas PB, Parry MG. The difficult paediatric airway: a new method of intubation using the laryngeal mask airway, Cook airway exchange catheter and tracheal intubation fibrescope. Paediatr Anaesth. 2001;11:618–621.
33 Hasan MA, Black AE. A new technique for fibreoptic intubation in children. Anaesthesia. 1994;49:1031–1033.
34 Ellis DS, Potluri PK, O’Flaherty JE, Baum VC. Difficult airway management in the neonate: a simple method of intubating through a laryngeal mask airway. Paediatr Anaesth. 1999;9:460–462.
35 Wrigley SR, Black AE, Sidhu VS. A fibreoptic laryngoscope for paediatric anaesthesia. Anaesthesia. 1995;50:709–712.
36 Bein B, Wortmann F, Meybohm P, Steinfath M, Scholz J, Dörges V. Evaluation of the pediatric Bonfils fibrescope for elective endotracheal intubation. Pediatric Anesthesia. 2008;18:1040–1044.
37 Ravishankar M, Kundra P, Agrawal K, Kutralam NS, Arun N, Vijaykumar OP. Rigid nasendoscope with video camera system for intubation in infants with Pierre Robin sequence. Br J Anaesth. 2001;87:728–731.
38 Depierraz B, Ravussin P, Brossard E, Monnier P. Percutaneous transtracheal jet ventilation for paediatric endoscopic laser treatment of laryngeal and subglottic lesions. Can J Anaesth. 1994;41:1200–1207.
39 Seefelder C, Kenna MA. Don’t rely on the surgical airway: a case of impossible tracheostomy [letter]. Paediatr Anaesth. 2000;10:224.
40 Hatch DJ. Paediatric anaesthetic equipment. Br J Anaesth. 1985;57:672–684.
41 Brown TCK, Fisk GC. Anesthesia for children, 2nd ed. Oxford: Blackwell Scientific Publications; 1992. p. 53–76
42 Dhara SS, Pua HL. A non-occluding bag and closed scavenging system for the Jackson Rees modified T-piece breathing system. Anaesthesia. 2000;5:450–454.
43 Orlikowski CE, Ewart MC, Bingham RM. The Humphrey ADE system: evaluation in paediatric use. Br J Anaesth. 1991;66:253–257.
44 Rasch DK, Bunegin L, Ledbetter J, Kaminskas D. Comparison of circle absorber and Jackson Rees systems for paediatric anaesthesia. Can J Anesth. 1988;35:25–30.
45 Revell DG. A circulator to eliminate mechanical dead space in circle absorption systems. Can J Anesth. 1959;6:98–103.
46 Rasch DK, Bunegin L, Ledbetter J, Kaminskas D. Comparison of circle absorber and Jackson Rees systems for paediatric anaesthesia. Can J Anaesth. 1988;35:25–30.
47 Meakin GH. Low-flow anaesthesia in infants and children. Br J Anaesth. 1999;83:50–57.
48 Brock-Utne JG. Humidification in paediatric anaesthesia [editorial]. Paediatr Anaesth. 2000;10:117–119.
49 Heinsen A. A phylogenetic analysis elucidating a case of patient-to-patient transmission of hepatitis C virus during surgery. J Hosp Infect. 2000;46:309–313.
50 Lawes EG. Hidden hazards and dangers associated with the use of HME/filters in breathing circuits. Their effect on toxic metabolite production, pulse oximetry and airway resistance. Br J Anaesth. 2003;91:249–264.
51 Chau A, Kobe J, Kalyanaraman R, Reichert C, Ansermino M. Beware the airway filter: deadspace effect in children under 2 years. Pediatric Anesthesia. 2006;16:932–938.
52 Bell GT, Martin KM, Beaton S. Work of breathing in anesthetized infants increases when a breathing system filter is used. Pediatric Anesthesia. 2006;16:939–943.
53 The Medicine and Healthcare Products Regulatory Agency. Breathing system filters: an assessment of 104 breathing system filters. www.mhra.gov, March 2004.
54 Cann C, Hampson MA, Wilkes AR, Hall JE. The pressure required to force liquid through breathing system filters. Anaesthesia. 2006;61:492–497.
55 Rees LM, Sheraton TE, Modestini C, Wilkes AR, Hall JE. Assessing the efficacy of HME filters at preventing contamination of breathing systems. Anaesthesia. 2007;62:67–71.
56 Pirotte T, Veyckemans F. Ultrasound-guided subclavian vein cannulation in infants and children: a novel approach. Br J Anaesth. 2007;98:509–514.
57 National Institute for Clinical Excellence. Guidance on the use of ultrasound locating devices for placing central venous catheters. London: NICE; 2002.
58 Kam PCA, Kavanaugh R, Yoong FFY. The arterial tourniquet: pathophysiological consequences and anaesthetic implications (review article). Anaesthesia. 2002;56:534–545.
59 Crossley AWA, Holdcroft A. Physiology of heat balance. In: Jones RM, editor. Royal College of Anaesthetists newsletter. 1993;47:155–158.
60 Giesbrecht GG, Ducharme MB, McGuire JP. Comparison of forced-air patient warming systems for perioperative use. Anesthesiology. 1994;80:671–678.
61 Cole R. When every second counts. Child Health. 1995;3:63–67.
62 Rawlinson SC, Short JA. The representation of anaesthesia in children’s literature. Anaesthesia. 2007;62:1033–1038.
Bingham R, Lloyd-Thomas A, Sury M, eds. Hatch and Sumner’s textbook of paediatric anaesthesia, 3rd ed, London: Hodder Arnold, 2007.
Hatch D, Fletcher M. Anaesthesia and the ventilatory system in infants and young children. Br J Anaesth. 1992;68:398–410.
Steven JM, Cohen DE, Sclabassi RJ. Anesthesia equipment and monitoring. In: Motoyama EK, Davis PJ, eds. Smith’s Anesthesia for infants and children. 6th ed. New York: Mosby; 1996:229–279.